Esam A. Gomaa
Chemistry Department, Faculty of Science, Mansoura University, 35516, Mansoura, Egypt
Correspondence to: Esam A. Gomaa, Chemistry Department, Faculty of Science, Mansoura University, 35516, Mansoura, Egypt.
Email: |  |
Copyright © 2012 Scientific & Academic Publishing. All Rights Reserved.
Abstract
The molal solubility for saturated solutions of glycine at 298.15 K in various solvents was determined. The solvents used are, water (W), dimethylsulphoxide (DMSO), ethanol (Et), 1-4, dioxane (Di), acetonitrile (AN), N,N-dimethylformamide (DMF),acetone (Ac) and methanol (Me) . From the experimental data for solubility, pH and densities. the different volumes, free energies, dissociation constants, association constants and solvation parameters were estimated. The different types of volumes for glycine in different solvents like molar volume, Van der Waals and electrostriction volumes were evaluated. Also the solvation numbers were also cited for glycine in the used solvents. All the solubility parameters estimated explain the different ion solvent and ion ion interactions to help any analyst to analyse glycine. Different association, dissociation parameters were calculated using model explained before by us concentrating glycine zwitter ion has effective hydrogen atom. The results were also discussed.
Keywords:
Solubility, Association Constant, Dissociation Constant, Free Energies, Organic Solvents, Densities, Different Volumes, Glycine
Cite this paper: Esam A. Gomaa, Dissociation, Association and Solvation Parameters for Saturated Glycine in Various Solvents at 298.15 K, Science and Technology, Vol. 3 No. 4, 2013, pp. 123-126. doi: 10.5923/j.scit.20130304.05.
1. Introduction
Knowledge of the existence of amino acids dates back over a century in many cases, as does knowledge of their extended in proteins. When amino acids were discovered, their identity was established by isolating and purifng the individual compounds and obtaining elemental analysis. After the advent of paper chromatography, this technique was used with a variety of different solvents to identify elution characteristic and demonstrate the purity of isolated compounds. Amino acids were located by the use of a reagent that produced colour with the compound. The most common reagent used for locating amino acids is ninhydrin. Which produces a purple colour with imines, a pink or yellow colour with amino acids, and various intermediate colours, with compounds containing an amino group and a sulphonic group.It also reacts with small peptides such as glutathione. The techniques of paper chromatography were applied to the separation of mixtures of amino acids, such as the components of the protein after hydrolysis, and then the separation of free amino acids in physiologic fluids and tissues.It was extended by the use of two dimensional chromatography, in which a different solvents were used in each direction[1]. Later electrophoresis was employed as one of the separating techniques . After locating the amino acids by reaction with ninhydrin, the spots could be cut out, eluted with alcohol or acetone and measured spectrophotometrically[2]. In order to measure the free amino acids in biological tissues and fluids, all proteins must be removed. Various methods have been employed to accomplish this, such as ultra filtration, equilibrium dialysis, and proton precipitation. A variety of protein precipitation has been employed for this purpose, the most common being picric acid. For extracting tissues the most common methods employ 10% trichloroacetic acid , usually by blending or homogenizing with 5 vol of acid followed by a high speed centrifugation[3]. Plasma, cerebrospinal fluid and urine are most commonly deproteinized using 5 vol 3% sulphosalicylic acid as protein precipitate, again followed by centrifugation. The precipitated proteins can be purified by washing with hot trichloaceic acid to remove nucleic acids and with a Varity of organic solvents to remove lipids. The proteins can then be hydrolysed, usually with 6N HCl under vacuum at 120℃ for 24 h or more, and their amino acid composition determined[3].Many publications have done on the behaviour of weak acids in anhydrous solvents. Interesting work has been done by Kolthoff et al.[4,5]. Aleksandrov et al.[6,7] studied the dissociation of salicylic acid in butane-2-one. Kreshkov et al.[8] studied the dissociation of amino acids (as weak) acids) in mixtures of formic and ethylmethylketone and in mixtures of acetic acid-ethylmethylketone. Gomaa et al.[9] studied association, dissociation and hydrogen bonding of salicylic acid in water-N, N-dimethylformamide mixtures from solubility measurements.The aim of this work is to evaluate the solubility of glycine in different solvents and discuss in details the solvation parameters for the solubility process. Study the effect of different solvents of the solubility and the physical behaviour accompanied is very important for the biochemical analysers. Also apply a theoretical model for evaluating different microscopic interactions of glycine is necessary.
2. Experimental
The glycine and solvents used, dimethylsulphoxide (DMSO) , ethanol (Et), 1-4 dioxane (Di) acetonitrile (AN), dimethylformamide (DMF),acetone (Ac) and methanol (Me) were supplied from Merck. The saturated solutions of glycine were prepared by dissolving it in the solvents used. The solutions were saturated with N2 gas in closed test tubes. The tubes were placed in a shaking water bath of the type assistant for a period of four days, followed by another two days without shaking to reach the necessary equilibrium. The solubility of glycine in each solution was determined gravimetrically by taking 1 ml of the saturated solution and subjecting it to complete evaporation using small aluminium disks heated by an infrared lamp. The pH readings of the saturated solutions were measured using a pH-meter of the type Tacussel /Minis 5000. The densities were measured by using weighing bottle 1 ml and analytical balance (4 digits) of the type Mettler Toledo DA.
3. Results and Discussion
Amino acid contents have been studied in a variety of biological samples. Of these , plasma , urine , cerebrospinal fluid , which are accessible and clinically significant, have received most attention of these samples investigated include amniotic fluid , bloodcells,feces,sweat,saliva,hair,fingernails and fingerprints. Even small amounts of protein in biological samples interfere in chromatographic analysis and have been removed[1]. Therefore solubility measurements are very important to solve this problem[1].The calculated molal solubility (m) for glycine saturated solutions was given in Table (1) from at least three average measurements. Also the measured densities and pH values of the acid used are also listed in Table (1).The molar volumes (VM) of glycine were obtained by dividing the molar mass by the densities and their values are listed in Table (2). The packing density as reported by Kim et al.[10] and Gomaa et al.[11], i.e. the relation between Van der Waals volume (VW) and the molar volume (VM) of relatively large molecules (above 40) was found to be a constant value and equal to 0.661.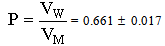 | (1) |
The electrostriction volume (Ve) which is the volume compressed by the solvent, was calculated by using equation (2) after Gomaa[12]. | (2) |
The molar, Van der Waals and electrostriction volumes of glycine in various solvents at 298.15 K were tabulated in Tables (2).The apparent molar volumes V[10,11] were calculated using equation (3)[13] | (3) |
Where M is the molar mass of glycine, m is the concentration; d and do are the densities of saturated solution and pure solvents, respectively. The values of V for glycine in various solvents at 298.15 K are presented in Table (2).The different association, dissociation parameters were calculated using model explained before by us concentrating glycine zwitter ion has effective hydrogen atom and that is clear from the pH values which are in weak organic acid range. Various analytical methods have been described in literature, only a few were developed for clinical use. For a good route analytical procedure, several factors have to be considered. Cost (both time and money), choice of simple reagent, simplicity of the procedure, and chromatographic properties of derivatives[2]. In this work simple and cheap methods for analysis can be used.Table (1). Molal solubilities (m), densities (d) and pH values for saturated glycine solutions in different solvents at 298.15 K |
| Solvent | m/(g.mol/kg solvent) | d | pH | H2O | 1.8752 | 1.0332 | 5.530 | DMSO | 0.1482 | 1.1060 | 13.250 | Et | 0.7028 | 0.7829 | 8.720 | Di | 0.2036 | 1.0147 | 4.060 | AN | 0.3048 | 0.7766 | 8.070 | DMF | 4.7051x10-5 | 0.9612 | 9.408 | Ac | 1.6979x10-4 | 10.7864 | 5.464 | Me | 0.0409 | 0.8075 | 6.929 |
|
|
Table (2). Molar volumes (VM), Van der Waals volumes (VW), electrostriction volumes (Ve) and apparent molar volumes (V) for saturated solutions of glycine in different solvents at 298.15 K (in cm3/mole) |
| Solvent | VM | VW | -Ve | V | H2O | 75.240 | 49.7336 | 25.5063 | 71.736 | DMSO | 68.463 | 45.2540 | 23.4043 | -162.827 | Et | 95.557 | 63.1632 | 32.3938 | -2250.5 | Di | 85.145 | 56.2824 | 28.8644 | -178.220 | AN | 96.578 | 63.8383 | 32.7400 | -164.168 | DMF | 79.447 | 52.5146 | 26.9326 | -2744.67 | Ac | 95.569 | 63.1713 | 32.3980 | -2546.5 | Me | 95.375 | 63.0428 | 32.3321 | -4309.1 |
|
|
The activity coefficient was calculated by using the relation
[14,15].Kass values were calculated[16-18] from the ratios of association constant to dissociation constant (i.e., K1/K2) for the dimmers of glycine which form a complex ion (
and hydrogen ion (H+), and the values of K` (where K` is the dissociation constant of the associated acid complex, H2A2) are given by the following equations | (4) |
 | (5) |
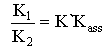 | (6) |
Where a is the activity. The values obtained K1/K2, K` and Kass are reported in Table (3). The maximum value of Kass was found to be by using DMF where water is the least association parameters.The free energies of dissociation (ΔGd), free energies of association (ΔGA), difference free energies (ΔΔG) and free energies of solvation (ΔGs) for glycine saturated solutions in various solvents were calculated by using the following equations and tabulated in Table (4).Amino acids are strongly polar compounds with a variety of functional groups and can not be analysed by a GC method without prior conversion into suitable volatile derivatives. The preparation of such derivatives should be simple, rapid, and reproducible and give high product yield. | (7) |
 | (8) |
 | (9) |
 | (10) |
 | (11) |
The solvation volumes for glycine were evaluated from the difference between Van der Waals of benzoic acid in absence and given before in various solvents. The Van der Waals volume of glycine was calculated from Bondi method[19] and found to be 106.4361 cm3/mole. Subtracting this value from Vw in solvents and divide the results by the molar volumes of the solvents taken from ref.[18], n (the solvation number) was obtained and given in Table (5).Table (3). Log activity coefficients (log γ±), dissociation constants (K) and association constants for benzoic acid saturated solutions in different solvents at 298.15 K |
| Solvent | log γ± | Pa | K` |

| Kass | H2O | -0.6932 | 6.2232 | 1.0176x10-13 | 1299241 | 1.2766x1014 | DMSO | -0.1904 | 13.4404 | 5.9974x10-26 | 25.2222 | 4.2055x1026 | Et | -0.1342 | 8.8541 | 3.9625x10-8 | 7.7016 | 1.9434x108 | Di | -0.2284 | 4.2884 | 6.3163x-8 | 3.5973 | 5.6953x107 | AN | -0.2285 | 8.2985 | 6.0307x10-10 | 7.6099 | 1.2619x1016 | DMF | -3.2769x10-3 | 9.4113 | 6.7511x1011 | 5.0838 | 7.5303x1016 | Ac | -6.5959x10-3 | 5.4706 | 3.9719x10-4 | 1.7005 | 4.2814x103 | Me | -0.1024 | 7.0314 | 5.1151x1012 | 5.5406 | 1.0831x1011 |
|
|
Table (4). Free energies of dissociation (ΔGd), free energies of association (ΔGA), difference free energies (ΔΔG) and free energies of salvation (ΔGs) for glycine saturated solutions in different solvents at 298.15 K (in k Joule/mole) |
| Solvent | ΔGd | ΔGA | ΔΔG | ΔGs | H2O | -1.5580 | 74.1698 | -80.5279 | -6.3576 | DMSO | -4.7342 | 143.9851 | -151.9873 | 8.0023 | Et | -6.5822 | 42.25559 | -47.3169 | -5.0611 | Di | -3.9458 | 41.0999 | -44.2739 | -3.1704 | AN | -3.9305 | 86.8843 | -91.9580 | -5.0315 | DMF | -24.7009 | 58.0610 | 62.0925 | -4.0314 | Ac | -21.5196 | 19.4153 | -20.7316 | -1.3163 | Me | -7.9248 | 64.4577 | -68.7025 | -4.2448 |
|
|
Table (5). Molar volumes of solvents (Vs), difference in different Van der Waals volumes (Vw) and solvation numbers (ns) for glycine saturated solutions in different solvents at 298.15 K |
| Solvent | Vs | ΔVw | ns | H2O | 18.0724 | 56.8643 | 3.1466 | DMSO | 71.2995 | 61.3399 | 0.8604 | Et | 58.6804 | 43.4308 | 0.7401 | Di | 86.3552 | 50.3126 | 0.5826 | AN | 52.8450 | 42.7557 | 0.8091 | DMF | 77.4118 | 54.0794 | 0.6985 | Ac | 63.0510 | 43.4226 | 0.6887 | Me | 40.7322 | 43.5511 | 1.0692 |
|
|
4. Conclustions
It was concluded that the solute-solvent interaction increased by increasing ΔΔG and ΔGs due mainly to the greatest of the association parameters in the corresponding solvents.Table (5). Molar volumes of solvents (Vs), difference in different Van der Waals volumes (ΔVw) and solvation numbers (ns) for glycine saturated solutions in different solvents at 298.15 K .Also it was observed that the association , dissociation and microscopic interactions between solute and solvent is important for any solvent individually, whether it is polar , aprotic or amphiprotic. Increasing of the solvation numbers favour more solute- solvent interactions between glycine and solvents. Also small solvation numbers favour more solute solute interaction or ion pair formation resulting in the decrease of the solute–solvent interactions in the solvent under discussion. This work give a lot of data which help any analyst to use suitable solvent for glycine in chromatographic analysis.
References
[1] | Volker, F. Wedish,”Amino acid biosysthesis-pathways, regulation and metabolic enegineering”, Springer – Verlag , Berlin,Heidelberg (2007). |
[2] | Alan A.Bculton,Glen B. Baker and James d. Wood,”Neuromethods 3 ,amino acid “.,The Humana Press Ins ,Clifton ,NJ O7015 (1985). |
[3] | Gamerith, G., J. Chromatogr. 256 ,326 (1983). |
[4] | Kolthoff, I.M. and Chantooni, M.K., J. Am. Chem. Soc., 87, 4428 (1965). |
[5] | Kolthoff, I.M., Chantooni, M.K. and Bhownik, S., J. Am. Chem. Soc., 90, 123 (1968). |
[6] | Aleksandrov, V.V., Zudochkina, A.I., and Sandovinchaya, L.P., Zh. Fiz. Khim., 52, 1295 (1978). |
[7] | Alekandrov, V.V., and Burakhovich, Fizicheskaya Khimiya Rastrorov (Physical Chemistry in Solutions), Nauka, Moscow, 1972, p. 154. |
[8] | Kreshkov, A.P., Tanganor, B.B., Yarovenko, A.N. and Batoreva, T. Kh., Zh. Fiz. Khim., 54, 105 (1980). |
[9] | Gomaa, E.A., Mousa, M.A. and El-Khouly, A.A., Thermochimica Acta, 89, 133-139 (1985). |
[10] | Kim, J.T., Cecal, A., Born, H.J. and Gomaa, E.A., Z. Physikalische Chemic, Neue Folge, 110, 209-227 (1978). |
[11] | El-Khouly, A.A., Gomaa, E.A. and Abou-El-Leef, S., Bull. of Electrochemistry, 19, 153-164 (2003). |
[12] | El-Khouly, A.A., Gomaa, E.A., Abou El-Leef, S., Bull. Of electrochemistry, 19, 193-202 (2003). |
[13] | Oswal, S.L., Desai, J.S., Ijardar, S.P. and Jain, D.M., journal of Mol. Liquids, 144, 108-114 (2009). |
[14] | Dorota Bobicz, Waclaw Grzybkowski and Andrzej Lwandowski, J. of Mol. Liquids, 105, 93-104 (2003). |
[15] | Marcus, Y. “The properties of solvents”, Wiley, London, 1998. |
[16] | Moelwyn-Hughes, E.A., “Physikalische Chemie”, George Thieme Verlag, Stuttgart, 1970, p. 489. |
[17] | Gomaa, E.A., Mousa, M.A. and El-Khouly, Thermochim. Acta, 89, 133-139 (1985). |
[18] | Gomaa, Esam, A., Thermochim. Acta, 120, 183-190 (1987). |
[19] | Bondi, A., J. Phys. Chem., 68 (1964) 441. |