Abdel El-Aziz A. Said 1, Aref A. M. Aly 1, Mohamed M. Abd El-Wahab 1, Soliman A. Soliman 1, Aly A.Abd El-Hafez 1, V.Helmey 2, Mohamed N.Goda 1
1Chemistry Department, Faculty of Science, Assiut University, Assiut, Egypt
2Mechanical Engineering Department, Faculty of Engineering, Assiut University, Assiut, Egypt
Correspondence to: Abdel El-Aziz A. Said , Chemistry Department, Faculty of Science, Assiut University, Assiut, Egypt.
Email: |  |
Copyright © 2012 Scientific & Academic Publishing. All Rights Reserved.
Abstract
Raw sugarcane bagasse modified with propionic acid (RBMPA) was used as an efficient, low cost and eco-friendly adsorbent for the removal of methylene blue (basic) and orange II (acid) dyes from aqueous solutions. The effect of different system variables, namely contact time, dye concentration, adsorption dose, pH and particle size were investigated and optimal experimental conditions were ascertained. A greater percentage of dye removal was observed in acidic medium (pH=2) in case of orange II whereas in (pH=7) in case of methylene blue dyes and increases with the increase of the dosage of adsorbent. Maximum dye removal was attained after 60 min. Equilibrium isotherms were analysed by the Langmuir and Freundlich models of adsorption and it was found that the Langmuir isotherm provides a good model for the adsorption of methylene blue whereas the Freundlich isotherm is fit for the adsorption of orange II. The kinetics of the adsorption of both dyes was consistent with pseudo second-order kinetics with a good correlation (R2 > 0.99). Furthermore, the amounts of dye adsorbed at equilibrium, qe (mg g-1) for methylene blue and orange II were 59.5 and 25.5, respectively.
Keywords:
Modified Bagasse, Adsorption, Methylene Blue, Orange II, Kinetics
1. Introduction
Dyes are type of organic compounds that can provide bright and lasting color to other substances1. There are more than 100.000 dyes available commercially, which are specifically designed to resist fading upon exposure to sweat, light, water, and oxidizing agents and, as such, are very stable and difficult to degrade2. Synthetic dyes have been increasingly used in the textile, leather, paper, rubber, plastic, cosmetics, pharmaceuticals, and food industries. Contamination of water sources by visible colored pollutants mainly dye staff has become a major problem for the environment. The colored wastewater discharged into environmental bodies of water is not only an esthetically unpleasant but also interferes with light penetration and reduce photosynthetic action. Many dyes or their metabolites have toxic as well as carcinogenic, mutagenic, and teratogenic effects on aquatic life and humans3. Hence, the removal of dyes from wastewater is essential to prevent continuous environmental pollution.The conventional wastewater treatment, which rely on aerobic biodegrading have low removal efficiency for reactive and other anionic soluble dyes. Due to low biodegrading of dyes, a conventional biological treatment process is not very effective in removing of dyes from wastewater. It is usually treated either by physical or chemical processes. However, these processes are very expensive and cannot effectively be used to treat the wide range of dyes waste4. The adsorption process is one of the effective methods for removal dyes from the waste effluent.Activated carbon (powder or granular) is the most widely used adsorbent because it has an excellent adsorption efficiency for organic compounds. But, commercially available activated carbon is very expensive. This had lead to further studies for cheaper substitutions. Nowadays, there are numerous numbers of low cost, commercially available adsorbents which had been used for the dye removal. A variety of agricultural biosorbents, including bagasse, have been recently investigated for their dye removal ability5-8. The chemical modification of sorbents with acids or bases was reported in the literature in order to enhance their adsorption capacity3, 9. The removal of methyl red from simulated wastewater by using modified bagasse has been investigated for different variables 10. They have shown that sugarcane bagasse treated with phosphoric acid and untreated sugarcane bagasse has a lower adsorption efficiency compared to powdered activated carbon. Congo Red adsorption by ball-milled sugarcane bagasse has been studied by Zhang et al 11. They found that the adsorption capacity of Congo Red increased significantly with small changes in bagasse surface area. Maximum capacity was 38.2 mg g-1 at a concentration of 500 mg L-1. Karla et al 12 studied the application of succinylated sugarcane bagasse as adsorbent to remove methylene blue and gentian violet from aqueous solutions. The results revealed that, the adsorption processes could be described by pseudo-second order kinetic model. Adsorption isotherms were well fitted by Langmuir equation. Moreover, the maximum capacities for methylene blue and gentian violet were found to be 478 and 1273.2 mg g-1. Sugarcane bagasse modified with tetraehylenepentamine for removal of eosin Y has been studied by Gang-Biao et al 13. They concluded that the modified bagasse exhibited interesting sorption properties towards anionic dyes to the plentiful introduced amino groups. The experimental data showed also that the sorption process was rapid and followed a pseudo-second order model and well fitted Langmuir's isotherm model. However, the use of modified sugarcane bagasse with propionic acid for removing methylene blue and orange II dyes from wastewater to our knowledge has not yet reported. Therefore, in this paper, we studied the performance of sugarcane bagasse modified with propionic acid on the removal of the basic dye methylene blue and the acid dye orange II. We also studied several factors influencing the adsorption efficiency such as pH, contact time, dye concentration, biomass dosage and particle size. The equilibrium adsorption capacity of both dyes on the modified bagasse was determined making use of the Freundlich and Langmuir isotherm equations. Furthermore, the adsorption kinetics of the two dyes was studied by application of pseudo-first and second-order models.
2. Materials and Methods
2.1. Preparation of Adsorbent
Sugarcane bagasse was purchased from the Egyptian sugar and integrated industries company (ESIIC) and were subjected to repeatedly washing with distilled water to remove dust and soluble impurities .It was dried overnight at 60oC before crouching and shredding. The dried and shredded bagasse was then stored in a glass container and designated as a raw bagasse (RB). The propionic acid modified RB was prepared by mixing 5 wt. % acid with RB at room temperature. Finally the treated RB was dried in an oven at 60℃ overnight and stored and labelled as raw sugarcane bagasse modified with propionic acid (RBMPA). A stock solution of dyes (600 and 300 mg L-1) of methylene blue and orange II respectively were prepared and stored in a dark place and used freshly. All the chemicals used throughout this study were of analytical grade reagents. The chemical structures of the two dyes are presented in Fig.1. Double distilled water was used for preparing all of the reagents solutions throughout the study. All the adsorption experiments were carried out at room temperature
using 1 gm (0.25 mm) and pH,s 7.0 and 2.0 for the adsorption of methylene blue and orange II respectively.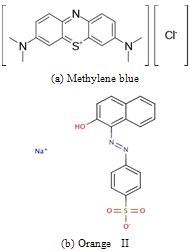 | Figure 1. The chemical structure of methylene blue (a) and orange II (b) |
2.2. Methods
All pH measurements were carried out with a pH-meter model number JENWAY 3450. The initial pH values were adjusted with 0.1 M HCl or NaOH to form a series of pH 's from 2 to 11. Concentration of dye solutions were estimated using a thermo Fischer scientific, aquamate, and evolution 166 UV-visible spectrophotometer.
2.3. Adsorption Study
The adsorption study was mainly carried out by batch technique to obtain rate and equilibrium data. The experiments were performed to observe the effect of pH, contact time, particle size, concentration, etc. One gram of adsorbent was taken in 100 mL airtight volumetric flasks containing 50 mL each of different adsorbate solutions of known concentrations. The flasks were then shaked intermittently for 1 hr to attain the equilibrium condition of adsorption at room temperature. The solutions obtained were then filtered using Whattmann filter paper and analysed spectrophotometrically by measuring the absorbance at λmax of each dye.
2.4. Kinetic Studies
To assess the applicability of the adsorption process it is necessary to determine the kinetic parameters, using the batch technique. A series of volumetric flasks containing 100 mL capacity, containing 50 mL dye solutions of known concentrations at the appropriate pH and agaited by mechanical shaker at room temperature. After a definite time interval, the solutions were filtered and the filtrates thus obtained were then analyzed spectrophotometrically. All the experiments were duplicated and only the mean values are reported. The maximum deviation observed was less than ± 5%.
3. Results and Discussion
3.1. Effect of Contact Time
The contact time between pollutant and the adsorbent is of significant importance in the wastewater treatment by adsorption. A rapid uptake of pollutants and establishment of equilibrium in short period signifies the efficiency of that adsorbate for its use in wastewater treatment. Available adsorption studied in literature reveal that the uptake of the adsorbate species is fast at the initial stages of the contact period, and therefore, it becomes slower near the equilibrium. The effect of contact time for the adsorption of methylene blue and orange II was studied for a period of 240 min and the results are shown in Fig.(2). It shows that the dye removal was rapid in the first 10 min and the curves of contact time are single, smooth and continuous leading to saturation. These curves indicate the possible mono-layer coverage of dye on the surface of modified bagasse14-16. In addition the adsorption of methylene blue is faster than that of orange II and the equilibrium was attained after 60 min. The percentage removal of methylene blue dye after 2 min was found equal to 84% relative to 62% for orange II dye. Furthermore, these results reveal that the available sites on the RBMPA surface are more active towards adsorption of methylene blue than the adsorption of orange II.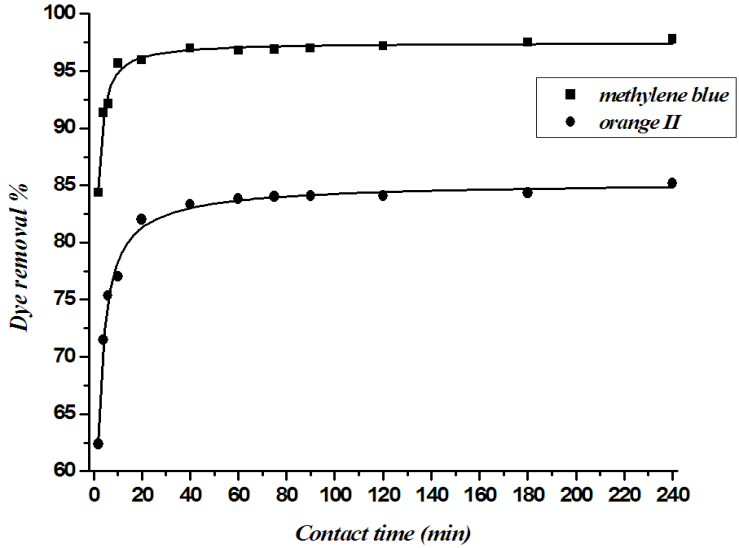 | Figure 2. Variation of dye removal with contact time for the adsorption of methylene blue and orange II onto RBMPA |
3.2. Effect of Initial Dye Concentration
The effect of initial methylene blue and orange II concentrations for their removal by RBMPA is shown in Fig (3&4), from these figures it is evident that as the initial dye concentration increased, the adsorption capacity increased which exhibited a strong linear relationship for both dyes with the correlation coefficient (R2) close to unity. However, the adsorption capacity of RBMPA to methylene blue reached to 100 % when the dye concentration increased from 25 up to 450 mg L-1. On the other hand, the adsorption capacity of RBMPA to the orange II attained 100 % when the dye concentration increased from 25 up to 100 mg L-1. These results revealed that the available active sites on RBMPA surface have greater tendency to the adsorption of methylene blue than that of orange II. These results may indicate that there is a possibility to treat textile efficient with relatively higher dye concentration using low cost RBMPA.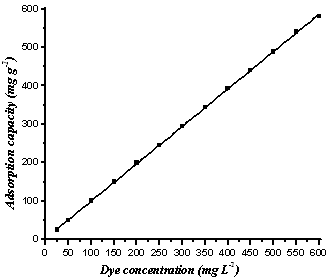 | Figure 3. Effect of methylene blue concentration with the adsorption capacity by RBMPA at pH=3.0 |
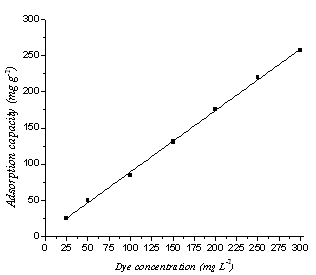 | Figure 4. Variation of orange II concentration with the adsorption capacity by RBMPA at pH=2.0 |
3.3. Effect of pH
The pH of the solution affects the surface charge of the adsorbents as well as the degree of ionization of different pollutants. Change of pH also affects the adsorptive process through dissociation of functional groups on the adsorbent surface active sites. Consequently, this leads to a shift in reaction kinetics and adsorption equilibrium. The adsorption of methylene blue and orange II over a pH range of 2-11 were carried. The results presented in Fig. 5 indicate that the % dye removal for methylene blue was 90 % and increased with the increase of pH up to 3.0 keeping its value (97%) on increasing the pH value up to 11. On the other hand the maximum % dye removal (80 %) for orange II was found at pH=2 and followed by a contentious decrease on increasing the pH up to 4 with value of 38 %. Further increase in the pH value is accompanied with no change in the dye removal. The high percentage removal may be attributed to a significantly high electrostatic attraction exists between the positively charged surface of the adsorbent and the anionic dye17. It is interest to mention here that as the pH of the system increased above 3 the dye removal of methylene blue is kept constant indicating the number of the positively charged sites is unchanged. On the other hand, on increasing the pH above 2 a negatively charged surface sites on the adsorbent did not favour the adsorption of orange II dye anions due to electrostatic repulsion. In conclusion the removal of methylene blue is unaffected by increasing the pH value, whereas the removal of orange II is drastically affected by increasing the pH value.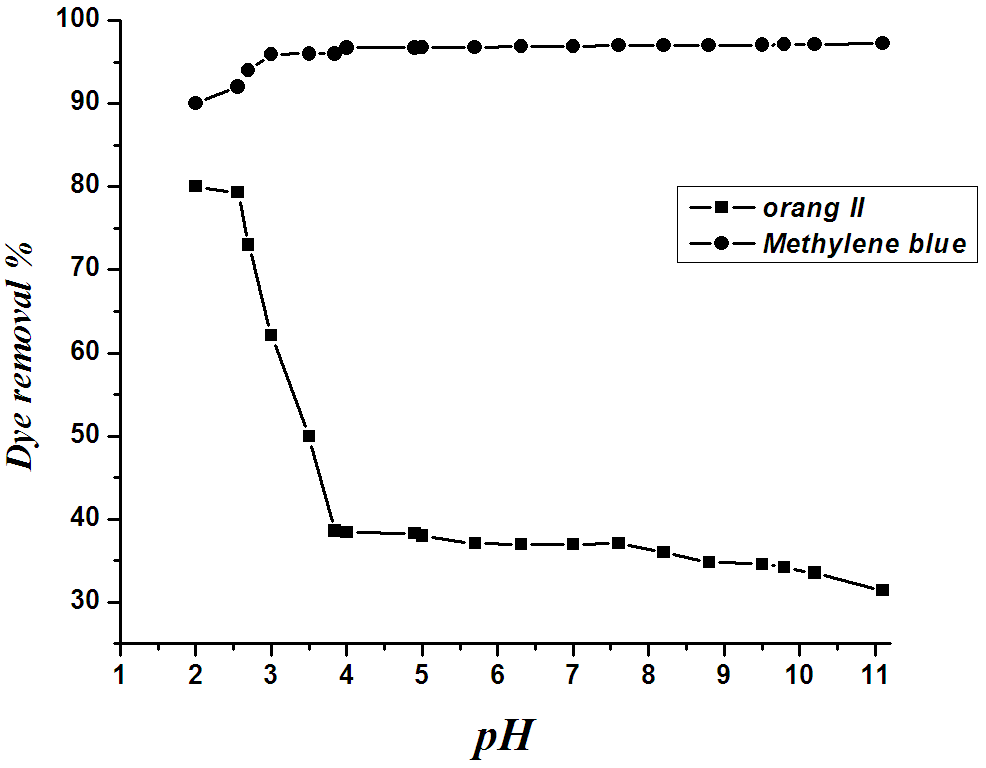 | Figure 5. Effect of pH on adsorption of methylene blue and orange II onto BMPA |
3.4. Effect of Adsorbent Dosage
The effect of RBMPA dosage on the removal of methylene blue and orange II are shown in Fig. (6). the percentage removal increased with the RBMPA dosage up to a certain limit then it reached a constant value. The increase in adsorption of dyes with adsorbent dosage was due to the availability of more adsorption sites on bagasse surface for adsorption. The results clear that remarkable removal of the methylene blue dye in comparison with the % removal of orange II in presence of 0.2 g adsorbent. This behaviour indicates that the number of available sites on the RBMPA surface is active towards the adsorption of methylene blue.
3.5. Effect of Particle Size
The particle size has a significant influence on the kinetic of adsorption. The influence of particle size furnishes important information for achieving optimum utilization of adsorbent. Rate of adsorption on solid surface is expected to vary with available surface area for a constant adsorbent weight with the particle size.The adsorption capacity is directly proportional to the total exposed surface and inversely to the particle diameter for non-porous adsorbents. The presence of a large number of smaller particles provides the adsorption system with a greater surface area available for dye removal 18. Fig. 7 shows the results of removal of methylene blue and orange II dyes on the different particle sizes (0.25-1.0 mm). It was observed that as the particle size decreased the amount of adsorbed dye increased. This is due to the large external surface area available from fine particles19. Furthermore, the variation of methylene blue removal is higher than that of orange II and quite constant with the different particle size. This behaviour means that the available active sites on RBMPA are little changed with the different particle size.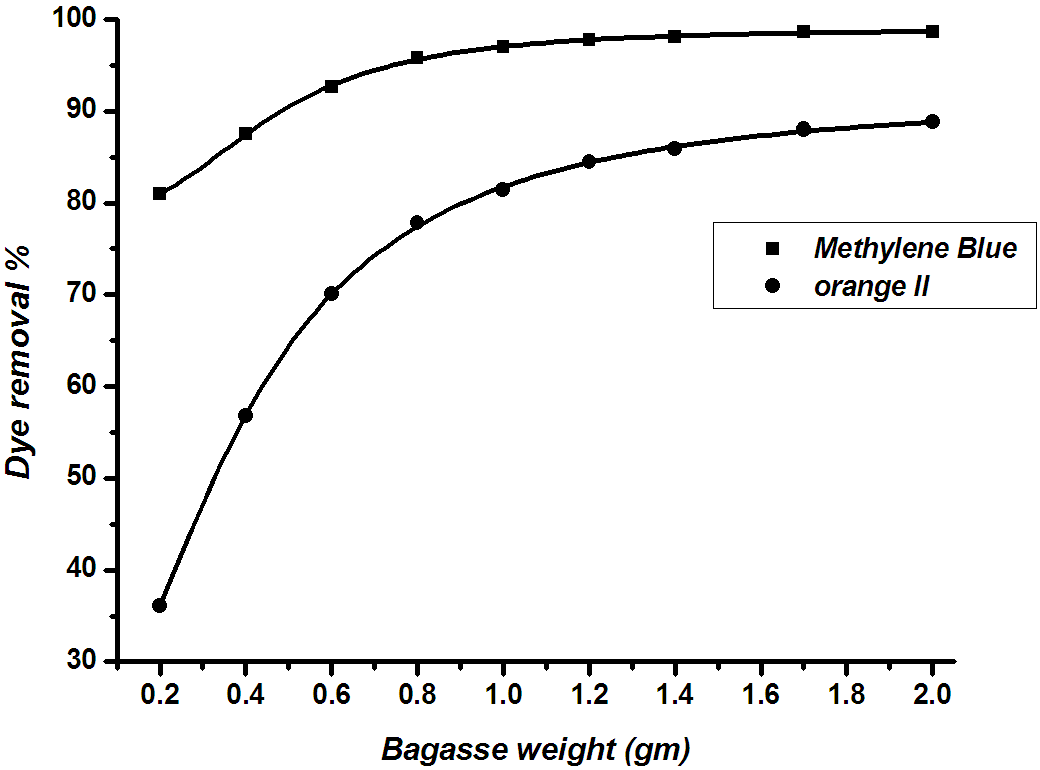 | Figure 6. Effect of RBMPA dosage on the adsorption of methylene blue and orange II |
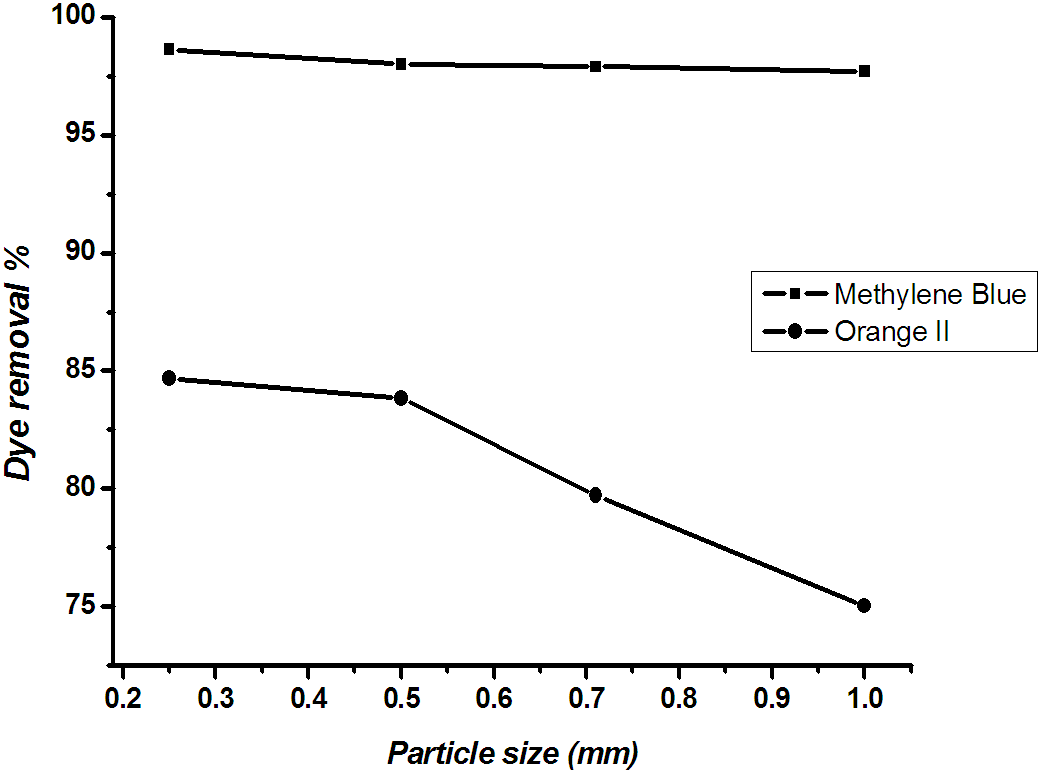 | Figure 7. Effect of particle size of RBMPA on the adsorption of methylene blue and orange II |
3.6. Adsorption Equilibrium Study
Equilibrium isotherm equations are used to describe the experimental adsorption data. The parameters obtained from the different models provide important information on the adsorption mechanisms and surface properties and affinities of the adsorbent. The most accepted surface adsorption models for single-state systems are the Langmuir and Freundlich models.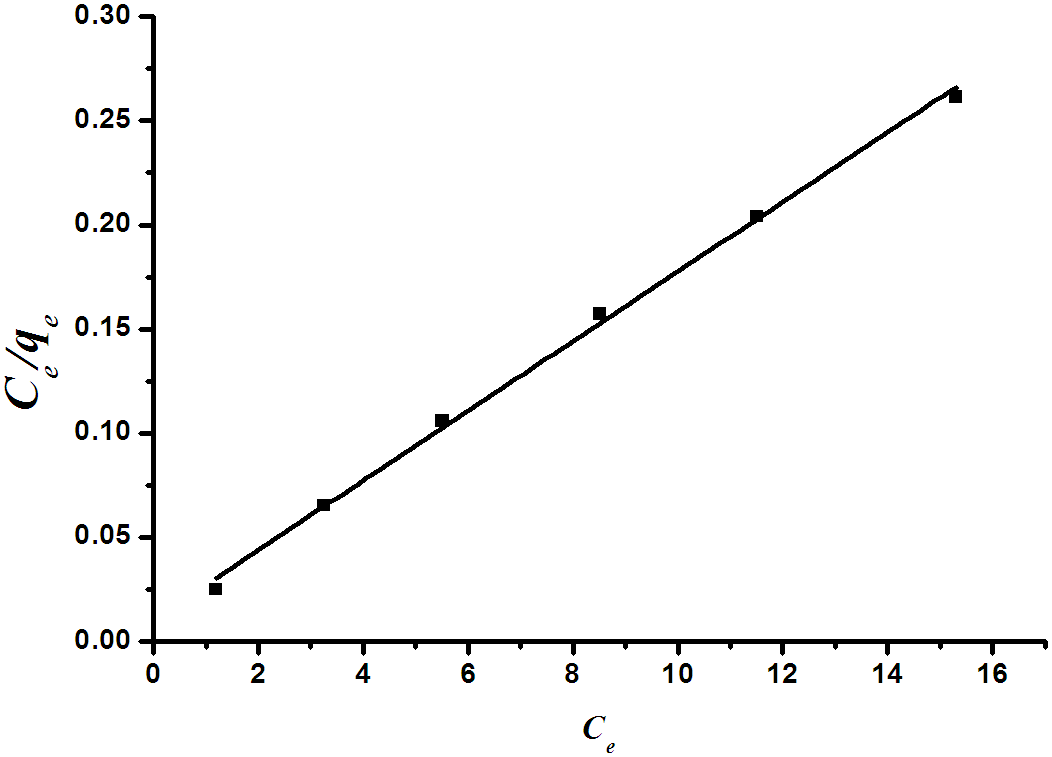 | Figure 8a. Langmiur plot for the removal of methylene blue dye by RBMPA |
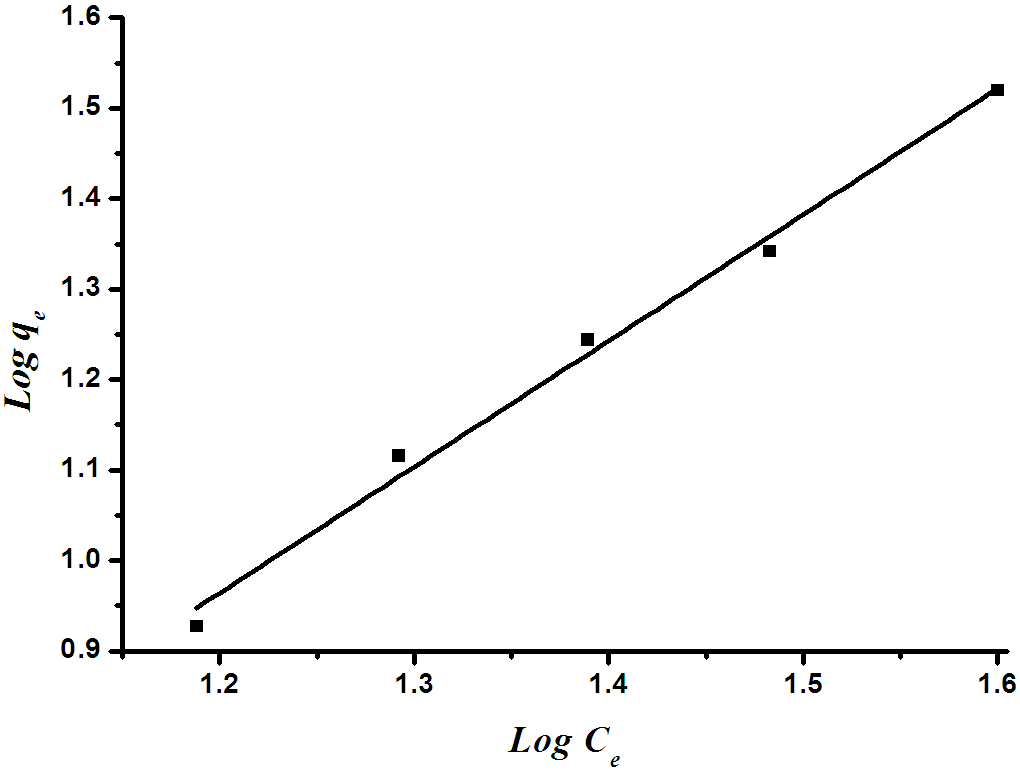 | Figure 8b. Freundlich isotherm plot for the removal of orange II dye by RBMPA |
Langmuir isothermA basic assumption of the Langmuir theory is that adsorption takes place at specific homogenous sites within the adsorbent. It is then assumed that once a dye molecule occupies a site, no further adsorption can take place at that site. Theoretically, therefore, a saturation value is reached, i.e., monolayer adsorption. The Langmuir isotherm is represented by the following equation: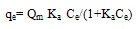 | (1) |
where qe (mg g-1) is the amount of dye adsorbed at equilibrium, Ce (mg L-1) is the equilibrium concentration of the dye, Qm is a constant and reflects a complete monolayer (mg g-1) and Ka is the adsorption equilibrium constant (Lmg-1) that is related to the apparent of adsorption.The Langmuir isotherm ( Eq.1) can be linearised into the following form: | (2) |
The Qm and Ka values can be obtained from the slope (1/Qm) and intercept (1/KaQm) of the linear plot of Ce/qe versus Ce.Freundlich isothermThe Frundlich isotherm is derived by assuming a heterogeneous surface with a non-uniform distribution of heat of adsorption over the surface. The Freundlich isotherm is represented by the following equation: | (3) |
Where qe and Ce are defined as before in eq. (1) and KF is a constant related to bonding energy and adsorption capacity. 1/n indicates the adsorption intensity of dye onto the adsorbent and the type of isotherm to be irreversible (1/n=0), favourable (0<1/n<1) and unfavourable (1/n >1).This equation can be linearized as follow: | (4) |
Fig.8 (a&b) illustrates the Langmiur and Freundlich linear isotherm for methylene blue and orange II. The coefficients isotherm parameters for both dyes on RBMPA are shown in Table (1). The correlation coefficients reported in Table (1) show strong positive evidence on the adsorption of the two dyes on modified bagasse. The applicability of the linear form of Langmuir and Freundlich models to modified bagasse was proved by the high correlation coefficients R2>0.99. This suggests that the Langmuir isotherm provides a good model for the adsorption of methylene blue whereas the Frundlich isotherm is a suitable model for the adsorption of orange II.Table 1. Langmuir and Frundlich parameters for the removal of methylene blue and orange II dyes by RBMPA |
| dye | Frundlich | R2 | KF | n | Methylene blue | - | - | - | Orange II | 0.9902 | 0.1959 | 0.7176 | dye | Langmuir | R2 | Ka(Lmg g-1) | Qm (mgg-1) | Methylene blue | 0.9974 | 1.5755 | 59.8802 | Orange II | - | - | - |
|
|
3.7. Adsorption Kinetic Study
In order to investigate the adsorption process of methylene blue and orange II dyes on RBMPA pseudo-first-order and pseudo-second-order were used.Pseudo-first-order and pseudo-second-order rate equations are expressed as follows: | (5) |
 | (6) |
where qe and qt are the amount of adsorbed dyes (mg g-1) at equilibrium and at time t, respectively,K1 is the rate constant of pseudo-first-order adsorption (min-1), h (K2 qe2) is the initial adsorption rate (mg g-1min-1) and K2 is the rate constant of pseudo-second-order adsorption (mg g-1 min-1). The straight lines obtained and presented in Fig. 9 indicate the applicability of the above equations. The values of K1 and K2 were calculated from the slopes of the respective linear plots and are noted in Table (2). Comparing the correlation coefficients of both equations it was to be concluded that the kinetic model for methylene blue and orange II is consistent with a pseudo-second-order (R2 >0.99).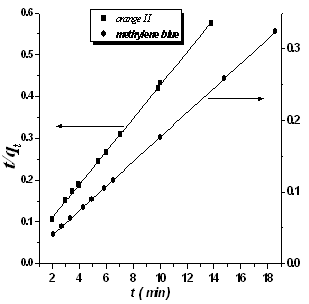 | Figure 9. The pseudo-second-order plot for methylene blue and orange II dyes |
Table 2. the pseudo-second-order parameters for methylene blue and orang II dyes |
| Dye | R2 | K2 (mg g-1min-1) | Methylene blue | 0.9999 | 0.0548 | Orange II | 0.9995 | 0.0501 |
|
|
3.8. Intra-particle Diffusion Study
Weber-Morris20 found an empirical function relationship common to most adsorption processes in which the uptake varies almost proportionally with t1/2 as the following equation: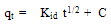 | (7) |
Where Kid is the intra-particle diffusion rate constant, qt (mg g-1) is the amount of dye adsorbed at time t (min). A plot of qt versus t1/2 should be a straight line with a slope Kid and intercept C. Values of intercept give an idea about the thickness of the boundary layer, i.e., the larger the intercept the greater the boundary layer effect 21. Fig. 10 shows plots of mass of dyes adsorbed qt versus t1/2 for methylene blue and orange II. The results indicate that the adsorption process of both dyes exhibits two separate regions with two straight lines. The values of intercept, slope and the correlation coefficient (R2) were calculated and presented in Table 3.The deviation of the straight lines from the origin (Fig.10) may be due to the difference in the rate of mass transfer in the initial and final stages of adsorption 22. Further, the first straight portion is attributed to a macropores diffusion process and the second linear portion can be ascribed to a micropore diffusion process 23. In addition, it is clear from Fig.10 that the first stage is faster than the second one. This behaviour may be correlated with the very slow diffusion of the adsorbate from the surface film into the micropores, which are the least accessible sites for adsorption. Furthermore, the values of R2 clearly prove the good applicability of the Weber-Morris equation20. The parameters of the intra-particle diffusion data for methylene blue and orange II are listed in Table 3. Table 3. Intra-particle diffusion model parameters for methylene blue and orange II dyes |
| dye | Stage I | R2 | Slope | Intercept | Methylene blue | 0.9915 | 5.9335 | 42.0435 | Orange II | 0.9833 | 3.5484 | 13.6684 | dye | Stage II | R2 | Slope | Methylene blue | 0.9687 | 0.7354 | Orange II | 0.9499 | 0.4486 |
|
|
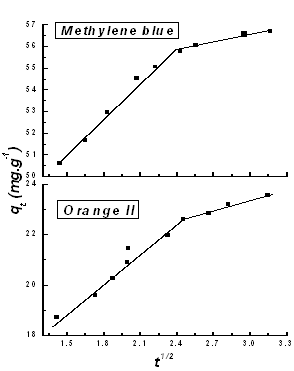 | Figure 10. Intra-particle diffusion model for methylene blue and orange II dyes |
4. Conclusions
The present study shows that sugarcane bagasse modified with propionic acid (RBMPA) is an effective adsorbent for the removal of methylene blue and orange II dyes from aqueous solutions. The effective pH for metylene blue and orange II were 7 and 2.0 respectively. Quasi-equilibrium was practically achieved in 60 min. The adsorption equilibrium data of the two dyes indicated that the adsorption of methylene blue well fitted the Langmuir equation whereas the Freundlich equation is an applicable model for the adsorption of orange II. Adsorption kinetics revealed that methylene blue and orange II follow a pseudo-second-order model. The intra-particle diffusion results pointed to the presence of macropores in the first stage and micropores as a second slow stage of the adsorption process. The present study concluded that the RBMPA could be employed as a low cost and ecofriendly adsorbent as an alternative to commercial activated carbon for the removal of dyes from wastewater.
ACKNOWLEDGEMENTS
The authors would like to thank the financial supports from the Science Technology Development Fund, STDF, Ministry of Higher Education, Egypt.
References
[1] | J R.Gong, Y.Sun, J.Chen, H.Liu and C.Yang,. Effect of chemical modification on dye adsorption capacity of peanut hull. Dyes Pigments, 67 (2005) pp. 175-181. |
[2] | P.Nigam, G.Armour, I.M. Banat, D.Singh and R.Marchant,. Physical removal of textile dyes from effluents and solid-state fermentation of dye-adsorbed agricultural residues. Bioresource technol., 72 (2000) pp. 219-226. |
[3] | R.Gong, Y. Jin, J.Chen, Y.Hu and j. Sun,. Removal of basic dyes from aqueous solution by sorption on phosphoric acid modified rice straw. Dyes pigments, 73 (2007) pp. 332-733. |
[4] | V.K.Grag, R.Gupta, A.Bala and R.Kumar,. Dye removal from aqueous solution by adsorption on treated sawdust. Bioresource Technol., 89 (2003) pp. 121-124. |
[5] | C.Nomasivayam and N.Kanchana. Removal of congo red form aqueous solution by waste banana pith. J.Sci.Technol. 1 (1993) pp. 33-42. |
[6] | V.Vadivelan and K.V.kumar. Equilibrium, Kinetics, mechanism and process design for the sorption of methylene blue onto rice husk. Colloid. Interf. Sci. (2005) pp. 90-100. |
[7] | L.S.Oliveira, A.S. Franca, T.M.Alves and S.D. F. Rocha. Evaluation of untreated coffee husks as potential biosorbents for treatment of due contaminated water. J.Hazard. Mater. 155 (2008) pp. 507-512. |
[8] | V.K.Gupta and suhas, “Application of low-cost adsorbents for dye removal – A review,” J.Environ. Manag., vol. 90 June (2009) pp.2313-2342. |
[9] | A. A. Said, A. G. Ludwick and H. A. Aglan, Usefulness of raw bagasse for oil absorption : A comparison of raw and acylated bagasse and their components. Bioresource Technol. 100 (2009) pp. 2219-2222. |
[10] | S.A. Saad, K.Md. Isa, R. Bahari Chemically modified sugarcane bagasse as a potentially low-cost biosorbent for dye removal, Desalination 264 (2010) pp. 123–128 |
[11] | Z. Zhang, L. Moghaddam, Ian M. O’Hara, W. O.S. Doherty, Congo Red adsorption by ball-milled sugarcane bagasse, Chem. Eng. J. 178 (2011) pp. 122– 128. |
[12] | K. A. G. Gusmãoa, L. V. A. Gurgel, T. M. S. Meloa and L. F. Gil, Application of succinylated sugarcane bagasse as adsorbent to remove methylene blue and gentian violet from aqueous solutions e Kinetic and equilibrium studies. Dyes and Pigments 92 (2012) pp. 967-974. |
[13] | J. Gang-Biao, T. L-Zuan, H. Xiao-Yi, Z. Yu-Qiang, R. Cui-Cui, H. Chen-Ke, H. Zhi-Jian, Potential biosorbent based on sugarcane bagasse modified with tetraethylenepentamine for removal of eosin Y. Int. J. Biol. Macro. 50 (2012) pp. 707– 712. |
[14] | Y.wong and j.yu, lactase catalyzed decolonization of synthetic dyes, water res 33 (16) (1999) pp. 3512-3520. |
[15] | P.K Malik, use of activated carbons prepared from sawa dust and rice-husk for adsorption of acid dyes, dyes and pigments 56(2003) pp. 239-249. |
[16] | B.S.Inbraj and n.sulochana, basic due to adsorption on a low cost carbonaceous sorbent-kinetic and equilibrium studies, Indian J.Technol 9 (2000) pp. 201-208. |
[17] | I.D.Mall, V.C.srivastava and N.K.Aqarwal, Dyes and pigments, 69(2006) pp. 210-223 |
[18] | A.G. Liew Abdullah, M.A.Mohdsaleh, M.K.siti Mazlina , M.J.Megate Mohd Noor , M.R.Osman, R.wagiran,S.Sabri, Azo dye removal by adsorption using waste biomass sugar cane bagasse, Int.J. ENG.Technol 2(1) (2005) pp. 8-13 |
[19] | S. D. Khattri, M.K.Singh, Color removal from aqueous solution by adsorption, Indian J.chem.Technol 5(4) (1998) pp. 230-234. |
[20] | W. J. Weber Jr and J. C. Morris, kinetics of adsorption on carbon from solution, Jessant Engg. Div ASCE 89(1963) (SA2), pp. 31-59. |
[21] | K.Kannan and M.M.Sundaram , kinetics and mechanism of removal of methylene blue by adsorption on various carbons A comparatiestudy, Dyes and Pigments 51(2001) PP. 25-40. |
[22] | K.K.Panday, G.Prasad and V.N.Singh , Mixed adsorbent for Cu(II) removal from aqueous solutions, Envron. Technol. lett.50 (7 ) (1986) PP. 547-554 |
[23] | B.S.Inbraj and N.Sulochana, Basic due to adsorption on a low cost carbonaceous sorbent-kinetic and equilibrium studies, Indian J.Technol 9 (2002) pp. 201-208. |