Abdalla M. Darwish1, Simeon Wilson1, Ashley Balckwell1, Keylanta Taylor1, Sergey S. Sarkisov2, Darayas N. Patel3, Brent Koplitz4
1PhysicsDepartment, Dillard University, New Orleans, USA
2SSS Optical Technologies, LLC, Huntsville, USA
3Department of Mathematics & Computer Science, Oakwood University, Huntsville, USA
4Chemistry Department, Tulane University, New Orleans, USA
Correspondence to: Abdalla M. Darwish, PhysicsDepartment, Dillard University, New Orleans, USA.
Email: |  |
Copyright © 2015 Scientific & Academic Publishing. All Rights Reserved.
Abstract
The objective of the paper was to demonstrate feasibility of an ammonia sensor using polymer –inorganic nano-composite thin film upconversion light emitters made by the new double-beam pulsed laser deposition method. The existing pulsed laser deposition vacuum chamber was modified to accommodate two laser beams of different wavelengths for the in-situ ablation of two targets: a polymer host poly(methyl methacrylate) mixed with indicator dye Phenol Red and the brilliant rare earth doped upconversion phosphor NaYF4:Yb3+, Er3+. Nano-composite films were deposited on silicon substrates by the proposed method with near-infra-red laser radiation (1064-nm wavelength) ablating the polymer target dissolved in Gamma-butyrolactone together with the indicator dye, and frozen in circulating liquid nitrogen (matrix assisted pulsed laser evaporation – MAPLE), and visible radiation (532 nm) ablating the inorganic target. The deposited nano-composite films retained bright green upconversion fluorescence with a spectral peak at 540 nm attributed to the inorganic phosphor nano-particles pumped with the 980-nm infrared laser diode. The spectrum of the green emission matched the absorption band of the indicator dye exposed to ammonia. When the films were exposed to ammonia, they demonstrated an optical response in the form of the drop of the intensity of green radiation monitored with a silicon photodiode. The sensitivity of the opto-electronic sensor of ammonia based on the nano-composite films was measured to be close 0.4% ammonia in air, and the response time was 5 minutes.
Keywords:
Polymer-inorganic nano-composite films, Pulsed laser deposition, Nano-particle dopants, Ammonia sensors
Cite this paper: Abdalla M. Darwish, Simeon Wilson, Ashley Balckwell, Keylanta Taylor, Sergey S. Sarkisov, Darayas N. Patel, Brent Koplitz, Ammonia Sensor Based on Polymer-Inorganic Nano-composite Thin Film Upconversion Light Emitter Prepared by Double-Beam Pulsed Laser Deposition, American Journal of Materials Science, Vol. 5 No. 3A, 2015, pp. 8-15. doi: 10.5923/s.materials.201502.02.
1. Introduction
Ammonia is a common hazardous air pollutant. A study by the National Research Council [1] identified ammonia emissions as a major air quality concern at regional, national, and global levels. Besides its well-documented direct negative effect on humans and animals at relatively high concentrations (above 25 ppm), it also has many significant negative impacts on ecology, which build up over time at much lower concentrations (within ppb range). Deposition of atmospheric ammonia can cause eutrophication of surface waters, where phosphorus concentrations are sufficient to support harmful algal growth. Nutrient enrichment and eutrophication lead to the decline of aquatic species, including those with commercial value. Sensitive crops such as tomatoes, cucumbers, conifers, and fruit cultures can be damaged by over-fertilization caused by ammonia deposition if they are cultivated near major ammonia sources [2]. The deposition of ammonia on soils with a low buffering capacity can result in soil acidification or basic cation depletion. Volatilized ammonia can travel hundreds of miles from the site of origin. In Europe, scientists have concluded that nitrogen pollution in the Mediterranean Sea is caused in large part by ammonia emissions in northern Europe. Ammonia emissions from the Midwestern United States may contribute to eutrophication of the Gulf of Mexico. The Chesapeake Bay is likely receiving ammonia deposition from upwind areas with intensive agricultural operations such as Ohio and North Carolina. In addition to its effects on water, plant, and soil systems, ammonia reacts with other compounds to form particulate matter (PM) with a diameter of 2.5 microns or less, which is referred to as PM2.5. This classification of PM is of particular concern because the small size of the particles allows them to penetrate deep into the lungs. Several recent community health studies indicate that significant respiratory and cardiovascular problems are associated with exposure to PM2.5. Other problems associated with long-term exposure to fine particles include premature death and increased hospital admissions from respiratory causes. These fine particles also contribute to the formation of haze. In the United States, haze has reduced natural visibility from 90 miles to between 15 and 25 miles in the East and from 140 miles to between 35 and 90 miles in the West [3]. EPA estimates that animal agriculture accounts for 50% to 85% of total man-made ammonia volatilization in the United States [4]. Currently, ammonia emissions in ambient air are regulated only indirectly through the EPA issued National Ambient Air Quality Standards (NAAQS) for PM2.5 closely associated with atmospheric ammonia [5]. However, some states established their own direct standards for ammonia emissions. For instance, Arizona has an acceptable level of ammonia concentration in ambient air of 2000 ppb (24-h long exposure) [6]. Some regulatory reports mandate maximum ammonia concentrations of 500 ppb at property fence lines of agriculture facilities. Attempts to put ammonia emissions under control are facing the lack of inexpensive, reliable, and maintenance free sensor of ammonia. EPA with the help of Battelle conducted verification testing of the instruments from different providers in terms of continuous monitoring of ambient ammonia [7]. All the tested instruments were expensive ($20 to $150K) and required substantial maintenance. In a separate development, the Department of Homeland Security (DHS) has issued comprehensive Guide 100-04 with the description of several dozens of sensors of ammonia [8]. The group of sensors (0-200 ppm range), which might be used for environment monitoring, is dominated by electrochemical devices. Their major disadvantage is a short operational lifetime (5000 ppm/h or less). Optical colorimetric sensors are good candidates to address the problem: they offer heightened sensitivity to ammonia (within sub-ppm and ppm range) combined with low cost and ruggedness [9]. They have three major components: light source, reagent film changing its optical absorbance in response to ammonia, and the photodetector. The reagent film is made of some host material (polymer, sol gel, etc.) impregnated with a pH indicator dye changing its color (optical absorbance in a particular spectral region) in response to ammonia (which reduces pH). Further advancement in the technology has been recently proposed by Marder et al. [10]. They added to a polymer matrix doped with indicator dye Phenol Red nano-particles of the rare earth (RE) compound that upconverts the frequency of the infra-red (IR) pumping source and re-emits in several bands of visible light. The advantage here is that one band of the emitted visible light matches the ammonia-sensitive absorption band of the indicator dye while the others fall out. So the “matching” emission band could be used to read the response to ammonia while the others were used as the references to compensate parasitic responses to temperature, humidity, and other fluctuations thus improving sensitivity. This paper describes the result of the investigation of a colorimetric sensor of ammonia based on the polymer / indicator-dye / RE-compound nano-composite reagent film prepared by the pulsed laser deposition method (PLD). PLD technique in its new variant of the double beam deposition combined with the matrix assisted pulsed laser evaporation (DB-MAPLE) of the polymer/indicator-day material [11, 12] improves the homogeneity of the nano-composite organic-inorganic film and better controls its thickness and the doping proportion the RE nano-particulate in the polymer matrix. This paper describes the preparation method, physical properties of the reagent film, the performance of the sensor of ammonia, and makes conclusions on its usefulness.
2. Reagent Film of the Sensor
The principle of functioning of the ammonia sensor under consideration is illustrated in Figure 1. A polymer nano-composite film is additionally doped with an indicator dye (Phenol Red), which turns from yellow to red upon exposure to atmospheric ammonia. The upconversion phosphor nano-particulate of the film is illuminated with an infrared laser diode (980 nm wavelength) and generates upconversion visible light of green color (~ 540 nm spectral peak). When the indicator dye exposed to ammonia turns red, it absorbs the green light from the phosphor: higher concentration of ammonia corresponds to the weaker intensity of the green light recorded by a photodetector. As can be seen from Figure 2, green emission peak of the upconversion phosphor falls within the absorption band of Phenol Red exposed to ammonia. Reagent nano-composite film was made using the DB-MAPLE method. A dye-doped polymer host (deposited with the MAPLE method using the first laser beam) was impregnated with the nano-particles of a highly efficient upconversion RE compound (co-deposited using the regular PLD with the second laser beam). 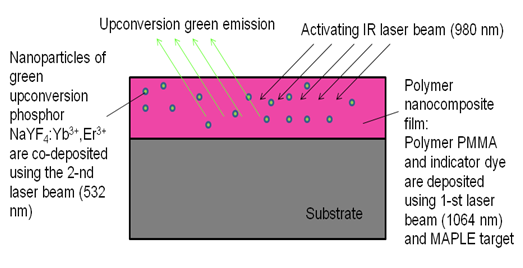 | Figure 1. Diagram explaining the principle of operation of the polymer-inorganic nano-composite upconversion film as a chemical sensor |
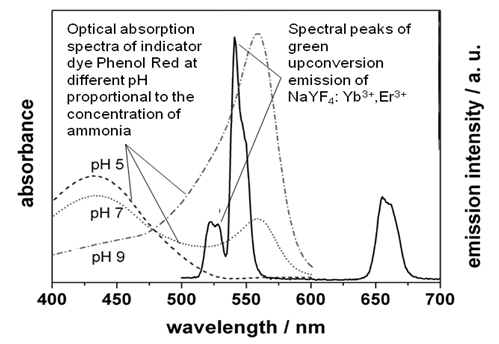 | Figure 2. Preliminary experimental results: absorption spectrum of indicator dye Phenol Red (dissolved in water) matches green upconversion spectral peaks of the phosphor |
2.1. Upconversion Phosphor
Suitable hosts for the RE ions with efficient energy upconversion are based on materials with low phonon energies, which minimize the non-radiative multi-phonon relaxation process of the RE dopant. Very efficient upconversion phosphors are based on fluorides, such as crystalline hexagonal-phase of NaYF4 (β-NaYF4), doped with RE ions [13, 14]. In this work NaYF4 crystals co-doped with trivalent RE ions of Yb3+ and Er3+ were synthesized in powder form using the solution based technique (wet process) in the presence of Na2-ethylenediaminetetraacetic acid (EDTA) during the co-precipitation procedure to obtain homogeneous nucleation. First, 0.5 mol of NaF was dissolved in about 60 ml of water. An aqueous rare-earth chloride solution was prepared by mixing 16 ml of 0.2-mol YCl3, 3.4 mol YbCl3, and 0.6 ml of 0.2-mol ErCl3. The YCl3 solution was obtained by dissolving Y2O3 in hydrochloric acid and adjusting to pH 2 to avoid any hydrolysis. An RE (Yb or Er) chloride solution was allowed to mix with 20 ml of 0.2-mol EDTA solution for metal-EDTA complex to occur. All the RE chlorides, EDTA and NaF were obtained from Aldrich, and the Y2O3 was synthesized in the lab using Y(NO3)3 and Na2CO3 from Aldrich. The EDTA complex solution was quickly introduced into the NaF solution and the mixture was allowed to stir vigorously for several hours. After stirring, the solution was allowed to sit overnight for the precipitate to settle. The precipitate was filtered, washed several times with distilled water and with ethanol. The precipitate was dried under vacuum to remove any traces of water. The freshly prepared NaYF4:Yb3+, Er3+ crystalline powder prepared in the above procedure did not show any upconversion fluorescence. After annealing at a temperature of 400°C for one-hour, strong upconversion fluorescence from the powder illuminated with a 980-nm IR laser diode could be observed by naked eye at room illumination. As can be seen from the optical absorption spectrum of the compound in Figure 3, a laser diode with the emission peak near 980-nm is the optimal pumping source for the upconversion emission. The energy of the laser photons is mostly absorbed by the ions of Yb3+ acting as synthesizers, then transferred radiationlessly to the ions of Er3+, which generated the upconversion, higher energy photons due to the two-photon process [12].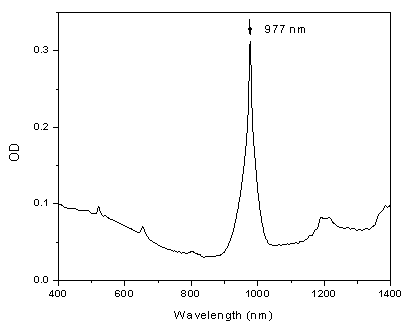 | Figure 3. Optical absorption spectrum of the NaYF4: Er3+, Yb3+ phosphor |
Optical fluorescent spectroscopy of the powder was conducted using a 980-nm laser diode PL980P330J from Thorlabs (330-mW maximum power, quantum-well laser chip, pigtailed with a wavelength stabilizing fiber Bragg grating) as a pumping source. The samples were at room temperature. Optical upconversion fluorescent spectrum was taken with the Princeton Instruments 500-mm-focal-length Spectra Pro (SP – 2500i) imaging spectrometer/monochromator equipped with 1200 gr/mm (blazed at 500 nm) holographic diffraction grating and PI-Max 1024 HQ Digital Intensified CCD Camera system. The crystalline powder was compressed into a flat pellet. The emission measurement was done in reflectance mode using a sample chamber with the sample pellet placed approximately at an angle of 45° with respect to the optical axis of the entrance slit of the monochromator. The upconversion emission spectrum of the compound (Figure 2) had two prominent peaks in visible region: green and red. The green peak falls in the absorption band of indicator dye Phenol Red when it is exposed to ammonia.
2.2. Polymer Host
Indicator dye Phenol Red Sodium Salt (PR) was dissolved in Gamma-butyrolactone (GBL) at a concentration of 88 mM/L using heating to a temperature of 60°C and stirring with a magnetic stirrer. Polymer poly (methyl methacrylate) known as PMMA in powder form was added to the solution at a concentration of 1 g of solids per 10 mL of liquids and dissolved using heating (at 70°C) and magnetic stirring. In order to check the response to ammonia, a 20-µm-thick film was made on a glass slide by dipping and drying for one hour. The film had initially yellow color. After exposure to the fume (ammonia) of ammonium hydroxide, the film changed color to dark purple and returned back to yellow after the exposure. The optical absorption spectrum of the film exposed and unexposed to ammonia matched the optical absorption spectrum of PR presented in Figure 1. The “green” band of upconversion emission of NaYF4:Yb3+, Ho3+ around 540 nm fell in the ammonia initiated absorption band of the dye. It could thus be expected that in the nano-composite film containing the dye and the RE material, the green upconversion emission would be noticeably absorbed in the presence of ammonia.
2.3. Pulsed Laser Deposition
The conventional pulsed laser deposition (PLD) of a single material onto a substrate includes the sequence of the processes: (a) heating a single target with the laser pulse; (b) melting the heated target material followed by its vaporization; (c) ionizing the atoms of the vaporized target material by the electrons accelerated in the strong electric field of the laser pulse and creating weakly ionized plasma; (d) expanding of the plume made of the weakly ionized plasma driven by electrostatic repulsion of the positive ions of the target material towards the ambient gas or vacuum separating the target from the substrate; (e) condensing of the target material from the plume on the substrate and thin film formation. Since the spread of the plume is driven by electrostatic repulsion, the axis of the plume is always normal to the surface of the target regardless of the direction of the incident laser beam. A new double-beam PLD method was used to make the nano-composite organic-inorganic reagent film [11, 12].The PLD system at Dillard University is schematically presented in Figure 4. Only laser beams 1 and 2 were used in this work. The laser beams were forced to scan over the targets with oscillating (in X and Y directions) reflectors (Figure 5) to eliminate pre-mature target erosion and cracking due to laser ablation of a target in a single spot.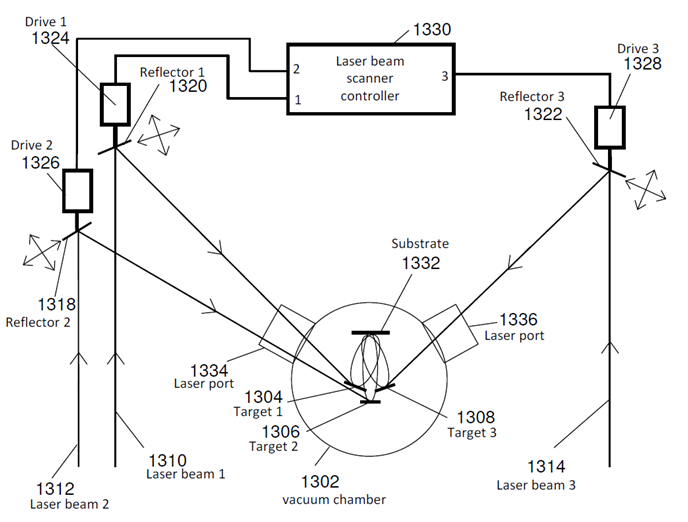 | Figure 4. Schematic of the multiple (three) beam PLD system with laser beam scanners |
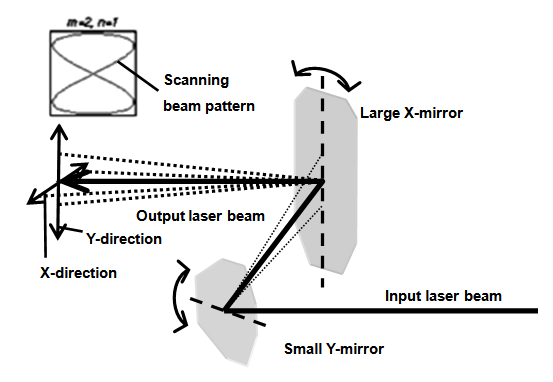 | Figure 5. Schematic of the laser beam scanner based on oscillating reflectors |
Two laser beams of different wavelength ablated/evaporated concurrently two targets with two different materials: dye-doped polymer and inorganic upconversion compound. There had to be an optimal tilt angle maintained between the targets in order to achieve constant overlapping of the plumes from both targets on the substrate. The overlapping is needed to have the uniform composition of the constituents over the film. Figure 6 presents the schematic of the optical feedback system, which makes possible manual, semi-automatic, or automatic control of the plume overlapping. The target holder is split in two halves: the static holder 104 of the 1-st target (110) and holder 106 of the 2-nd target with variable tilt (112). Holder 106 rotates around hinge 202. The optimal tilt angle θ (216) is reached when the plumes from both targets overlap on substrate 118, in other words, their axes 208 and 210 intersect on the surface of the substrate in point 220. The feedback loop comprises base 1701, linear actuator 1702, link 1703 connecting the actuator to tilting target holder 106, hinge 1704, digital video camera 1705 with line of sight 1706, computer (image processor) 1707, controller of the linear actuator 1708 connected to the linear actuator with an electrical cable through a vacuum feed through mounted on the wall of vacuum chamber 1709. Linear actuator 1702 is mounted on base 1701 holding stationary target holder 104. The actuator is mechanically connected to tilting target holder 106 with link 1703 that is joined to the holder with hinge 1704. Digital camera 1705 is mounted outside of the PLD vacuum chamber (not shown) on one of the flanges with an optical window in such a way that its line of sight 1706 passes through near the location of substrate 118 where plumes 114 and 116 from respective targets 110 and 112 must overlap. The digital camera is connected to computer (image processor) 1707. In case of semi- or automatic control of the plume overlapping, the computer is connected to controller 1708. During the PLD process, video camera 1705 captures the image of the plumes and sends it to computer 1707. The computer displays the image. In case of manual control, the operator manually adjusts the position of tilting target holder 106 with linear actuator 1702 in order to maintain plumes 114 and 116 overlapping on substrate 118, thus making sure of the consistency of the proportion of mixing the target materials from targets 110 and 112 in the deposited composite film. In semi-automatic mode, software in the computer analyses overlapping of the plumes and sends the warning message to the operator when the plumes do not overlap. Upon receiving the message, the operator manually corrects the problem. In automatic mode, the computer software analyses the image of the plumes to establish if they do not overlap and sends the signal to the linear actuator controller to tilt target holder 106 until the plume overlapping is reached. 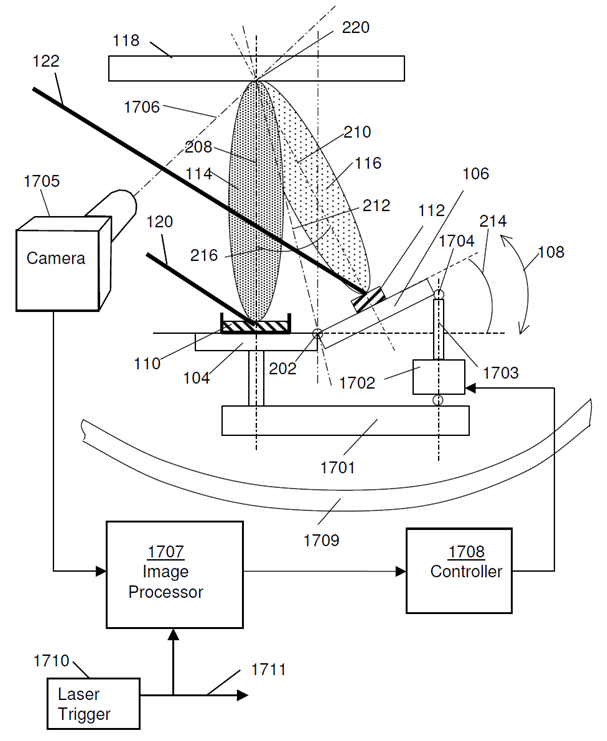 | Figure 6. Schematic of the plume overlapping control system |
The spherical vacuum chamber of the PLD system is connected to a two-stage vacuum pumping system including a mechanical and turbo pumps. The chamber has provisions for filling an inert gas in during the PLD process. However, no inert gas was used in this work. The setup was used to make polymer-inorganic films using the proposed DB-MAPLE method. A sample of the solution of PMMA and dye PR in GBL (-44°C freezing point) was poured in a copper cup of the MAPLE target holder (1-st target 110 in Figure 6)) and frozen with circulating liquid nitrogen. Then the copper cup with the frozen polymer-dye solution was mounted and installed in the vacuum chamber. The second target 112 was made of a solid pellet prepared by compressing the powder of the upconversion phosphor and retained in the second, tilted target holder 106. The laser source was a Spectra Physics Quanta Ray Nd:YAG Q-switched Lab-170-10 laser with a pulse repetition rate of 10 Hz, 850-mJ energy per pulse at the 1064-nm fundamental wavelength and 450-mJ energy per pulse at the 532-nm second harmonic. The targets were exposed to the fluences ranging from 0.053 J/cm2 to 0.84 J/cm2. Frozen polymer Target 1 was ablated with the 1064-nm laser beam. Target 2 was concurrently ablated with the 532-nm frequency doubled Nd:YAG beam. The fluences were adjusted to control the proportion of the upconversion material coming in the polymer at approximately 5% by weight.
2.4. Properties of the Nano-composite Films
The morphological and optical properties of the nano-composite reagent films of PMMA-PR: NaYF4: Yb3+, Er3+ prepared by the DB-MAPLE method were similar to those described elsewhere [11, 12]. The dominant crystalline phase of the embedded nano-particles of the inorganic phosphor compound (fluoride host of the RE ions) was the hexagonal β-phase, the most favourable for the efficient visible upconversion fluorescence. The average size of the phosphor nano-particles was 5-10 nm. They were evenly distributed in the polymer matrix. The average thickness of the films was close to 20 nm. Being illuminated with a 980-nm laser diode, the films exhibited bright visible upconversion emission with the spectrum similar to that of the bulk phosphor powder (Figure 2).
3. Experimental Setup
The prepared reagent nano-composite films were tested as ammonia sensors using the experimental setup schematically depicted in Figure 7. The photograph of the general view of the setup is presented in Figure 8. 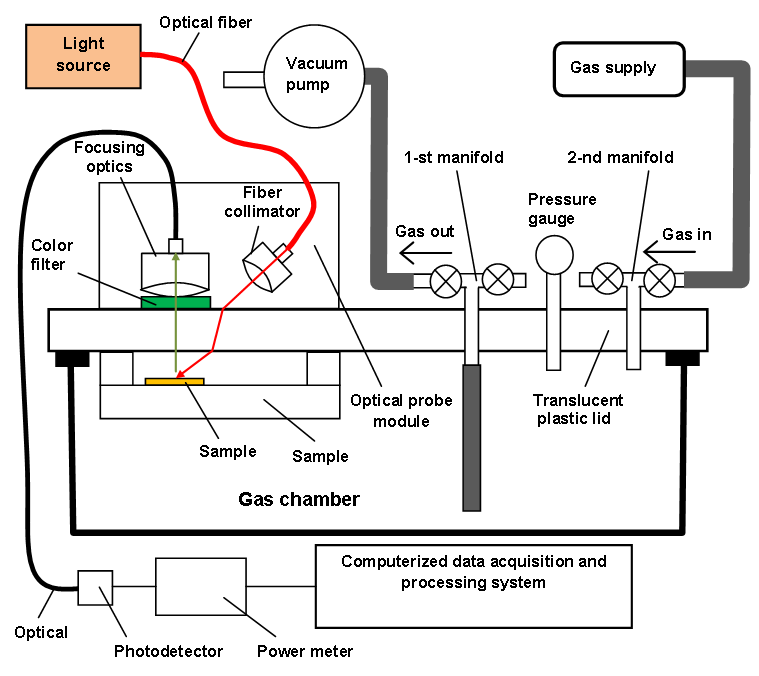 | Figure 7. Schematic of the experimental setup to investigate the nano-composite films for sensing ammonia |
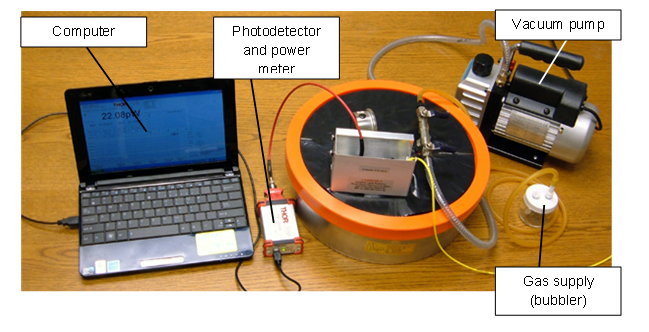 | Figure 8. Photograph of the experimental setup to investigate nano-composite films for chemical sensing |
The reagent film deposited onto a silicon substrate (Sample) was placed inside a sealed gas chamber. It was illuminated with a 980-nm radiation from a 300-mW laser diode. The radiation was delivered from the laser diode via a single-mode optical fiber terminated with a collimator. The collimated infrared beam was sent to the film through a transparent plastic lid of the chamber. The green component of the upconversion radiation from the film passed through the transparent lid and got collected by the focusing optics that sent it through a multimode fiber to a silicon photodetector. The signal from the photodetector was digitized in a power meter module and sent to a computerized data acquisition and processing system.“Gas supply” was comprised of a mixture of ammonia and ambient air drawn through a water bubbler and further in the gas chamber with a vacuum pump.
4. Results and Discussion
The nano-composite reagent films were exposed to ammonia using the above-mentioned setup. Figure 9 presents a typical response (the drop of the intensity of the green upconversion emission recorded with a power meter using a silicon photodetector) to ammonia (~5% molar concentration of ammonia in air). The relative humidity (RH) of the air during the exposure was ~ 50%. The film response to ammonia was not significantly affected by the variation of RH between 30 and almost 100%. Typical response time to the exposure was ~ 5 min.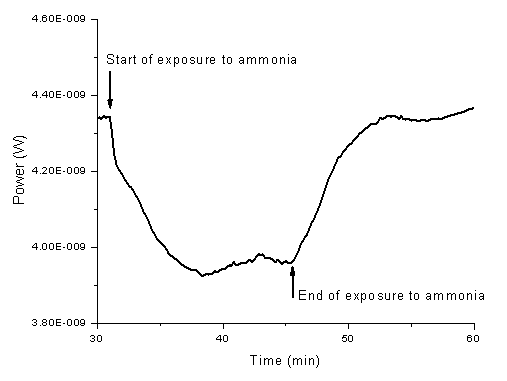 | Figure 9. Time plot of the response of the sample nano-ocomposite reagent film to the exposure to 5% ammonia in air |
Figure 10 presents a typical calibration plot “Ammonia concentration vs. Sensor Output”. The absolute value of the drop of the power of the upconversion emission –ΔP (in nanoWatts) from the exposed reagent film was chosen as the sensor output. The plot is nonlinear and can be approximated with a quadratic curve (solid line). The nonlinearity of the response is typical for colorimetric sensors [9] and is an advantage when dealing with very wide range of possible exposure (from zero to 100% NH3). The reagent film has the capability of recovering from the exposure to very high ammonia concentrations without experiencing any irreversible damage.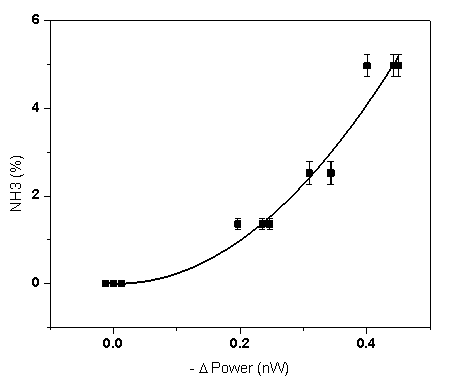 | Figure 10. Ammonia concentration plottet versus the sensor output. Solid line presents the approximation quadratic calibration curve |
Based on the level of the fluctuations of the output readings, the sensitivity of the sensor could be estimated as ~ 0.4% of ammonia in air or 8% of the range 0 to 5% ammonia. In order to make the proposed reagent films suitable for such applications as early detection of ammonia leaks, evaluation of exposures of human operators or animals to hazardous environment, the sensitivity must be ~ 0.0001% (1 ppm) or less. There are several ways of improving the sensitivity: increasing signal-to-noise ratio with the use of proper signal processing methods; increasing the thickness of the films, increasing the concentration of the indicator dye, and using the indicator dye more sensitive to ammonia.
5. Conclusions
It has been thus demonstrated for the first time, to the authors’ knowledge, that the reagent nano-composite films prepared by the new DB-MAPLE method of simultaneous co-deposition of a polymer-indicator dye mixture and a RE-based upconversion phosphor could be used in chemical sensors of ammonia in air. The sensing effect is based on the increased absorption of the visible upconversion emission from the phosphor nano-particles (illuminated by an infra-red laser diode) by the indicator dye exposed to ammonia. Preliminary testing indicated that the sensor based on such films had a sensitivity of ~ 0.4% of NH3 in air and a response time of ~ 5 min. Such sensor is suitable for the applications that include detection and alarming on severe leaks from ammonia storage vessels (pressurized tanks), industrial refrigeration, and industrial ammonia transportation pipelines. Further improvement of the sensitivity might extend potential applications to the early leak detection and environment pollution hazardous to humans and animals.
ACKNOWLEDGEMENTS
The authors like to acknowledge the support from DoD AFOSR grant FA9550-12-1-0068, FA9550-12-1-047, Army W911NF-14-1-0093, W911NF-11-1-0192, and NSF award # HRD 1002541. The authors like to thank Metal Fab south Inc. Mr. Jason and Kevin Bergeron for their invaluable machining service to the project.
References
[1] | Air Emissions from Animal Feeding Operations. National Research Council. Washington, D.C.: The National Academies Press. 2003. |
[2] | L. J. M. Van der Eerden, P.H.B. de Visser, and C.J. Van Dijk, “Risk of damage to crops in the direct neighborhood of ammonia sources,” Environmental Pollution, vol. 102(S1), pp. 49-53, 1998. |
[3] | “What is Visibility Impairment?” Washington, D.C.: United States Environmental Protection Agency. [Online]. Available: www.epa.gov/oar/visibility/what.html. Accessed 21 October 2004. |
[4] | R.W. Battye, C. Overcash, and S. Fudge. “Development and Selection of Ammonia Emission Factors.” EPA/600/R-94/ 190. Final report prepared for U.S. Environmental Protection Agency, Office of Research and Development. USEPA Contract No. 68-D3-0034, Work Assignment 0-3. 1994. |
[5] | National Ambient Air Quality Standards for Particulate Matter; Final Rule, EPA, Federal Register. 62(138): 38651, 1997. |
[6] | Arizona Ambient Quality Guidelines. [Online]. Available: http://www.azdeq.gov/environ/air/permits/download/ambient.pdf. |
[7] | “Final Meeting Minutes.” U.S. EPA Environmental Technology Verification Program, Advanced Monitoring Systems Center, Air Stakeholder Committee Meeting, September 24, 2003, Crystal City, VA. 2003. |
[8] | “Guide for selection of chemical agent and toxic industrial material detection equipment for emergency first responders”, Guide 100-04, Department of Homeland Security. Washington, D.C. 2005. |
[9] | S. S. Sarkisov, M. Czarick III, B. D. Fairchild, Yi Liang, T. Kukhtareva, M. J. Curley, “Colorimetric polymer-metal nanocomposite sensor of ammonia for the agricultural industry of confined animal feeding operations, “ Opt. Eng., vol. 53 (2), pp. 021107-1 - 021107-10, Feb. 2014. |
[10] | H.S. Mader and O.S. Wolfbels, “Optical ammonia sensor based on upconverting luminescent nanoparticles,” Anal. Chem., vol. 82, pp. 5002-5004, 2010. |
[11] | A.M. Darwish, M.T. Sagapolutele, S. Sarkisov, D. Patel, D. Hui, and B. Koplitz, “Double beam pulsed laser deposition of composite films of poly(methyl methacrylate) and rare earth fluoride upconversion phosphors,” Composites Part B: Engineering, vol. 55, pp. 139–146, 2013. |
[12] | A.M. Darwish, A. Burkett, A. Blackwell, K. Taylor, S. Sarkisov, D. Patel, B. Koplitz, and D. Hui, “Polymer-inorganic nano-composite thin film upconversion light emitters prepared by double-beam matrix assisted pulsed laser evaporation (DB-MAPLE) method,” Composites Part B, vol. 68, pp. 355–364, 2015. |
[13] | J.F. Suyver, J. Grimm, K.W. Kramer, H.U. Gudel, J. Lumin. 114, pp. 53-59, 2005. |
[14] | G. Yi, H. Lu, S. Zhao, Y. Ge, W. Yang, D. Chen, and L. Guo, Nano Letters vol. 4 (11), pp. 2191-2196, 2004. |