K. Jovaag , J. Dekker , B. Atchison
Weed Biology Laboratory, Department of Agronomy, Iowa State University, Ames, Iowa, 50011, USA
Correspondence to: J. Dekker , .
Email: |  |
Copyright © 2012 Scientific & Academic Publishing. All Rights Reserved.
Abstract
The fate of heteroblastic Setaria faberi seed entering the soil post-abscission is elucidated. Introduction of four populations of S. faberi seeds with heterogeneous dormancy capacities into the soil of a no-till Glycine max field resulted in the formation of enduring pools with varying cycles of dormancy, after-ripening, germination, dormancy reinduction and death. The buried seed rain of these highly dormant seed after-ripened with time and became highly germinable, awaiting favourable temperature and moisture conditions: the heterogeneous germination candidate pool. As this pool was depleted in the spring and early summer by seedling emergence and death, dormancy was re-induced in the living seeds that remained in the soil. These seeds remained dormant throughout the summer, then resumed after-ripening during late autumn. This dormancy-germinability cycle exhibited complexity both within and among S. faberi populations. Seed heteroblasty within S. faberi populations was retained, and germinability responses to the yearly seasonal environment varied among S. faberi populations. Further, local adaptation was shown by the differential germinability responses among S. faberi populations in common location agricultural nurseries. Seed mortality patterns also exhibited complexity within and among populations. Within an individual S. faberi population, mortality patterns changed as seeds aged in the soil. Among S. faberi populations differential mortality responses were observed in response to yearly seasonal environments and common nurseries. Observations of both germinability cycling and mortality are consistent with the hypothesis that S. faberi seed behaviour in the soil is predicated on dormancy capacity heterogeneity at abscission and modulated by the seasonal environmental conditions experienced in the field. The observations of seed fates obtained from heteroblastic seeds of four S. faberi populations buried at two common nurseries utilized a “bare core” technique. Cores were extracted periodically to determine seed fates. Inevitably, the fates of a fraction of those seeds could not be determined and were thus classified as unknown. Despite the equivocal nature of the unknowns they provided evidence that unaccounted seed losses were most likely not due to migration out of the core area. The lack of migration and high seed recovery (approximately 88.5%) emphasized the utility of the bare core technique in comparison to enclosed seed-soil cores.
Keywords:
Seed Heteroblasty, Seed Dormancy, Setaria, Soil Seed Pool
Cite this paper:
K. Jovaag , J. Dekker , B. Atchison , "Setaria Faberi Seed Heteroblasty Blueprints Seedling Recruitment: II. Seed Behavior in the Soil", International Journal of Plant Research, Vol. 1 No. 1, 2011, pp. 1-10. doi: 10.5923/j.plant.20110101.01.
1. Introduction
The fate of Setaria faberi seed entering the soil subsequent to abscission is elucidated. Introduction of S. faberi into the soil can result in the formation of long-lived seed pools (e.g., Burnside, et al., 1981). With time the dormancy states, or germinability, of these seeds can change but no systematic observations of the fates of these seeds in agricultural field soils has been reported. Herein are reported the behaviour of seeds from four populations of S. faberi (Jovaag, Dekker & Atchison, 2009a) with heterogeneous dormancy capacities (heteroblasty) that were buried in a no-till Glycine max (soybeans) field without enclosures and their fates determined by periodic excavations of subsample soil cores.What occurs to heterogeneous S. faberi seeds after abscission from the parent plant when they are dispersed into field soils? One hypothesis is that environmental signals in the soil (after-ripening) during the winter (post-abscission) will overcome any differences in dormancy capacity (quantity of environmental signals required to stimulate a change in state) and thus by spring all seeds converge on a single, or much narrowed range of highly germinable seeds. These seeds will remain in this highly germinable state for days to years until they germinate and are recruited as seedlings (or they die). In either case, heteroblastic differences are lost and subsequent seed behaviours by the population are relatively homogeneous in response to local soil microsite conditions. If this occurs, it raises the question of why heteroblasty is induced during embryogenesis only to lose dormancy heteroblasty once the seed enters the soil. An alternative hypothesis is that the germinability states of individual seeds will change with soil conditions over time, but the overall heterogeneity of the population will remain. If this is the case, the dormancy capacity of an individual at abscission is an inherent quality of the seed that retains throughout its life. Its immediate germinability state will change as a function of the amount of soil signals required by its inherent dormancy capacity (Dekker et al., 2003).Experimental observation of the several behavioural processes (e.g., after-ripening, dormancy re-induction, germination, growth) of an individual seed in field soils over time, as well as mortality, cannot be directly observed. Seed states (e.g., live, dormant, fully-after-ripened, germinated, emerged, dead), however, can be observed. Therefore, to test these hypotheses of S. faberi fates, we observed changes in the relative frequencies of the different seed states over time in replicated, unenclosed, soil cores. The processes leading to changes in state were then inferred from the observed changes in these frequencies. This is the second in a series of three papers on the dynamics of S. faberi soil seed pools. The first paper demonstrated heteroblasty in 39 locally adapted populations (of which four are reported herein). The third paper examines seedling emergence and its relationship to this heteroblasty.
2. Materials and Methods
2.1. Experimental
2.1.1. Setaria Seed Populations
Setaria faberi seed was gathered from four populations, two locations in 1997 (Curtiss Farm, Oakwood Drive) and two locations in 1998 (Curtiss Farm, Johnson Farm) (Table 1). Seed was gathered in the field from S. faberi monocultures, ensuring no contamination from non-target species occurred. Seed was collected in September, Julian weeks (JW) 36 and 37. Our Julian week calendar assumes 29 days for every February. At collection time, S. faberi synflorescences were tapped against the inside wall of an empty plastic dishpan to dislodge the ripe, recently abscised (e.g., 0-5 days; Dekker et al., 1996) seed.
2.1.2. Seed Preparation
Seed populations were dried separately in #16 (1.18mm mesh openings) and/or #18 (1.00mm mesh openings) soil separation sieves (Seedburo Equipment Co., Chicago, IL, 60607) to provide airflow around the seed. Seed lots were dried at room temperature (20° C) and ambient humidity for three to four days, stirred once per day. Once dry, hard, dark, mature seeds were separated from other plant material using a seed separation blower (Seedburo Equipment Co.). Six hundred seeds from each lot were counted manually (200 seeds per envelope) to provide three replications per treatment. A sub-sample of the envelopes were recounted to ensure accuracy.
2.1.3. Seed Burial
Seeds were buried in two common location, agricultural nurseries. Both nurseries were on the Iowa State University Agricultural Experiment Research Station, at Johnson Farm, Ames, and the Southeast Research Station near Crawfordsville, Iowa (Table 1).Field soil seedbank site preparation. The areas selected for this study had been previously maintained as turf grass (primarily bromegrass, Bromus sp.) for more than 12 years. Well in advance of burial, these areas were treated with a 3% solution of glyphosate (isopropylamine salt of N-(phosphomethyl) glycine; Roundup) to kill the grass sod. After allowing time for the herbicide to work, the dead sod was tilled to a depth of about 20 cm using a rototiller. Time was allowed for continued decay of the sod and the area was tilled again. Finally, the soil was raked smooth and even for seedbed preparation. Soil cores were removed periodically in the experimental areas to confirm that no previous, contaminating S. faberi seeds were present. Crop (soybean) rows were planted 96 cm apart. Individual S. faberi seed burial sites (soil cores) were located in the inter-row area. Burial sites were arranged in a completely randomized design using three replications. Burial procedure. At each burial site, three equidistant metal-staffed marker flags were used to define an 11cm diameter round “bare core” area. The core area was unbounded to ensure continuity with the adjacent soil matrix without the experimental artefacts confining structures introduce. A 7cm diameter, 10cm deep (volume: 390cm³), soil core was extracted from the centre of the three flags. The soil was placed in a dishpan and thoroughly mixed with the 200 seeds from an envelope (seed density: 0.5 seeds/cm³). The soil was then carefully returned to the hole from which it was extracted. Seed burial occurred one to three weeks after the seed was harvested (Table 1). Table 1. Location (name, latitude, longitude), nomenclature (lot no., population name) and burial date (at Johnson anCrawfordsville nurseries) of S. faberi populations. Population names consist of 3 parts: a prefix letter designating the location, 2 digits designating the collection year, and the Julian week of abscission and collection. All locations were in Ames, IA. 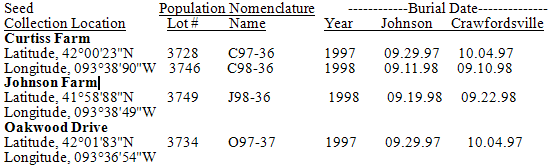 |
| |
|
Field management. No-till Roundup Ready soybeans were planted each spring between the soil core rows, simulating normal stand density and row spacing and ensuring the seed-soil cores were undisturbed. Both chemical and manual weed control methods were used to keep the plots weed free. Soybeans were harvested by hand and threshed, then the residue was redistributed over the entire plot area.
2.1.4. Seed and Seedling Recovery
Each week during the growing season, emerged seedlings in each core were counted, then severed at the soil surface and killed to prevent them from influencing subsequent germination and emergence. Soil Core Extraction. The volume of the extracted seed-soil cores (1140cm³; 11cm dia., 12cm deep) was larger than that of the buried cores to ensure all buried seed were extracted. Three seed-soil cores (three replicates) per individual population were extracted at each sampling time. Core extraction for the burial year included O97-37 from Johnson farm at 2 and 22 days after burial (1997), and C98-36 and J98-36 from both nurseries 25-45 days after burial (1998). Core extraction for the first year after burial occurred about once a month from each population and nursery (Julian Weeks 17-46). Core extraction of O97-37 for the second year after burial occurred about once a month (1999 season). Seed and seedling extraction. Seedlings and debris were removed from the extracted soil cores. The remainder of the soil was placed on screens to dry overnight. The next day the dry soil was washed through a #6 (top, 3.35mm mesh openings) and #16 (bottom, 1.18mm mesh openings) screen set. The material caught on the #16 screen was placed in Petri dishes with moistened filter paper, then examined under a microscope to separate intact (entire seeds with closed germination lids) S. faberi seeds, and seed pieces, from the remaining soil and debris.
2.1.5. Seed Fate Assays
Procedurally, the fates of intact seeds were determined using a series of three assays. Forceps assay. Whole, intact seeds were first subjected to a forceps assay (Forcella, et al., 2003). Seeds are probed with a fine tip forceps to determine if they were firm or soft.Seed germination assay. Firm intact seeds were then subjected to a germination assay. Seeds were placed in 60x15mm glass culture Petri dishes (Fisher Scientific Company, Pittsburgh, PA, 15275), 20 per dish, with two 51cm disks of Anchor Blue germination blotter paper (Anchor Paper Co., St. Paul, MN, 55101) and 2ml (1998) or 3ml (1999) of distilled, de-ionized water. The dishes were sealed with two layers of Parafilm “M” (American National Can, Chicago, IL, 60631) to prevent water loss. The dishes were then placed in a controlled environment seed germination cabinet (Model SG-30, Hoffman Manufacturing, Inc., Albany, OR, 97321) for eight days. Daily conditions in the germination chamber alternated between 16 hours of light at 30℃ followed by 8 hours of darkness at 20℃. After eight days seed in each dish was evaluated for germination. A seed was considered germinated if the coleorhiza and/or coleoptile protruded outside the seed hull (Dekker, et al., 1996).Tetrazolium seed viability assay. Firm intact seeds which did not germinate during the germination assay were subjected to a tetrazolium (TZ) viability assay (Cottrell, 1947; Flemion and Poole, 1948). They were nearly bisected longitudinally with a scalpel, the halves flattened, and placed in small Petri dishes with enough 0.1% solution of distilled, de-ionized water and 2,3,5-triphenyl-2H-tetrazolium chloride to cover the seeds. The dishes were sealed with two layers of Parafilm “M” to prevent water loss, and one layer of aluminium foil to exclude light. The dishes were then placed in a 24℃ germination cabinet for a minimum of 24 hours, and then examined to determine if they were viable (red or pink staining of the embryo) or not viable (unstained).
2.1.6. Seed and Seedling Fates
Ultimately, any seed in the soil seed bank will either germinate and become a seedling or die. However, at the time of seed-soil core extraction, a buried seed could be in one of six observable states (fates): fully after-ripened seed, dormant seed, dead intact seed, emerged seedling, dead seedling, or unknown. Individual seed fates were determined by the following criteria: Fully after-ripened seed: Seeds that germinated during the 8 day germination assay. Dormant seed: Seeds that did not germinate, but were viable (TZ assay).Dead intact seed: Seeds that failed the forceps assay, and those that were not viable (TZ assay). Emerged seedling: Intact seedlings with green shoot tissue evident.Dead seedling (or “fatal germination”): Intact seedlings that were obviously dead or dying (brown or necrotic shoots and/or roots).Unknown: The remainder of the seeds and seedlings. The unknown group consisted of non-recovered seed, and recovered seeds and seedlings whose fate was ambiguous. Non-recovered seed. Non-recovered seed could have been a consequence of predation, rotting, movement out of the core, or loss during the extraction process.Ambiguous fates. Near-entire seeds with open or missing germination lids, and seed pieces, were classified as dead rotted seed, but they could also have been the remains of germinated seed. Intact seedlings with white or yellow shoot tissue were classified as healthy unemerged seedlings, but they could have either emerged or died in the soil had they not been extracted. Broken seedlings could have been the remains of severed, emerged seedlings or dead seedlings.
2.2. Analysis
The goal for this paper was to determine how the relative frequencies of seed fates change over time in the soil. Thus the analysis focused primarily on the first year after burial, for which there was monthly data from each population and nursery. Separate analyses were conducted for the burial year, which had samples from only one or two times, and the second year after burial, which had data for a single population and nursery (O97-37 at Johnson).
2.2.1. Fully After-ripened and Dormant Seed
The proportions of recovered, fully after-ripened live seed during the first year after burial (1998 for 1997 populations, 1999 for 1998 populations) and the second year after burial (for O97-37) were modelled using three parameter Lorentzian functions where appropriate. Spring (JW16-31) and Autumn (JW32-46) periods were modelled separately. This model was chosen by first fitting numerous linear and nonlinear models to the data using the program Tablecurve (SYSTAT, Inc.), then examining models with the best fits based on least squares. The three parameter Lorentzian function fit well for both spring and autumn data. Letting Yj represent the proportion of fully after-ripened seed in core j, and xj represent the seasonal time (JW) at which the core was extracted, a regression using a three parameter Lorentzian response curve is, for j=1,…n:  | (1) |
where
are independent error terms. This is a transition type model, appropriate for data bounded by 0 and 1, and its parameters were readily interpretable: β0=height of the function, interpreted as the maximum proportion of fully after-ripened seedsβ1=transition centre, interpreted as the time (JW) at which the proportion of fully-after-ripened seeds is ½ the maximum (parm β0)β2=½ the transition width, interpreted as ½ the time (in JWs) required for a linear decrease from 75% to 25% of the maximum (parm β0) in the spring; or linear increase from 25% to 75% in the autumn.Estimates were obtained using proc nlin in SAS (SAS Institute, Inc.). Plots of the cube root squared studentized residuals (Carroll & Rupert, 1988) against the log of the predicted values indicated that the variance increased with predicted value in some instances. Thus, variances were modelled using a power of the mean model. The model then becomes: | (2) |
where
is the Lorentzian response function as in (1) and
as previously. Numerous comparisons among years, nurseries, and populations were desired. This was conservatively done by comparing the Wald based approximate 95% confidence intervals of the parameters.
2.2.2. Emerged Seedlings
Emergence is presented herein as part of the overall seed recovery. However, a complete presentation of seedling emergence occurs in the third paper in this series (Jovaag, Dekker & Atchison, 2009b).
2.2.3. Mortality
Known mortality (dead intact seeds and dead seedlings) during the first year after burial (1998 season for 1997 populations, 1999 season for 1998 populations) was analyzed using ANOVA with year, populations within year, nurseries, seasonal time (JW groups), and their interactions as factors. Mortality for O97-37 during the second year after burial (1999 season) was analyzed using ANOVA with seasonal time (JW group) as the independent factor. Dead intact seeds and dead seedlings were analyzed separately with the same ANOVA structure as total mortality. As mean mortality within populations and JW groups increased, variability also increased. Thus the square roots of the mortality numbers were used in the analyses for total mortality, dead intact seeds, and dead seedlings to stabilize the variance.
2.2.4. Unknown
The total numbers of seeds in the unknown category were analyzed using ANOVA. Burial year. Seed recovery technique efficiency was estimated using the numbers of seeds in the unknown group from cores extracted during the burial year (1997 for O97-37; 1998 for C98-36 and J98). These numbers were analyzed using ANOVA with population, nursery and their interaction as factors. First year post-burial. The numbers of unknown seeds during the first year after burial (1998 season for 1997 populations, 1999 season for 1998 populations) were analyzed using ANOVA with year, populations within year, nurseries, seasonal time and their interactions as factors. Seasonal time was divided into four week periods beginning at JW 17 (JW groups; i.e. JW group 1=JW17-20, JW group 2=JW21-24, etc.) because cores were extracted monthly for each population and nursery, but not all could be done during the same week because of time constraints. Second year post-burial. Unknown seeds during the second year after burial from O97-37 at Johnson nursery were analyzed using ANOVA with JW group as the only factor.
3. Results
There were six observable fates for seeds from extracted cores: fully after-ripened seed, dormant seed, emerged seed, dead intact seed, dead seedling, and unknown. Generally, the fates of 50-90 percent of the buried seeds were known (Figure 1).
3.1. Living Seed Fates
3.1.1. Fully After-ripened and Dormant Seed
Generally, the proportion of recovered,fully-after-ripened (AR) seeds was high in the spring, declined, and remained low throughout the summer, then increased again in the autumn (Figure 2). The proportions of AR seed in the spring (JW16-31) and autumn (JW32-46) periods were modelled separately. There were two primary reasons for this approach. First, the rate of decline in the number of fully-after-ripened seed during late spring/early summer and the rate of increase in the autumn were not consistent. Modelling spring and autumn separately allowed for specification with the minimum number of parameters. Second, no late autumn (after JW42) data was available for the 1997 populations. Three parameter Lorentzian response functions (equation 2) were used to model the data where appropriate (Figure 2, Table 2).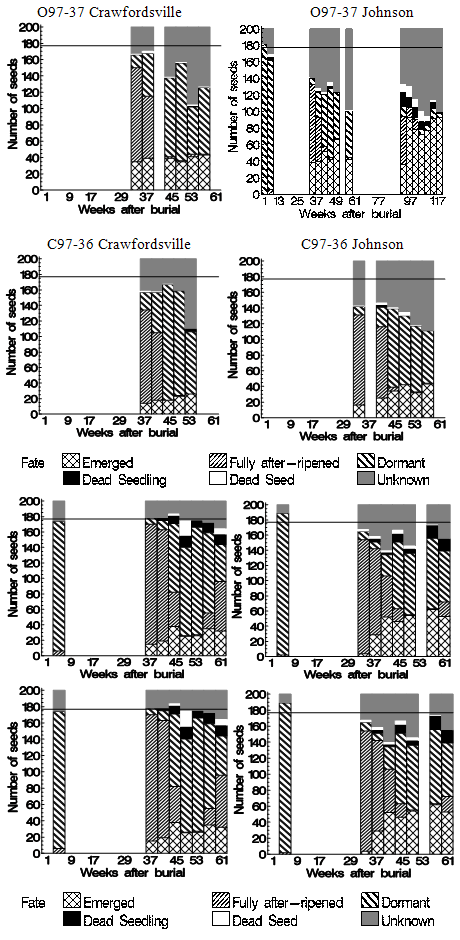 | Figure 1. Seeds (mean number) with each fate (fully after-ripened seed, dormant seed, emerged seed, dead intact seed, dead seedling, and unknown) from the S. faberi populations buried in 1997 (top four graphs) and 1998 (bottom four graphs) at the Crawfordsville (left) and Johnson (right) nurseries during the 60-117 weeks after burial (see Table 1 for burial dates). Bars represent all seeds extracted from the soil during a four week period; lengths of the divisions within a bar indicate the mean number of seeds per core of 200 with each fate. The reference line at 177 represents the average number of seeds recovered shortly (2-45 days) after burial. |
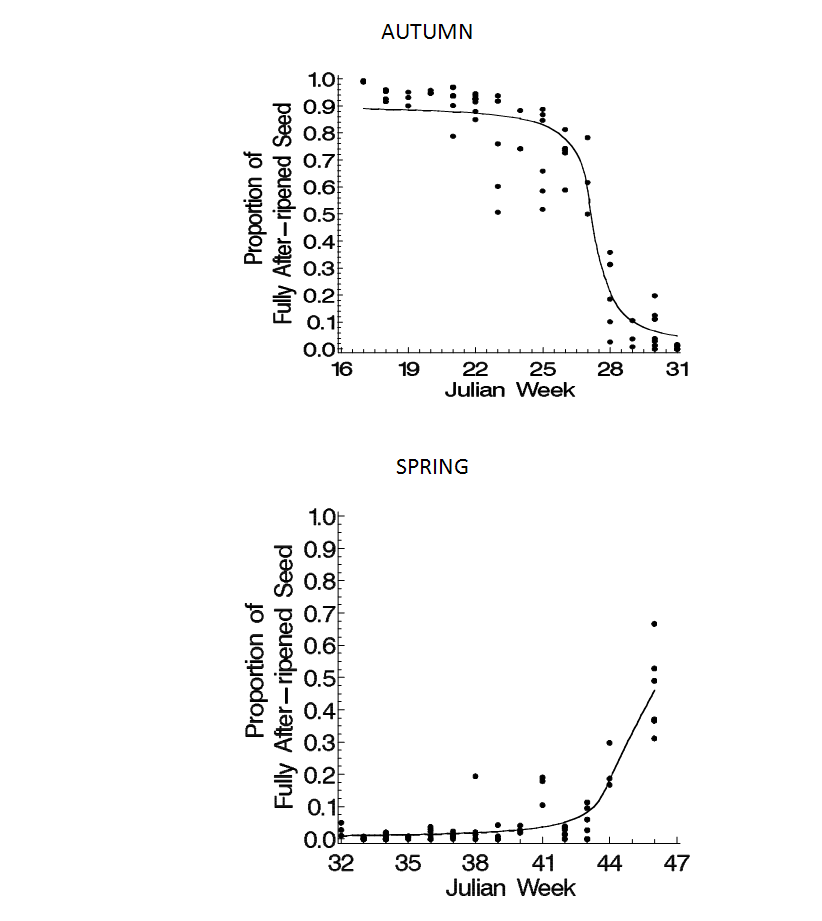 | Figure 2. Fully after-ripened live seed (proportion of recovered seed) versus Julian week (JW) for spring (JW 16-31, bottom) and autumn (JW 32-47, top) periods during the first year after burial for all populations, years and nurseries. Dots indicate proportions for a single core. Solid lines represent the fitted model (3 parameter Lorentzian functions with a power of the mean variance model; see equation 3 in materials and methods) where β0=maximum proportion, β1= JW at which the proportion is half the maximum, β2=half the time (JW) it takes for the proportion to go from 75% to 25% of the maximum (spring), or from 25% to 75% of the maximum (autumn). Parameter estimates (with standard errors): Spring, β0=0.91(0.02), β1=27.3(0.13), β2=-0.63(0.11); autumn, β0=0.54(.08), β1=44.4(0.42), β2=0.75(0.18). |
Spring germinability. 80-100% of the seeds were fully after-ripened early in the spring of the first year after burial (Figure 2, left). With time, the proportion declined as seed became dormant, emerged or died. Year. The maximum proportion of AR seeds (β0) was lower during the 1998 season (1997 populations, both nurseries; Table 2, top) than during the 1999 season (1998 populations, both nurseries). The time at which the proportion of AR seeds was half its maximum (β1) was similar between the two years, but the decline (β2) occurred more rapidly during the 1998 season.Population. The maximum proportion of AR seeds (β0) was lower for C97-36 than for either of the 1998 populations in the 1999 season (Table 2). The rate of decline in the proportion of AR seeds (β2) was slower for C98-36 than for C97-36 (in the 1998 season) or J98-36. The time at which the proportion of AR seed was half its maximum (β1) was earlier for O97-37 than for any other population in either season. Additionally, the proportion of O97-37 AR seed was half its maximum (β1) earlier at Johnson than at Crawfordsville. This was the only population and parameter for which a difference was found between the two nurseries.Second year post-burial. The proportion of AR seed in the spring of the second year after burial for O97-37 at Johnson (1999 season; Table 2) was not significantly different than that observed previously during the first year after burial (1998 season), primarily due to the larger variability in that second, 1999, season. Table 2. Lorentzian function (equation 2 in materials & methods) parameter estimates and standard errors (SE) for the proportion of fully after-ripened live seed (proportion of recovered seed) during spring (Julian week (JW) 16-31, top) and autumn (JW 32-47, bottom) periods for each season, each population, and for O97-37, each nursery. Estimates are for the first year after burial except for O97-37 2nd year. β0=maximum proportion, β1= JW at which the proportion is half the maximum, β2=half the time (JW) it takes for the proportion to go from 75% to 25% of the maximum (spring), or from 25% to 75% of the maximum (autumn). 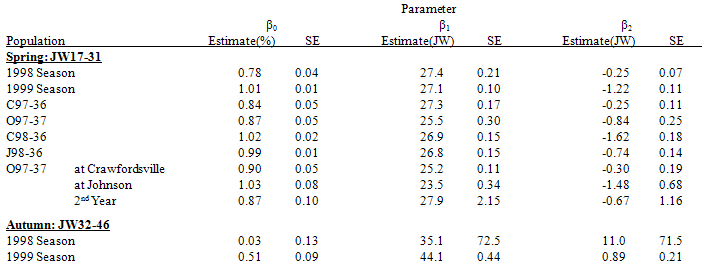 |
| |
|
Table 3. Difference (probability (p) values from ANOVA contrasts) in seed mortality ( dead intact seeds, dead seedlings, total) between the 1998 and 1999 seasons with time (Julian week (JW) group: 4 week periods beginning at JW17, top) and during the 1999 season between the Crawfordsville and Johnson nurseries and between the C98-36 and J98-36 populations at each nursery (bottom). NS=no significant difference (p>.05). 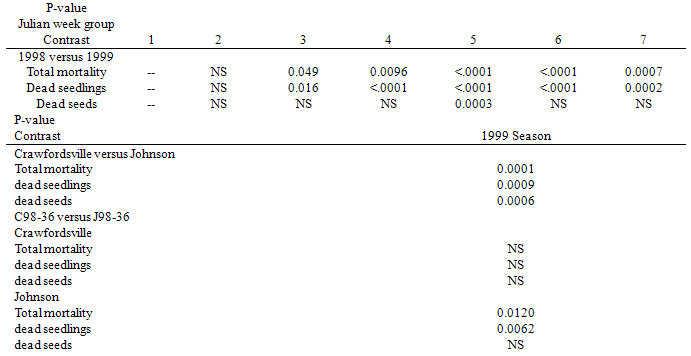 |
| |
|
Autumn germinability. The proportion of AR seed increased during the autumn of the 1999 season (Table 2, bottom). No increase was observed for the 1997 populations, but no data was available after JW42 for either the first (1998 season) or second (1999 season, O97-37 only) year after burial for those 1997 populations.
3.1.2. Emerged Seedlings
Emergence is crucial to the continuing life history of the seeds, and is reported herein (Figures 1 and 2) primarily as part of the overall seed recovery. Cumulative emergence by the end of the first year after burial was similar between the two years (late 1998 and 1999 seasons, p-value=.29). Seedling emergence is reported fully in the third paper in this series (Jovaag, Dekker & Atchison, 2009b).
3.2. Mortality
Total mortality numbers are conservative estimates because they consist only of dead intact seeds and dead intact seedlings. Broken or rotted seeds and seedlings were included with unknown because their fate was ambiguous.
3.2.1. Burial Year
3.2.2. First Year Post-Burial
Total mortality. Total mortality remained low (less than 10 of 200 seeds, 5%) throughout the 1998 season. Mortality during the 1999 season was greater than in the 1998 season after JW group 2 (Table 3, top). Total mortality was greater at Johnson than at Crawfordsville in the 1999 season (Table 3, bottom), but not in the 1998 season. Additionally, mortality of J98-36 was greater than that of C98-36 at Johnson during the 1999 season, but not at Crawfordsville (Table 3, bottom).Dead intact seeds. There were few dead intact seeds in either year. However, there were more dead intact seeds in the 1999 season than in the 1998 season during JW group 5 (JW33-36; Table 3, top). Also, there were more dead intact seeds at Johnson than at Crawfordsville during the 1999 season (Table 3, bottom). Dead seedlings. There were more dead seedlings during the 1999 season than during the 1998 season after JW group 2 (Table 3, top). There were more dead seedlings at Johnson than at Crawfordsville in the 1999 season (Table 3, bottom), but not in the 1998 season. Additionally, C98-36 had more dead seedlings than J98-36 at Johnson during the 1999 season (Table 3, bottom).
3.2.3. Second Year Post-Burial
Total mortality of O97-37 at Johnson during the second year after burial (1999 season) was greater during JW groups 1-4 (JW 17-32) than during JW group 7 (JW 41-44). Total mortality during JW group 1 (JW17-20) was also greater than mortality during JW groups 5-6 (JW33-40). However, there was no correlation between JW group and either dead intact seeds or dead seedlings (p-values>.11).
3.3. Unknown
The number of seeds in the unknown category depended on the time since burial and, during the first year after burial, on population and nursery.
3.3.1. Burial Year
An average of 23 (se 2.4) seeds (11.5% of 200) were in the unknown category during the burial year (2-45 days after burial). There was no statistical difference in the total unknown among populations or burial nurseries (ANOVA p-values > .10). These early data provided an estimate of the number of seeds lost due to the extraction process alone, and are therefore the basis for the reference lines in Figures 1 and 2.
3.3.2. First Year Post-Burial
The average number of unknown seeds was never more than 99 (49.5%) during the first year after burial, but varied depending on population, nursery (nursery*population(year) p-value=.0046), and seasonal time (JW group,p-value=.0001). The effect of the JW group on the unknown total did not depend on population or nursery (p-values of interactions with JW group >.09).Population and Nursery. Both 1998 populations at the Johnson nursery (1999 season) had fewer unknown seeds than the two 1997 populations at that nursery (1998 season; Table 4, top). At Crawfordsville, C98-36 (1999 season) had fewer unknown seeds than the 1997 populations at either nursery in the 1998 season. O97-37 at Johnson (1998 season) had more unknown seeds than O97-37 or C97-36 at the Crawfordsville nursery in that same 1998 season. There was no difference in the unknown total among the 1998 populations at the two nurseries (1999 season). Seasonal time. The total number of unknown increased at the end (after JW37) of the first year after burial (1998 season) for the 1997 populations (p-values <.005, Table 4, bottom). There was no association between seasonal time and the unknown total for the 1998 populations during the first year after burial (1999 season) (p-values>.42; Table 4, bottom).
3.3.3. Second Year Post-Burial
An average of 87 (se 4.9) seeds (43.5%) were in the unknown category from O97-37 at the Johnson nursery during the second year after burial (1999). The unknown total did not depend on seasonal time (p-value=.10). Additionally, the unknown total in the 1999 season (second year post-burial) for O97-37 was similar to that of the last collection date in the 1998 season (first year post-burial) for this same population and nursery (p-value=.37).Table 4. Seeds (Least Square mean (LSMean) no. per 200) with unknown fates during the first year after burial for each population and nursery (top) and for each Julian week (JW) group (4 week periods beginning at JW17, bottom). LSmeans in the same portion of the table with the same letter are not significantly different based on Tukey’s multiple comparison test (p=.05). 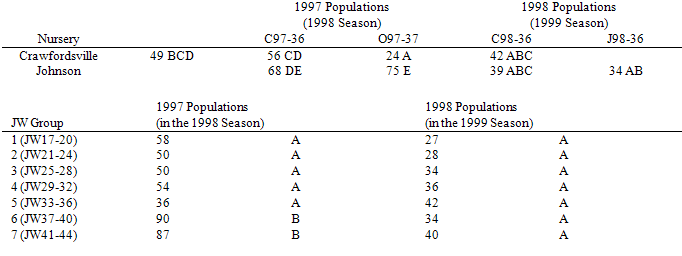 |
| |
|
4. Discussion
Introduction of four populations of S. faberi seeds with heterogeneous dormancy capacities into the soil of a soybean field resulted in the formation of enduring pools with varying seasonal cycles of dormancy, after-ripening, germination, dormancy reinduction and death (Figure 3). The buried seed rain of highly dormant seed after-ripened with time and became highly germinable, awaiting favourable temperature and moisture conditions: the heterogeneous germination candidate pool. As this pool was depleted in the spring and early summer by seedling emergence and death, dormancy was re-induced in the living seeds that remained in the soil. The seeds remained dormant throughout the summer, then resumed after-ripening during late autumn. This dormancy-germinability cycle exhibited complexity both within and among S. faberi populations: seed heteroblasty was retained and evidence of local adaptation was shown.
4.1. Live Seeds
Of the live seeds in this study, most (80-100%) germinated in ideal, controlled environmental, conditions by the spring of the first year after burial, but only a portion of those actually germinated and emerged in the field in the spring despite all experiencing similar seasonal environmental conditions (Jovaag, Dekker & Atchison, 2009b). Thus these fully after-ripened seeds may be considered germination candidates: seeds which are ready to germinate but await favourable conditions for germination in the field (enforced dormancy).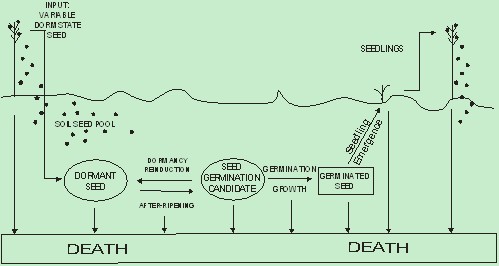 | Figure 3. Schematic diagram of weedy Setaria spp. soil seed pool behaviour based on the life history of the seed (seed states and processes (transitions between states)). Life history states: Dormant seeds, seed germination candidate, germinated seeds, seedlings, and dead seeds or seedlings. Life history processes: induction of dormancy and input (dispersal) of dormant seed at abscission (seed rain), after-ripening of dormant seed, dormancy re-induction of germination candidates, germination and growth of germination candidates, seedling emergence, and death. |
The proportion of germination candidates declined to near 0% by early summer (JW31). Thus dormancy was re-induced in the seeds that remained alive in the soil (Figure 5). This dormancy re-induction occurred as seasonal temperatures increased and moisture levels decreased during the late spring and early summer, after which most seeds would not germinate even if placed in ideal conditions. The germination candidate pool increased again in the autumn of 1999, the only year with data available for that late in the season (post JW42).These observations clearly indicate S. faberi seeds have an annual dormancy-germinability cycle similar to that of the summer annual weeds reported by Baskin and Baskin (1985): high spring germinability, summer dormancy re-induction, higher autumn germinability. Unlike that previous report, evidence is now provided that individual S. faberi seed populations retained heteroblastic qualities throughout this annual cycle, that cyclic responses to the yearly seasonal environment varied among populations, and that adaptation to local conditions occurred in the germinability of an individual population. The retention of heteroblasty within a population is evident in the variation of the proportions of candidate seeds within a single population and time, and the heterogeneous decline in germinability (Figure 2, Table 2), from early spring to early summer. The Lorentzian function model provided a sensitive method to distinguish this heteroblastic variation in the proportion of germination candidates over seasonal time among populations. Germinability responses to yearly environmental conditions were also shown: the proportion of germination candidates in the spring was lower, and the decline in germinability occurred more rapidly, during the 1998 season than the 1999 season. Local adaptation was evident in the differential germinability responses among S. faberi populations to the common nursery environments (i.e. a phenotype by environment interaction). This differential response occurred in both years: the decline in late spring germinability occurred more rapidly for J98-36 than C98-36 during the 1999 season (Table 2), and the midpoint of the decline in germinability occurred sooner for O97-37 than for C97-36 during the 1998 season. These observations provide evidence that the heterogeneous dormancy capacity among individual seeds remained, consistent with the hypothesis that dormancy capacity is an inherent quality of the seed and dormancy capacity heterogeneity of the population remains over time.
4.2. Mortality
Seed mortality patterns also exhibited complexity within and among populations. Within an individual S. faberi population, mortality patterns changed as seeds aged in the soil. Among S. faberi populations differential mortality responses were observed in response to yearly seasonal environments and common nurseries. Mortality is one of the most experimentally intractable estimates to make when accounting for seed behaviour in the soil. Dead S. faberi seeds can quickly rot and disappear from the soil, while others are removed by predation. Seed and seedling pieces provide ambiguous results as it is difficult to determine from what individuals they came. However, clear, reliable estimates of mortality were provided by observations of the dead intact seeds and seedlings. These estimates provided evidence of differential mortality responses to the yearly seasonal environment, of mortality differences among populations, and that, within an individual S. faberi population, mortality patterns changed as seeds aged in the soil. The mortality response to the yearly seasonal environment was shown by the greater mortality during the 1999 season, mostly due to greater fatal germination in the 1999 season than in the 1998 season. Heterogeneity of mortality among populations was indicated by the greater mortality of J98-36 than C98-36 at Johnson during the 1999 season (the location and year with the greatest overall mortality). Changes in mortality patterns with seed age were evident for O97-37 at Johnson, the only population and nursery studied for two years. Mortality of O97-37 remained low throughout the first year after burial (1998 season, 33-56 weeks after burial (wab)). But, during the second year after burial (1999 season), mortality was greater in the spring (JW17-20; 91-95 wab) than it was later in the season (JW 33-44; 108-119 wab) for O97-37 at Johnson. This is not solely a result of environmental conditions. Unlike the two year old O97-37 seeds in the 1999 season, the first year mortality of the 1998 populations during that same 1999 season was low in the spring (JW17-24; 30-40 wab), then higher during the rest of the season.Observations of both the germinability cycling differences among the live seeds and the mortality differences are consistent with the hypothesis that S. faberi seed behaviour in the soil is predicated on dormancy capacity heterogeneity at abscission—among seeds within a population and among locally adapted populations—modulated by the seasonal environmental conditions experienced in the field. Seeds with ambiguous or indeterminate fates in the soil were classified as unknown. Despite their equivocal nature, some inferences were possible. Differences in the unknown fraction occurred between years: the 1999 season had fewer such seeds, (along with greater known mortality) than the 1998 season. Evidence that unaccounted for seed losses were not due to migration out of the core area was also provided by the unknown fraction. During the late autumn and early spring, migration is most likely because of soil heaving caused by freezing and thawing. Little germination or rotting occurs between late autumn and early spring, thus if seed losses did occur during those months, they would most likely be due to migration. However, no seed losses were observed for the 1998 populations: the unknown fraction in the spring of the first year after burial (1999 season; 32-36 wab) was similar to that of the last collection time during the burial year (1998 season; 3-6 wab). Results for O97-37 were equivocal: there was an increase in the unknown fraction between the autumn of the burial year (1997 season; 3 wab) and the spring of the first year after burial (1998 season, 33 wab), but a decrease in the unknown fraction from the autumn of the first year after burial (1998 season; 56 wab) to the spring of the second year after burial (1999 season, 91 wab).
4.3. Experimental Recovery
Recovery of seed from unbound soil cores in this research was very good, approximately 88.5% of the buried seed was recovered during the burial year. The same extraction techniques were used throughout the study, thus it was reasonable to assume seed recovery remained relatively constant. The utility of this “bare core” technique was emphasized by the lack of evidence for seed movement out of the core provided above. Other burial techniques use seed-soil cores enclosed by artificial barriers (walls, bags, screened layers, jars) made from plastic, metal, glass, or treated wood to aid in seed recovery, but these barriers introduce experimental artefacts when making estimates of seed fates (Buhler & Hartzler, 2001; Burnside, et al., 1996; Davis, et al., 2005; Grundy, Mead, & Burston, 2003; Stoller & Wax 1973). For example, barrier materials possess different temperature and moisture holding qualities than soil. This alters the temperature and moisture conditions seeds experience, thus influencing their germinability. These barriers also prevent the natural movement of moisture, gases and predators within the soil, again influencing seed behaviour. Treated wood barriers (i.e Buhler & Hartzler, 2001) also introduce preservative chemicals into the soil which are toxic to soil organisms. Thus if our experience is more generally applicable, the good seed recovery and low risk of seed migration from unbounded cores, together with the high risk of artefacts from bounded cores altering seed behaviour in the soil, provide compelling reasons to consider use of bare cores when making estimates of seed fate in the soil. The soil weed seed pools of agricultural fields are the source of future annual weed infestations. Understanding the behaviour of soil seed pools is an essential predicate to prediction of the relative timing of seedling recruitment to advise weed control tactic use, the first critical step in any sustainable weed management system.
References
[1] | Burnside OC, Fenster CR, Evetts LL, Mumm RF (1981) Germination of exhumed weed seed in Nebraska. Weed Science 29, 577-586 |
[2] | Burnside OC, Wilson RG, Weisberg S & Hubbard KG (1996) Seed Longevity of 41 Weed Species Buried 17 years in Eastern and Western Nebraska. Weed Science 44(1), 74-86 |
[3] | Baskin JM & Baskin CC (1985) The Annual Dormancy Cycle in Buried Weed Seeds: A Continuum. BioScience 35(8), 492-498 |
[4] | Buhler DD & Hartzler RG (2001) Emergence and persistence of seed of velvetleaf, common waterhemp, wooly cupgrass and giant foxtail. Weed Science 49(2), 230-235 |
[5] | Carroll RJ & Ruppert D (1988) Transformation and Weighting in Regression. New York: Chapman and Hall |
[6] | Cottrell HJ (1947) Tetrazolium salt as a seed germination indicator. Nature 159, 748 |
[7] | Davis AS, Cardina J, Forcella F, Johnson GA, Kegode G, Lindquist JL, Luschei EC, Renner KA, Sprague CL & Williams II (2005) Environmental factors affecting seed persistence of annual weeds across the U.S. corn belt. Weed Science 53, 860-868 |
[8] | Dekker J (2003) The foxtail (Setaria) species-group. Weed Science 51, 641-646 |
[9] | Dekker J, Dekker BI, Hilhorst H & Karssen C (1996) Weedy adaptation in Setaria spp.: IV. Changes in the germinative capacity of S. faberi embryos with development from anthesis to after abscission. American Journal of Botany 83(8), 979-991 |
[10] | Dekker J, Atchison B, & Jovaag K (2003) Setaria spp. seed pool formation and initial assembly in agro-communities. Aspects of Applied Biology 69, 247-259 |
[11] | Dekker J & Hargrove M (2002) Weedy adaptation in Setaria spp.: V. Effects of gaseous atmosphere on giant foxtail (Setaria faberi) (Gramineae) seed germination. American Journal of Botany 89, 410-416 |
[12] | Flemion F & Poole H (1948) Seed viability tests with 2, 3, 5-triphenyl tetrazolium chloride. Contributions to the Boyce Thompson Institute 15, 243-258 |
[13] | Forcella F, Webster T, & Cardina J (2003) Protocols for weed seed bank determination in agro-ecosystems. In: Weed Management for Developing Countries, Addendum I. (ed R. Labrada). FAO-Rome Plant Production and Protection Paper 120, 3-18 |
[14] | Grundy AC, Mead A, & Burston S (2003) Does weed seed sowing density significantly effect weed emergence response to burial depth? Aspects of Applied Biology 69, 39-46. |
[15] | Menalled FD, Marino PC, Renner KA, & Landis DA (2000) Post-dispersal weed seed predation in Michigan crop fields as a function of agricultural landscape structure. Agriculture, Ecosystems and Environment 77, 193-203 |
[16] | Stoller EW & Wax LM (1973) Periodicity of germination and emergence of some annual weeds. Weed Science 21(6), 574-580 |