Somia M. Abad-Elwahad 1, Ali F. Bukhzam 2, Gamal A. H. Mekhemer 3
1Chemistry Department, Faculty of Science, El-Marj University, Benghazi, Libya
2Chemistry Department, Faculty of Science University, Benghazi, Libya
3Chemistry Department, Faculty of science Minia University, El-Minia, Egypt
Correspondence to: Somia M. Abad-Elwahad , Chemistry Department, Faculty of Science, El-Marj University, Benghazi, Libya.
Email: |  |
Copyright © 2015 Scientific & Academic Publishing. All Rights Reserved.
Abstract
Alumina–supported cobalt (II) oxide catalysts were prepared by using alumina gel and alumina as support materials and aqueous cobalt (II) nitrate hexahydrate as the impregnating solution or a precursor at different loading levels in the range 3–15%. Co3O4 were prepared by wet impregnation method and drying in an oven at 380 k for 16 hours followed by calcination at 870 K for 3h in air. The catalysts characterized by different technique like X–ray diffractometry, Ex–situ fourier transform infrared spectroscopy and nitrogen sorptiometry at 77 K. The adsorption data were analyzed by the BET method. The obtained data show that the catalysts prepared from cobalt nitrate have two different crystal phase assigned to Co3O4 and CoAl2O4 at15% loading level. Ex–situ FTIR spectroscopic analyses of the supported catalysts were performed to get more information about the surface cobalt oxides loading up to 15 wt% exist as high intensity were results cobalt nitrate suggested three bands indicated to formation of the cobalt (II) oxide but are clearly exhibit in CoAlON than CoAlN.
Keywords:
Characterization, Impregnation mothed and alumina supported and the adsorption data were analyzed by the BET method
Cite this paper: Somia M. Abad-Elwahad , Ali F. Bukhzam , Gamal A. H. Mekhemer , A Study of the Precursor Variation on the Surface Characterization of Supported Cobalt Oxide Catalyst, American Journal of Materials Science, Vol. 5 No. 2A, 2015, pp. 1-5. doi: 10.5923/s.materials.201501.01.
1. Introduction
Catalysis can be an important step in removing key contaminants, both from power plant effluent and from process plants, contributing to efficient energy production and utilization. Catalysis is also important to the chemical and petroleum industries, where advances in catalysis have created many new products and processes. Catalysis, as it is applied to the industrial production of materials, is both, broad and complex. It has significant implications on process costs and product properties, thereby affecting the competitiveness of the catalytic technology (via intellectual property).Catalysis is responsible for the efficient, low cost manufacture of products as diverse to each other as gasoline is to aspirin [1]. Catalysis can be classified into two main groups: homogenous and heterogeneous catalysis. Homogenous catalysis mainly occurs in the liquid phase, where the catalyst can be mixed completely with the reactants up to the molecular scale. The reactants and the catalyst are liquids or dissolved in the liquid phase. In heterogeneous catalysis, the catalyst is usually a solid and the reactants are gases or liquids, thus the phases are separated. Practical heterogeneous catalysts usually consist of metal or metal oxide particles stabilized on a high surface area support material.Unsupported cobalt oxide is a very active catalyst in the field of air pollution control for total removal of organic pollutants from effluent stream. Cobalt oxide is also used as the promoters for alumina supported molybdenum catalysts. In addition, the cobalt oxide is used as a reactant in the formation of certain technically important spinels [2]. According to the results obtained, the synthesized cobalt oxide system is prominent and useful in a wide field of applications in environmental protection. From a practical point of view it is important to investigate the catalytic properties of this oxide system upon deposition on support materials. In general, crystalline cobalt oxides exist in three different phases with stoichiometry of CoO, Co2O3 and Co3O4.The induced changes in the catalytic activity of cobalt oxide due to doping with small amounts of foreign oxides have been attributed to dissolution of some oxide added to the lattice of cobaltic oxide solid forming solid solutions with subsequent changes in the oxidation state of cobalt ions in Co3O4. The doping process may lead to an increase or decrease in the oxidation state of cobalt ions in Co3O4 depending on the valence state of dopant added. The resulting increase in the oxidation state of cobalt ions due to doping affected an increase in the catalytic activity of the treated Co3O4 solid while the possible decrease in the oxidation state of cobalt ions led to a decrease in its catalytic activity [3].It is known that Co3O4 and CoO are the stable oxides in the cobalt oxide system, while the valence of cobalt higher than +3 is thermally unstable. Some literatures reported the special methods to obtain higher valence cobalt oxides, such as Co2O3 and CoO2 [4]. However, these methods always end up with either a mixture of CoO and Co2O3 or a mixture of Co2O3 and CoO2.
2. Experimental
2.1. Materials
Cbalt (II) nitratehexahydrate(CoN), Co(NO3)2.6H2O, FERAK BERLIN,used as a precursor for supported and unsupport CoOx catalysts.Aluminum hydroxide Al(OH)3. xH2O, drygel was purchased from SURECHEM Products LTD (SCP, England), used as supplied. High surface area of γ - alumina was obtained by calcinations of alumina gel (SCP) at 870 K for 3 h, in static atmosphere of air.Nitrogen gas:used as the adsorbate for the surface area measurements (see below) was supplied by the Industrial Gases Company (Cairo). Traces of oxygen were removed by passing the gas over a well packed column of reduced copper turnings heated at 620 K. This was followed by freezing traces of moisture in a trap filled with glass wool and immersed in liquid nitrogen.
2.2. Physicochemical Characterization of Catalysts
2.2.1. X-ray Powder Diffractometry (XRD)
XRD is a technique used for the identification of the bulk structure of macro–crystalline materials, which is used on measurement of structure–sensitive high energy X–ray radiation. X-ray powder diffractograms were recorded, using Ni–filtered CuKα- radiation (λ = 1.54056 Å); on a JSX – 60 PA Jeol X–ray spectrometer. The generator at 35 Kv and 20 mA, and the diffractometer at 2θ diverging and receiving slits and at a scan rate of 20 mm/ min. The sample ground to a particle size less than 44 μ and packed into the wall of a sample holder, and then mounted in a horizontal position. Diffraction patterns (I/I0 vis. d-spacing (Å)) derived from the powder diffractograms were matched with relevant ASTM-standard patterns. Crystallite sizing (Dhkl) was carried out using the line broading technique in conjunction with Scherrer's formula:  | (1) |
Where, Bhkl is the width at half peak maximum in radians, is the incident wavelength (λ = 1.54051 Å) and θ is the diffraction angle.
2.2.2. Ex-situ Fourier–transform Infrared Spectroscopy
Ex–situ FT–IR spectra of supports, unsupported and supported catalysts were taken at frequency range 4000–400 cm-1. This was done at a resolution of 4 cm-1 using a model Genesis–II FT–IR Mattson Fourier- transform infrared spectrometer (USA) and an online PC with win first Lite (V 1.02) software for spectra acquisition and handling. The spectra were taken for thin ( > 20 mg / cm ) lightly loaded ( > 1–Wt.% ) wafer of KBr–supported test samples.About 1–2 mg of the catalyst as a fine powder was mixed well with spectroscopy pure KBr powder and was finally grinded in agate mortar.
2.2.3. Specific Surface Area Measurements (SBET)
The specific surface area of alumina supported cobalt oxide catalysts were determined by applying the linear form of the Brunauer, Emmett and Teller (BET) equation [24] to the adsorption date;  | (2) |
Where P = adsorption equilibrium pressure P0 = Saturated vapour pressure of adsorbate at its boiling Point, Vads = Volume at STP occupied by molecules adsorbed at pressure p, Vm = Volume of adsorbate required for a monolayer coverage and C =Constant related to heat of adsorption of adsorbate in 1st a subsequent layers. Nitrogen adsorption/desorption isotherms were determined volumetrically at 77K using an automatic, multi-station Nova 2000gas sorptiometer (Quantachrome Corporation, Boynton Beach, FL, U.S.A.) and employing test samples which had been pre-outgassed at 470 K and 10−5 mbar pressure for 1h. The reproducibility of the resulting isotherms was better than 98%.
3. Results and Discussion
3.1. X-ray Powder Diffraction (XRD)
X–ray powder diffraction of pure alumina and alumina supported cobalt oxide prepared from alumina gel as a support and cobalt (II) nitrate as a precursor of cobalt oxide (xCoAlN) at different loading levels are shown in Fig. 1. The XRD patterns of alumina displays a few weak broad peaks which are indicative of fine crystallites of the γ–Al2O3 phase (JCPDS 29–1486). The average crystallite size derived from the peak width at half maximum (PWHM) was ca. 10.3 ± 1 nm. X–ray diffractograms of the calcined products of supported catalysts CoAlN at 870 K for 3 h at different loading levels samples are shown in Fig. 1. The XRD patterns obtained for the alumina supported cobalt oxide catalysts (xCoAlN) display different peaks due to the alumina and cobalt oxide. The patterns display peaks at 2θ =19, 31.2, 36.9, 44.8, 55.6, 59.3 and 65.2, approximately. These peaks are due to Co3O4 and/or CoAl2O4 phase (JCPDS 43-1003 and 38-0814, respectively). These peaks become more intense as the cobalt oxide content of catalyst increased (see Fig. 1). These results suggest that the cobalt phases present in the cobalt–containing alumina catalysts have a spinel structure, which could be assigned to Co3O4 and/or CoAl2O4 phases. However, due to the structural similarity of these two phases, it is not possible to distinguish between one spinel phase and the other based on XRD data only [5]. No signal corresponding to cobalt monoxide phase (CoO) were detected by XRD analysis.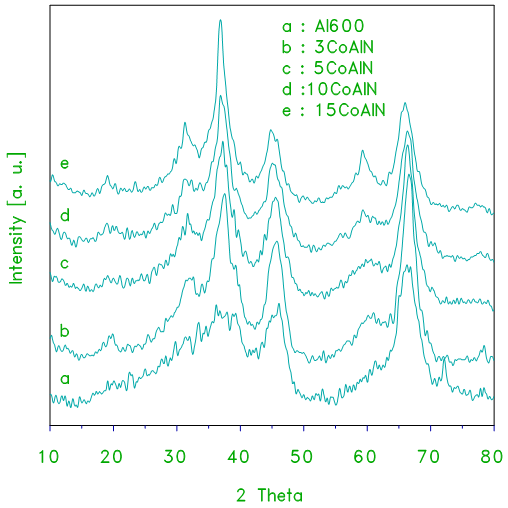 | Figure 1. XRD patterns obtained for alumina and the indicated set of alumina-supported cobalt oxide (xCoAlN) catalysts prepared from cobalt nitrate and alumina gel used as a support |
The XRD patterns obtained for pure and alumina supported cobalt oxide prepared from crystalline alumina as a support and cobalt (II) nitrate as a precursor of cobalt oxide (xCoAlON) at different loading levels are shown in Fig.2. They were basically very similar to those obtained for their alumina supported cobalt oxide xCoAlN catalysts (Fig. 1). The XRD difference is the intensity of these peaks are more intense than the peaks for the patterns of xCoAlON catalysts. This mean that the degree of crystallinity of the obtained phase increases with increasing the loading level of cobalt oxide, after which Co3O4 and/or CoAl2O4 may be formed. Also, the particle size of Co3O4 and/or CoAl2O4 in supported cobalt oxide catalysts prepared from alumina gel as a support is slightly lower than the catalysts prepared from crystalline alumina used as a support. Indeed, no peaks assigned CoO phases were detected in the diffractogram of xCoAlON catalysts. In the XRD pattern of the cobalt-containing cobalt-alumina catalysts (xCoAlON), it is noted a peak in 2θ = 36.9, which indicates the presence of cobalt phases with a spinel structure, such as Co3O4 and/or CoAl2O4. These results are in good agreement with the results obtained by Trigueiro et al. [6].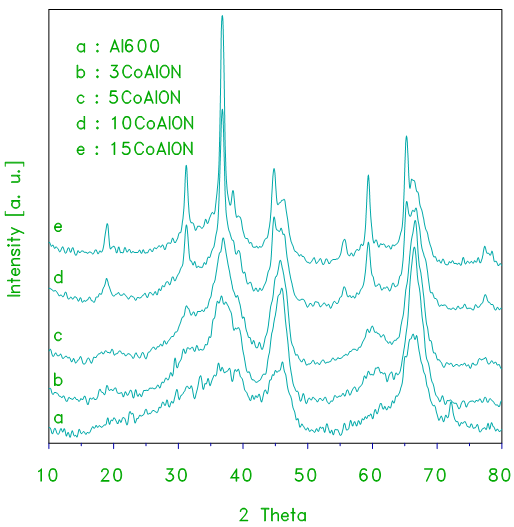 | Figure 2. XRD patterns obtained for alumina and the indicated set of alumina-supported cobalt oxide (xCoAlON) catalysts prepared from cobalt nitrate and crystalline alumina used as a support |
3.2. Ex-situ FTIR Spectra of Alumina Supported Cobalt Oxide Catalyst
Ex-situ FTIR spectroscopic analysis of the supported catalysts were performed to get deep information about the surface cobalt oxides. Fig.3, provides a comparison of the IR spectra obtained for the xCoAlN samples, with those exhibited by alumina and cobalt oxide. The IR spectrum of pure cobalt oxide is shown in Fig.3. IR spectrum of cobalt oxide displays three bands at 668, 580 and 520 cm-1 which are assigned to Co3O4 [7–8]. The spectrum of pure alumina displays two strong, broad absorptions centered at 765 cm-1 and 580 cm-1 in common. These frequencies may be attributed to the Al–O lattice vibrations of Al2O3 [9]. As the loading level of cobalt oxide is increased, these three absorptions gradually narrow with the emergence of three much strong absorptions (at 668, 580 and 520 cm-1) resembling those shown for Co3O4 surface species in the spectra of alumina supported cobalt oxide, (Fig.3.) In fact IR spectra shown in Fig.3 clearly exhibit three bands at 668, 580 and 520 cm-1 which were reported [7] to be assigned to the dominance of Co3O4 and/or CoAl2O4. However, the weak absorption band at 1386 cm-1 still remains while the Co3O4 absorption bands are clearly displayed. The persistence of the absorption band at 1386 cm-1 indicate the presence of some residual nitrate. Such residual nitrate may be attributed to the presence of strongly adsorbed surface species and/or a minute amount of soluble nitrate component that perhaps had been trapped within the porous structure of the developing oxide. Similar behavior of nitrates has been reported elsewhere [9].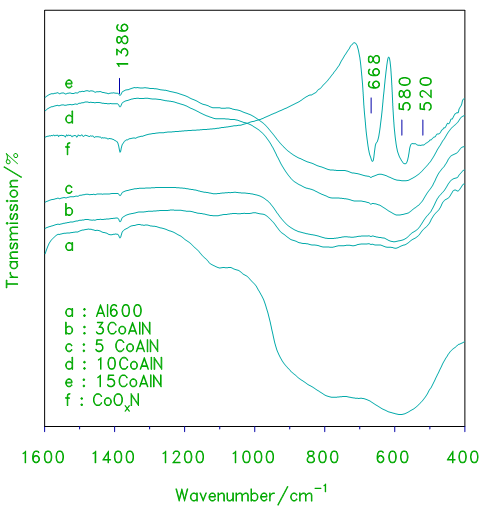 | Figure 3. FT–IR transmission spectra obtained for cobalt oxide, crystalline alumina and the indicated set of alumina supported cobalt oxide prepared from nitrate xCoAlN catalysts |
While, the IR spectra of alumina supported cobalt oxide used crystalline alumina as a support are shown in Fig.4. The FTIR data displays similar spectra of xCoAlN catalysts. The difference in the intensity of three bands are more intense than the spectra of xCoAlN catalysts. This indicates that the formation of cobalt oxide of higher bond order in xCoAlON catalysts than in xCoAlN catalysts.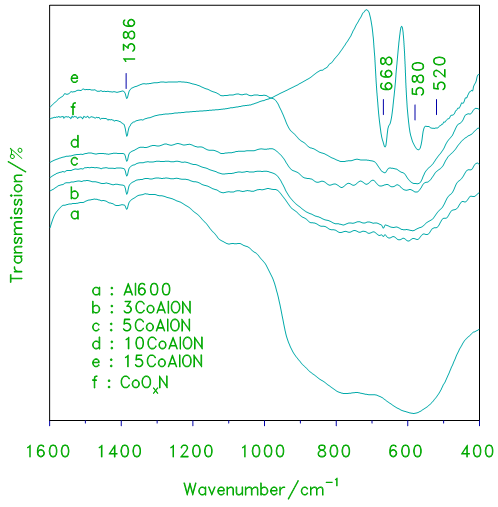 | Figure 4. FT–IR transmission spectra obtained for cobalt oxide, crystalline alumina and the indicated set of alumina supported cobalt oxide prepared from nitrate xCoAlON catalysts |
3.3. Measurement of Surface Areas
The different surface characteristics, namely specific surface area (SBET), total pore volume (VP) and Average pore diameter (Å) were determined from nitrogen adsorption isotherms measured at 77 K [10]. The results obtained are given in Table 1. The data show a gradual decrease in the surface areas of alumina supported cobalt oxide catalysts (when used alumina gel or crystalline alumina as a support, see Table 1). In view of the above one would conceive the relatively high values of the surface area of alumina supported cobalt oxide used crystalline alumina as a support as they compared with the corresponding ones for the alumina supported cobalt oxide used alumina gel as a support. It is clear from table. 3, that the surface area for all catalysts decreases with increases of cobalt oxide loading levels. The measurement of SBET shows dramatic decrease in the surface area with increase of cobalt oxide levels as shown in table 1.Table 1. Texture data of alumina supported cobalt oxide catalysts obtained from nitrogen adsorption-desorption data at 77 K 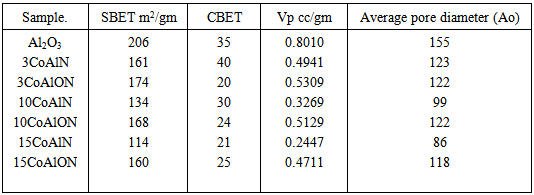 |
| |
|
This decrease can attributed to the closing of the pores of the support caused by crystallites of the cobalt oxide formed during calcination. One can assume that as the cobalt oxide increases the size of supported crystallites also increases and this, in turn, brings about an increasing plugging of the porous of the support. This actually confirmed by XRD results.
4. Conclusions
XRD results of alumina supported cobalt oxide show the formation of crystalline Co3O4 and/or CoAl2O4. Also, the formation of Co3O4 and/or CoAl2O4 on alumina supported cobalt oxide prepared from cobalt nitrate using as a precursor while IR spectra of alumina supported cobalt oxide catalysts show increase the formation of Co3O4 on the surface by increasing the loading level of cobalt oxide (up to 15 wt%).The surface area of alumina supported cobalt oxides decrease by increase of the loading level of cobalt oxides in two series. The surface area obtained from alumina gel is lower than of pure crystalline oxide. The particle sizes increases, while the total pore volume of these samples are decreases with increasing the loading level of cobalt oxide. The average pore diameter of catalysts using alumina gel as a support is decreased with increasing the loading levels of cobalt oxide, while no change was observed on the average pore diameter of the catalysts using crystalline alumina as a support.
References
[1] | I. Chorkendorff, J. W. Niemantsverdriet, Concepts in Modern Catalysis and Kinetics, (WILEY-VCH, Weinheim, 2003). |
[2] | G.A.H. Mekhemer, H.M.M. Abd-Allah, S.A.A. Mansour Coll. And Surf. A: Phy. Eng. Aspe., 160 (1999) 251–259. w.w.w.elsevier.com/locate/colsurfa. |
[3] | H. G. El–Shobaky, M. A. Shouman, A. A. Attia. Coll. And Surf. A: Phy. Eng. Aspe., 274,(2006), 62–70. w.w.w.elsevier.com/locate/colsurfa. |
[4] | H.–K. Lin, H.–C. Chiu, Hs.–Ch. Tsai, Sh.–Hu. Chien and C.–B. Wang, Catal. Let., 88, (2003), 3–4. 1011-372X/03/ 0600–0169/0 # 2003 Plenum Publishing Corporation. |
[5] | W. M. Shaheen, M. M. Selim. International Journal of Inorganic Materials 3, (2001), 417–425. |
[6] | F. E. Trigueiro, C. M. Ferreira, J.–C. Volta, W. A. Gonzales, and P. G. Pries de Oliveria, Catal. Today, 118, (2006) 425. |
[7] | G. A. H. Mekhemer, H. M. M. Abd–Allah, S.A.A. Mansour Colloids and Surfaces A: Physicochemical and Engineering Aspects 160, (1999), 251–259. |
[8] | H.P. Boehm, H. Knozinger, in: J.R. Anderson, M. Boudart (Eds.), Catalysis Science and Technolgy, vol. 4, Springer, Berlin, 1983, p. 38. |
[9] | C.–B. Wang, Ch.–W. Tang, Sh.–J. Gau, and S.–H. Chie CatalysisLettersVol.101,(2005), Nos. 1011-372X/05/0500 – 0059/0 _ 2005 Springer Science+ Business Media, Inc. |
[10] | M. N. Ramsis, Ch. A. Philip, M. Abd El Khalik and E. R. Souaya. Journal of Thermal Analysis, Vol. 46, (1996), 1775– 1788. |