B. A. Abd-El-Nabey1, S. El-Housseiny2, H. M. El-Kshlan3, M. A. Abd-El-Fatah3
1Faculty of Science, Chemistry Department, Alexandria University, Alexandria, Egypt
2Faculty of Science, Chemistry Department, Kuwait University, Kuwait
3Faculty of Education, Alexandria University, Alexandria, Egypt
Correspondence to: B. A. Abd-El-Nabey, Faculty of Science, Chemistry Department, Alexandria University, Alexandria, Egypt.
Email: |  |
Copyright © 2017 Scientific & Academic Publishing. All Rights Reserved.
This work is licensed under the Creative Commons Attribution International License (CC BY).
http://creativecommons.org/licenses/by/4.0/

Abstract
Potentiodynamic polarization, electrochemical impedance spectroscopy, SEM study and EDX analysis have been used to investigate effect of Tween-80, Cetyl trimethyl ammonium bromide (Cetrimide), and Sodium Louryl Sulphate (SLS) surfactants on the corrosion resistance, porosity, structure and composition of Zn-phosphate coat on steel. Electrochemical results indicated that the presence of 0.01M surfactants in the coating solution caused a decrease in the porosity and an increase of the protection efficiency of the coat up to about 90%. The results proved that the coating process was controlled by adsorption of the surfactant molecules on the steel surface. Application of the adsorption isotherms and Kinetic-Thermodynamic model to fit the experimental data indicated that Langmuir isotherm is not applicable, however, Flory-Huggins isotherm and Kinetic-Thermodynamic model are applicable, and the three surfactants are chemisorbed on the steel surface. SEM study and EDX analysis gave very good support to the electrochemical data.
Keywords:
Steel, Conversion Coating, Zn-Phosphate, Surfactant, EIS, Polarization, SEM, EDS
Cite this paper: B. A. Abd-El-Nabey, S. El-Housseiny, H. M. El-Kshlan, M. A. Abd-El-Fatah, Effect of Surfactants on the Protection Efficiency of Zn-Phosphate Coat on Steel, Physical Chemistry, Vol. 7 No. 4, 2017, pp. 75-86. doi: 10.5923/j.pc.20170704.01.
1. Introduction
Besides several useful properties like strength, workability, low-cost, ability to be recycled, and good mechanical workability, steel have a major disadvantage, it shows poor corrosion resistance in corrosive environment and because of this efforts have been carried out to enhance its corrosion resistance. There are several methods to protect steel from corrosion. Control of corrosion through the use of conversion coatings, is the most effective method and used in a wider range of applications [1-6]. Conversion coatings are applied to metal surfaces to produce a protective film, which is less reactive in aggressive environments than the original metal surface [6]. Phosphating is a conversion coating process that has been used for steel to receive a liquid, powder, or paint, and increase the adhesion and corrosion resistance [7, 8]. The phosphate conversion coating treatment has been used for many years in the industry due to its attractive corrosion protection properties and simplicity in operation, and the resultant exponential growth in automobiles and domestic appliances, zinc phosphate coatings for protecting steel surfaces have gained prominence since the turn of the century [9].Surfactants are usually organic compounds that are amphiphilic, meaning they contain both hydrophobic groups (their tails) and hydrophilic groups (their heads). Most commonly, surfactants are classified according to polar head group. A non-ionic surfactant has no charged groups in its head such as Tween-80. The head of an ionic surfactant carries a net positive or negative charge. If the charge is negative, the surfactant is more specifically called anionic such as (SLS); if the charge is positive, it is called cationic such as Cetrimide. Due to the ability of the surfactants to adsorb on the surface, they have a great influence on the growth of crystals and have the ability to alter crystallization kinetics. Recently, we studied the effect of Tween-80 surfactant on each of (1) electropolymerization and corrosion performance of polyaniline on carbon steel [10] and (2) corrosion resistance, porosity, structure and composition of Zn-Phosphate coat on steel in aqueous 0.6M ammonium nitrate. It has been found that the presence of Tween-80 in the polymerization medium increased the protection efficiency of the polyaniline coat to the corrosion of steel in 0.5M H2SO4 to about 80%. However, the presence of 0.01M Tween-80 in the Zn-Phosphate coating solution caused a decrease in the porosity and an increase of the protection efficiency of the coat up to 90%.The aim of this work is to study the effect of the presence of the three surfactants, Tween-80, Cetrimide, and SLS in the Zn-Phosphate coating medium on the corrosion resistance of the coated steel in 0.5M H2SO4 solution. The effect of the nature of the surfactant on the protection efficiency, porosity and composition of the Zn-Phosphate coat should be investigated using the electrochemical techniques, SEM, EDX analysis.
2. Experimental
2.1. Materials and Solutions
85% H3PO4, ZnO, NaNO2, and H2SO4 were purchased from Aldrich chemicals. Cetrimide, Tween-80, and SLS (Figure 1) were obtained from Alpha and 0.02M stock solutions of surfactants were used to prepare the test solutions.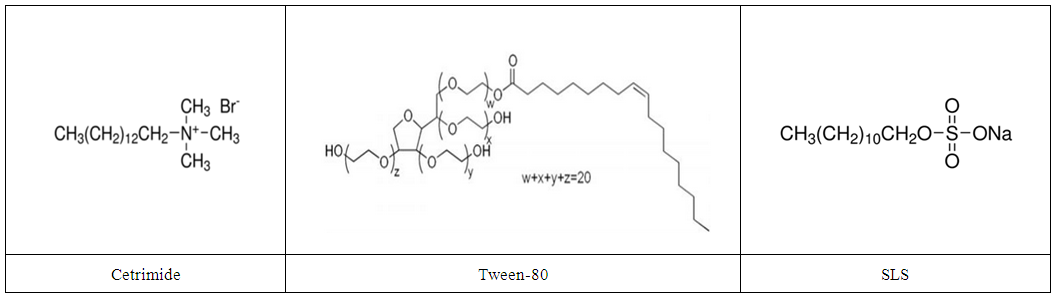 | Figure 1. Chemical structures of Cetrimide, Tween-80 and SLS surfactants |
To prepare the conversion coating solution, 0.75g of ZnO, 1g of NaNO2, 2 ml H3PO4 and an appropriate volume of the stock solution of the surfactant was added and completed to 100 ml with double distilled water to obtain the required concentration of the surfactant.
2.2. Application of Conversion Coating
The conversion coating was applied on the steel samples using the same method which has been reported in the recent previous work [9].
2.3. Electrochemical Measurements
Electrochemical impedance and polarization measurements were achieved using frequency response analyzer (FRA)/potentiostat supplied from Parstat Instrument (PARSTAT 2263.02 SN 194). The frequency range for EIS measurements was 1.0 × 104 to 0.1Hz with applied potential signal amplitude of 10 mV around the rest potential. The data were obtained in a three- electrode mode cell; graphite rod and saturated calomel electrodes (SCE) were used as counter and reference electrode. The working electrode was chemically treated or untreated steel that had the chemical composition (% wt) 0.21% C, 0.35% Si, 2.5% Mn, 0.04% P, and 0.04% S, rest Fe) has been used for the electrochemical corrosion studies in 0.5 M H2SO4 solution. The polarization and EIS measurements have been recorded using the same procedure that has been reported in the previous work [9].
2.4. Scanning Electron Microscope (SEM) and Energy Dispersive X-ray Spectrometer (EDS)
SEM was employed to characterize the surface morphology with JOEL instrument. Coupons with area 1 cm2 steel and with the same chemical composition of steel samples used in the electrochemical measurements were used in the experiments. The elemental composition of Zn-phosphate coat was analyzed by energy dispersive X-ray spectrometer (EDS, JEM-2100, Japan).
3. Results and Discussion
3.1. Effect of the Surfactants on the Protection Efficiency of the Coat
3.1.1. Potentiodynamic Polarization Results
Polarization curves of Zn-Phosphated Steel prepared from coating solutions containing different amounts of Cetrimide, SLS or Tween-80 surfactants in 0.5 M H2SO4 are presented in Figure 2(a, b, c). As seen from the figure, addition of the surfactants leads to the polarization of both the cathodic and the anodic curves.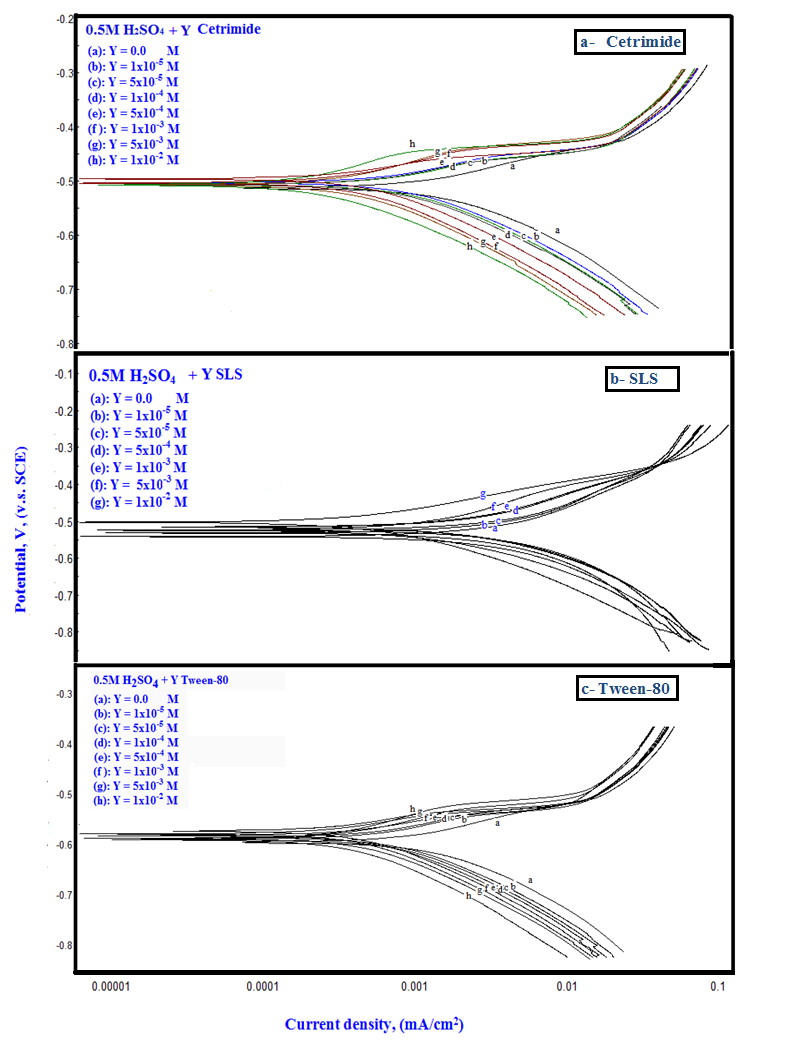 | Figure 2. Polarization curves of Zn-Phosphated Steel prepared from coating solutions containing different amounts of Cetrimide, SLS or Tween-80 surfactants in 0.5 M H2SO4 |
Tables (1-3) show the electrochemical polarization parameters; the corrosion current density, (icorr), corrosion potential (Ecorr); and anodic, cathodic Tafel line slopes (βa, and βc) for Zn-Phosphated steel prepared from coating solutions containing different amounts of the three surfactants in 0.5 M H2SO4. The protection efficiency of the coat was calculated using the equation: | (1) |
Where io and i are the corrosion current density, in absence and presence of the surfactant. Table 1. The potentiodynamic polarization parameters for Zn-Phosphated steel prepared from coating solutions containing different amounts of Cetrimide surfactant in 0.5 M H2SO4 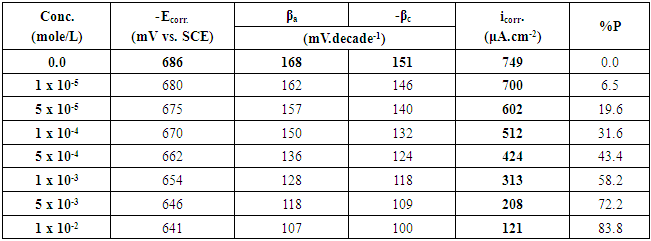 |
| |
|
Table 2. The potentiodynamic polarization parameters for Zn-Phosphated steel prepared from coating solutions containing different amounts of SLS surfactant in 0.5 M H2SO4 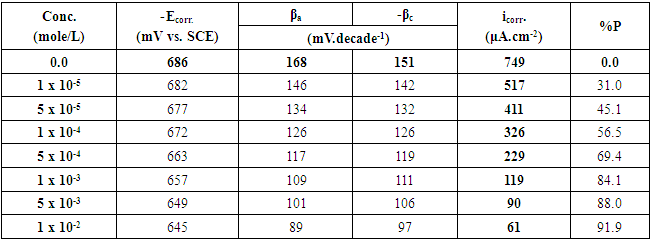 |
| |
|
Table 3. The potentiodynamic polarization parameters for Zn-Phosphated steel prepared from coating solutions containing different amounts of Tween-80 surfactant in 0.5 M H2SO4 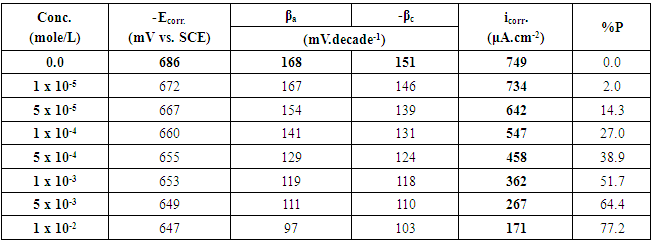 |
| |
|
The above tabulated data show the followings:1) The presence of the surfactants in the coating medium leads to the shift of the corrosion potential of the coated steel to less negative potentials which is probably due to the protection of the steel by the coat.2) The values of each of βa and βc of the coated steel markedly decrease with increasing the concentration of the surfactant which can be explained on the basis of the decrease of the porosity of the coat in presence of the surfactants.3) The corrosion current decreases and the protection efficiency of the coated steel increases with increasing the concentration of the surfactant in the coating medium which is probably due to the decrease of the porosity and increase of the thickness of the coat in presence of the surfactant.
3.1.2. Electrochemical Impedance Spectroscopy (EIS) Results
Nyquist impedance plots of bare steel and Zn-Phosphated Steel prepared from coating solutions containing different amounts of Cetrimide, SLS or Tween-80 surfactants in 0.5 M H2SO4 are presented in Figure 3(a,b,c). The Nyquist plots consist of distorted semicircles followed by diffusion tail indicative that the corrosion process occurs under diffusion control. All impedance spectra were analyzed by fitting the experimental data to the equivalent circuit model shown in Figure 4 [11]. 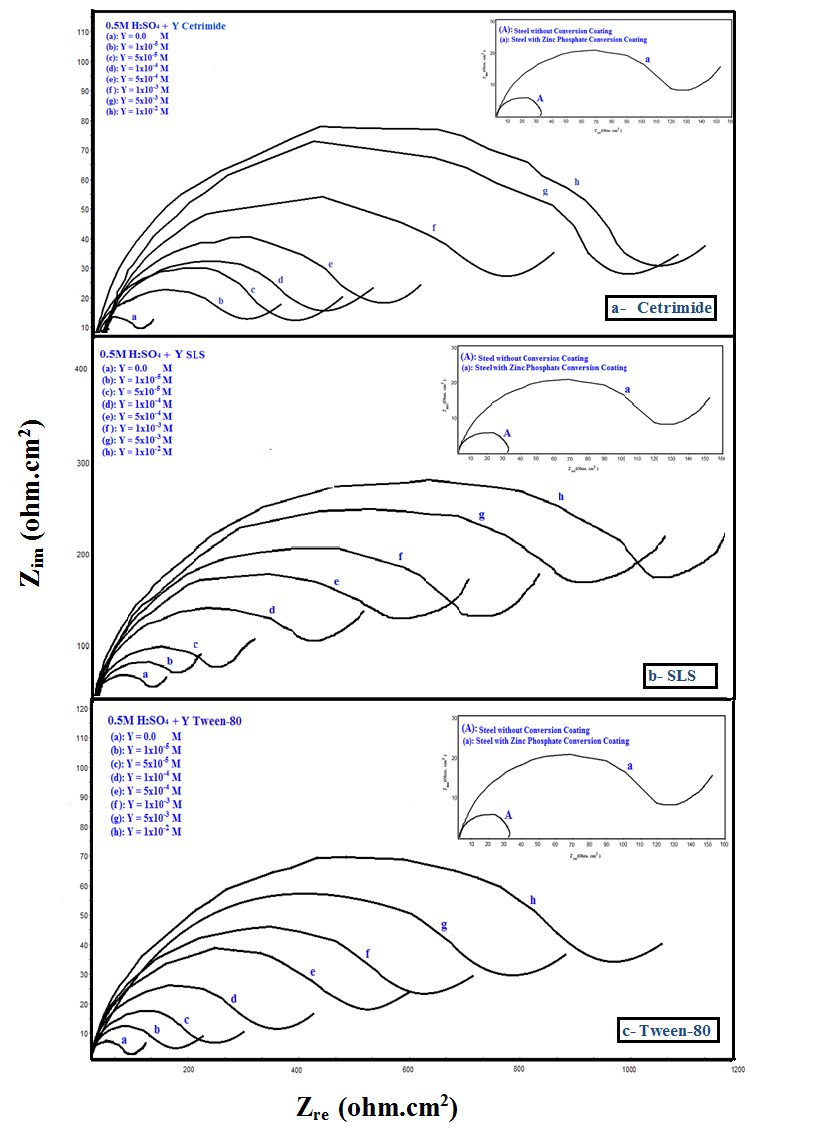 | Figure 3. Nyquist plots of bare steel and Zn-Phosphated steel prepared from coating solutions containing different amounts of Cetrimide, SLS or Tween-80 in 0.5 M H2SO4 |
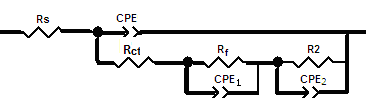 | Figure 4. Schematic for the equivalent circuit of coated steel |
The protection efficiency of the coat (% P) was calculated from the impedance measurements using the relation: | (2) |
Where Rct and Rcto are the charge transfer resistances, in presence and absence of surfactants respectively. The data shown in Tables (4-6) indicate that the increase in the surfactant concentration leads to an increase of the charge transfer resistance, a decrease of the double layer capacitance and an increase of the protection efficiency of the coat. This behaviour confirm the results obtained from the polarization measurements and can be discussed on the basis of the increase of the compactness and the decrease of the porosity of the coat in presence of the surfactant during the coating process of steel.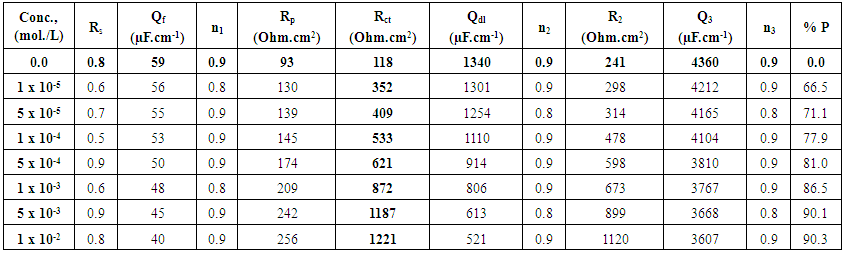 | Table 4. Electrochemical impedance parameters of Zn-Phosphated steel prepared from coating solutions containing different amounts of Cetrimide surfactant in 0.5 M H2SO4 |
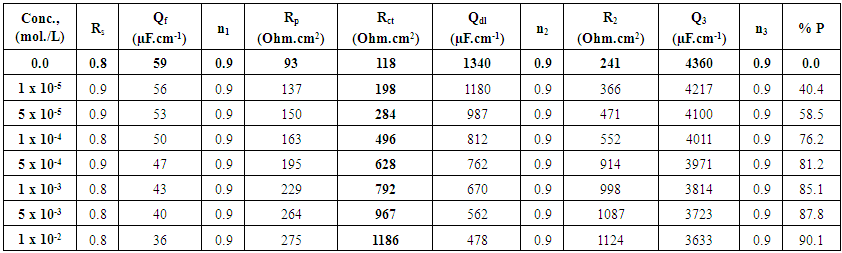 | Table 5. Electrochemical impedance parameters of Zn-Phosphated steel prepared from coating solutions containing different amounts of SLS surfactant in 0.5 M H2SO4 |
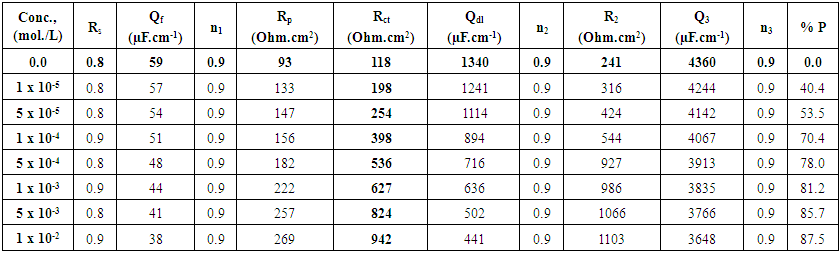 | Table 6. Electrochemical impedance parameters of Zn-Phosphated steel prepared from coating solutions containing different amounts of Tween-80 surfactant in 0.5 M H2SO4 |
Figure 5 shows the variation of the protection efficiency of Zn-Phosphate coat which obtained from the impedance results with the concentration of the examined surfactants. These curves show initial steeply rising part adsorption isotherm which indicates a mono-layer adsorbate film formation on the steel surface. As seen from the figure, the charged surfactants (Cetrimide and SLS) have high protection efficiency than neutral surfactant (Tween-80). This phenomenon can be discussed on the basis that the coating process is mainly controlled by the adsorption of the surfactant molecules or ions at the steel/solution interface.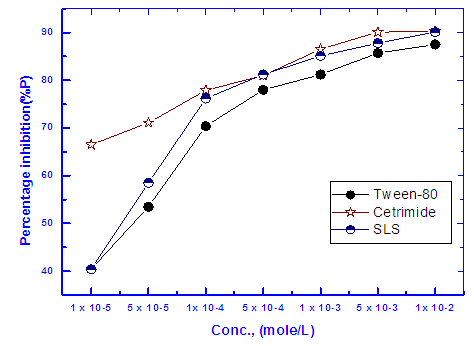 | Figure 5. Relation between protection efficiency of the coated steel and concentration of the surfactants |
3.2. Porosity of Coat
The pores in the coat are generally regarded as the exposed area of the substrate, which can be measured from the results of the potentiodynamic polarization and electrochemical impedance spectrophotometry techniques [12, 13]. Equation (3) was used for this purpose [14]:  | (3) |
Where Rps and Rp are the polarization resistances of the untreated and chemically treated samples, respectively. ΔEcorr is the difference between the corrosion potentials of the untreated and chemically treated samples. βa is referred to slope of the anodic Tafel line derived from the polarization curves. The porosity values of the zinc-phosphate coat on the steel surface in absence and presence of different concentrations of the three surfactants during the coating process were calculated using the electrochemical data presented in Tables (1-6) and given in Table 7.Table 7. The electrochemical parameters used in determination of the porosity of Zn-phosphate coat on steel in absence and presence of different concentrations of Cetrimide, SLS and Tween-80 surfactants 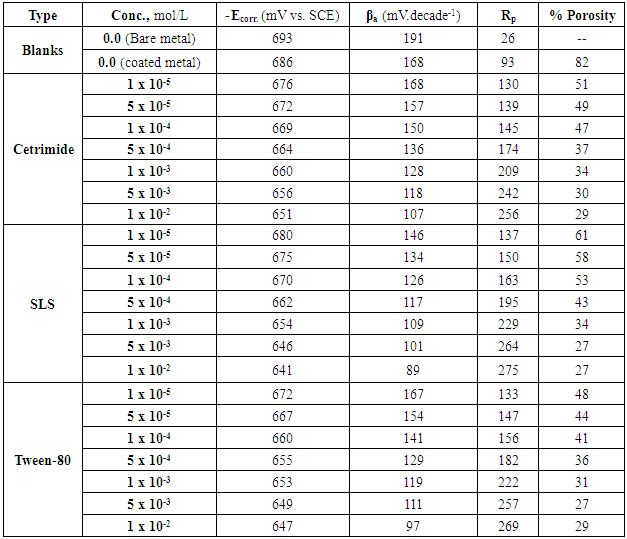 |
| |
|
Dependence of the porosity of Zinc-Phosphate coat on the concentration of Tween-80, Cetrimide and SLS is presented in Figure 6. It is clear that, in presence of low concentrations of the surfactants, porosity of the coat sharply decrease with increasing the concentration surfactants until
However, the presence of higher concentrations of the surfactants has a slight effect on the porosity of the coat, this behaviour can be discussed on the basis that the conversion coating process is mainly controlled by the adsorption of the surfactant molecules on the steel surface. The reduction in porosity and increase in corrosion resistance of Zn-phosphate coat on steel due to the presence of surfactants during the coating process can been discussed on the basis of the ability of these long-chain surfactants to form films over the surface helps to seal the pores effectively, causing low moisture permeability and high corrosion-resistance properties.Figure 6 shows also that the charged surfactants (Cetrimide & SLS) are more efficient in decreasing the porosity of Zn-phosphate coat on steel surface than the neutral one (Tween-80). This confirms the above results of the dependence of the protection efficiency of the coat on the concentration of the surfactants (Figure 5).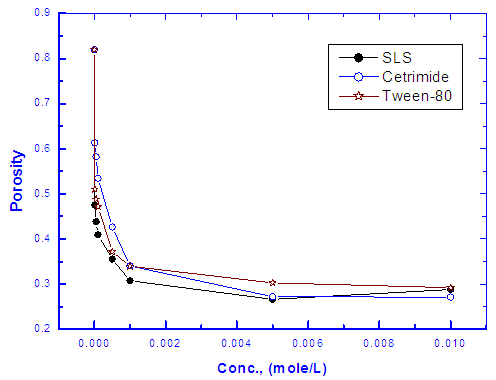 | Figure 6. Relation between the porosity of the coat and concentration of the surfactant |
3.3. Adsorption of the Surfactants at the Steel/Solution Interface
The Langmuir isotherm is given by 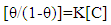 | (4) |
Where K is the binding constant representing the interaction of the additives with metal surface and C is the concentration of the additives. Flory-Huggins isotherm is given by 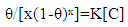 | (5) |
Where x is the size parameter and is a measure of the number of adsorbed water molecules substituted by a given surfactants molecules.And the Kinetic-Thermodynamic model is given by | (6) |
Where y is the number of surfactants molecules occupying one active site. The binding constant K is given by: | (7) |
Figures (7-9) show the application of the above mentioned models to the data of surfactants obtained from impedance measurements for Zn-Phosphated steel surface. The parameters obtained from the Figures are depicted in Table 8.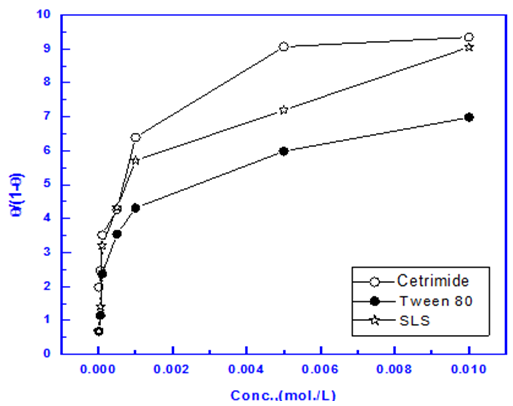 | Figure 7. Linear fitting of the data of the surfactants to Langmuir isotherm |
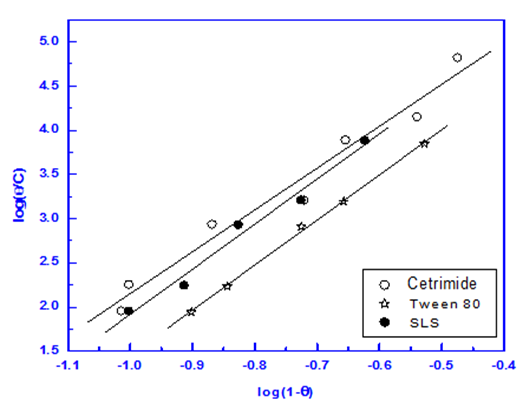 | Figure 8. Linear fitting of the data of the surfactants to Flory Huggins isotherm |
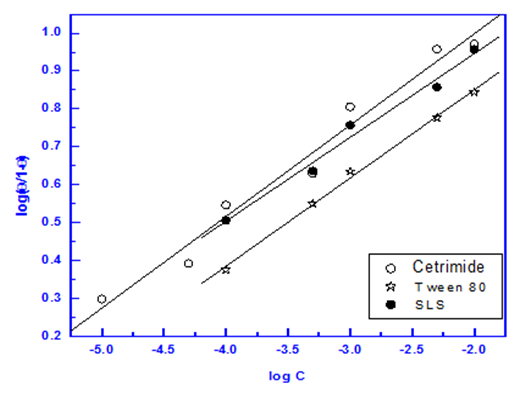 | Figure 9. Linear fitting of the data of the surfactants to Kinetic-Thermodynamic model |
Table 8. Linear fitting parameters of the data of the surfactants to the used models 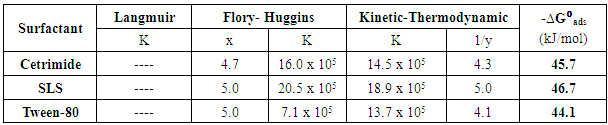 |
| |
|
It is clear that Langmuir isotherm is unsuitable to fit the data indicating that there might be non-ideal behavior in the adsorption processes [15] of surfactants on steel surface. On the other hand, Flory-Huggins isotherm and Kinetic-thermodynamic model are found to be applicable. Table 8 shows a good agreement between the values of K and (x&1/y) obtained from Flory- Huggins model and the Kinetic-Thermodynamic model. The values of the size parameter x indicates that five adsorbed water molecules were displaced by each surfactant molecule, the number of active sites occupied by a single surfactants molecules, 1/y, were nearly equal to the size parameter x. The binding constant K is related to the standard free energy of adsorption ΔG◦ads according to the following equation [16-18]:  | (8) |
Where; R is the universal gas constant, T is the absolute temperature, the value 55.5 is the concentration of water in solution expressed in mol/L. From the tabulated data, it is clear that, the charged surfactants have a large value of the standard free energy of adsorption than the neutral one and the three surfactants are chemisorbed at the steel surface. These results show a good agreement with that obtained from the dependence of each of the protection efficiency and the porosity of Zn-phosphate on steel on the concentration of the surfactants (Figures 5&6).
3.4. SEM Study and EDX Analysis
Figure 10 shows the SEM photographs of: A) bare steel; B) Converted coated steel; C, D, E) Converted coated steel in presence of 1 x 10-3 M Tween-80, Cetrimide or SLS respectively. It is clear that in the absence of surfactants during the coating process (B) the exposed area of the steel surface i.e. the porosity of the coat is large. On adding 1 x 10-3 M of surfactants during the coating process (C, D, E), the porosity of the coat decreases and the coat layer becomes more thick and compact.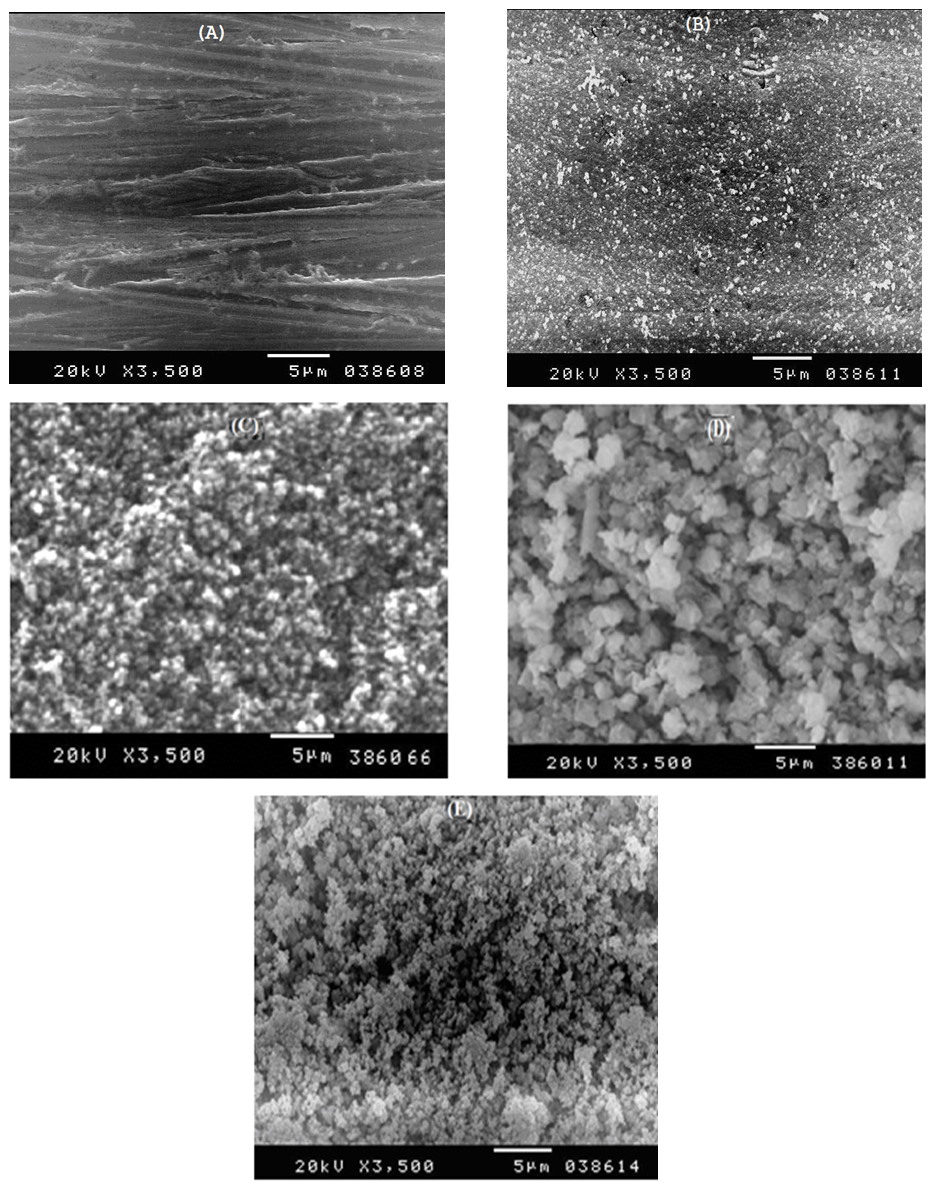 | Figure 10. SEM micrographs of (A) Bare steel, (B) Converted coated steel, (C) Converted coated + 1 x 10-3 M Cetrimide , (D) Converted coated + 1 x 10-3 M SLS, (E) Converted coated + 1 x 10-3 M Tween-80 |
The EDX analysis of the above five steel samples are given in Figure 11. The results in Table 9 indicating the following: (1) carbon is present in the coat which means that the Zn-phosphate conversion coats contain the surfactants. and (2) Percentage of each of Zn and P in the coat increases while the percent of iron decreases in presence of surfactants which indicates that the covered area of steel surface with the coat increases and porosity of the coat decreases. It is clear that SEM studies and EDX analysis gave very good support to the electrochemical data.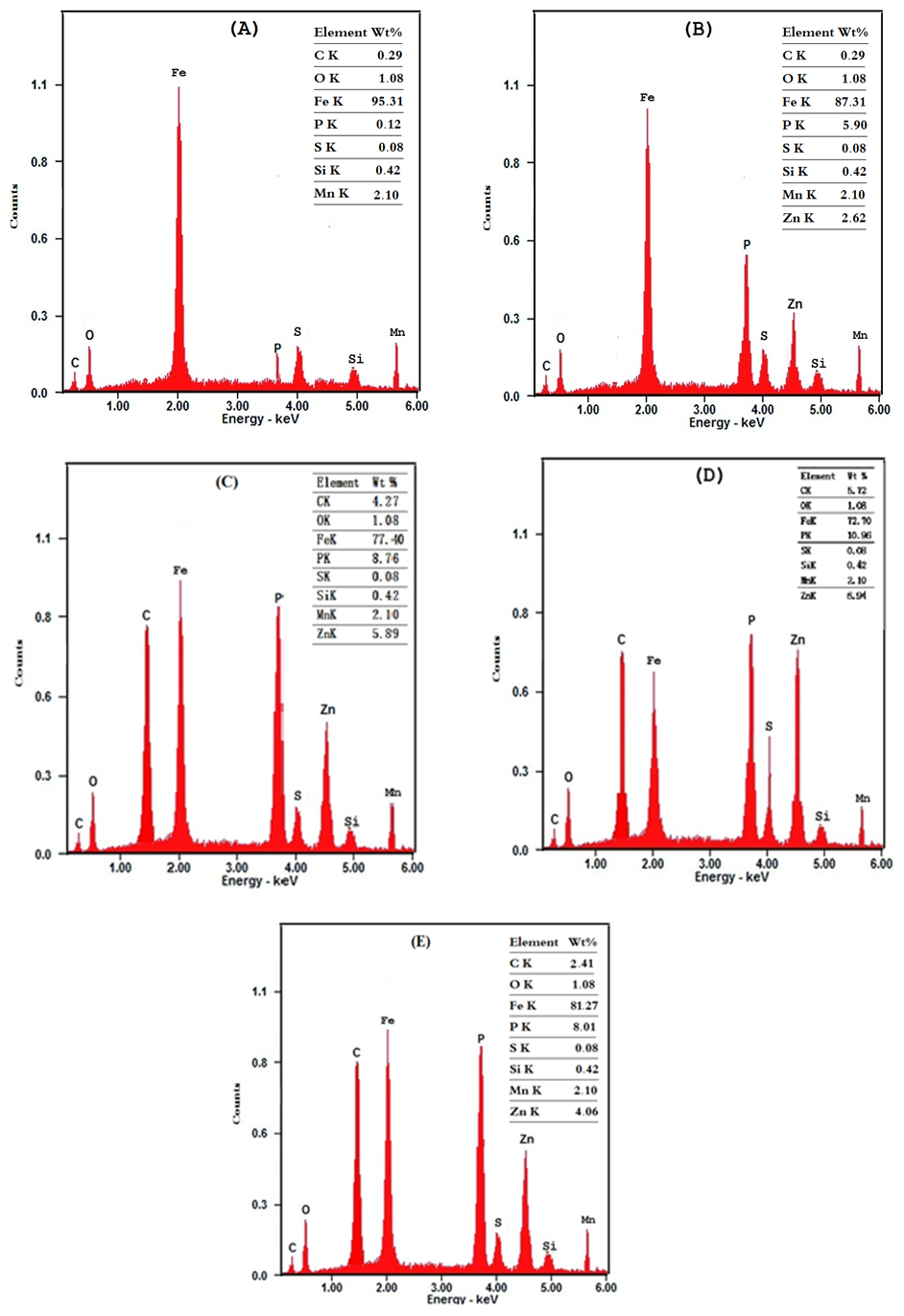 | Figure 11. EDS micrographs of (A) Bare steel, (B) Converted coated steel, (C) Converted coated + 1x10-3 M Cetrimide , (D) Converted coated + 1x10-3 M SLS, (E) Converted coated + 1x10-3 M Tween-80 |
4. Conclusions
1- Electrochemical results, SEM studies and EDX analysis indicated that the presence of the three investigated surfactants (Tween-80, Cetramide and SLS) in the coating medium caused a large increase of the protection efficiency of Zn-Phosphate coat on steel.2- The results indicated that the charged surfactants are more efficient than the neutral one in increasing the protection efficiency of the coat.3- This study confirmed the argument which stated that, the ability of the long-chain surfactants to form films over the metal surface helps to seal the pores effectively, causing low moisture permeability and high corrosion-resistance properties. Table 9. Weight percentage of C, P, Zn and Fe on surface of steel and Zn-Phosphated steel prepared from coating solutions containing surfactants 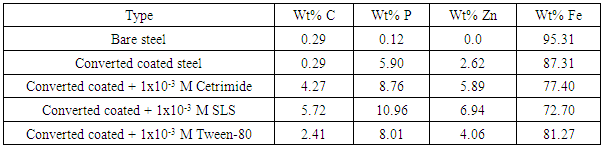 |
| |
|
References
[1] | R.C. Zeng, Z.D. Lan, J. Chen, X.H. Mo, and. E.H. Han, Nonferrous Metal Society of China, 19(2009) 397. |
[2] | B. L. Lin, J. T. Lu, G. Kong, and J. Liu, Transactions of Nonferrous Metals Society of China, 17(4)(2007)755. |
[3] | D. Wang, P. Jokiel, A. Uebleis and H. Boehni, Surf. & Coat. Tech., 88(1-3)(1997)147. |
[4] | T.S.N.S. Narayanan, Adv. Mater. Sci. 9(2005)130. |
[5] | R. Amini, A.A. Sarabi, Appl. Surf. Sci., 257(2011) 7134. |
[6] | E.P. Banczek, P.R.P. Rodrigues, and I. Costa, Surf. & Coat. Tech., 201 (4)(2006)3701. |
[7] | L.Y. Niu, Z.H. Jiang, G.Y. Li, Gu. C.D, and J.S. Lian, Surf. & Coat. Tech., 200 (9)( 2006)3021. |
[8] | L.C. Deepa, S. Sathiyanarayanan, C. Marikkannu, and D. Mukherjee, Anti-Corros. Meth. and Mater., 50(4)( 2006)286. |
[9] | B.A. Abd-El-Nabey, S. El-Housseiny, H. M. El-Kshlan, M. A. Abd-El-Fatah, Phys. Chem., 7(1)( 2017)17. |
[10] | B. A. Abd-El-Nabey, O. A. Abdullatef, G. A. El-Naggar, E. A. Matter and R. M. Salman, Int. J. Electrochem. Sci., 11 (2016) 2721. |
[11] | B.A. Abd-El-Nabey1, S. El-Housseiny, G. A. El-Naggar, E. A. Matter, G. Esmail, Phys. Chem., 5(3)( 2015)49. |
[12] | S.C. Lins, G.F. A. Reisb, C.R. Araujoa, and T. Matencio, Appl. Surf. Sci. 253 (2006) 2875. |
[13] | D. Weng, P. Jokiel, A. Uebleis, and H. Boehni, Surf. Coat. Tech. 88 (1996) 147. |
[14] | H.Y. Su, and C.S. Lin, Corros. Sci. 83 (2014) 137. |
[15] | B. Ramezanzadeh, Ind. and Eng. Chem. 30 (2015) 225. |
[16] | G. Lyberatos, and L. Kobotiatis, Corros. 47 (1991) 820. |
[17] | W. H. Li, Q. He, S. T. Zhang, C. L. Pei, and B. R. Hou, Appl. Electrochem., 38 (2008) 289. |
[18] | E. Khamis, Corros., 46 (1990) 476. |
[19] | G. Moretti, F. Guidi, and G. Grion, Corros. Sci., 46 (2004) 387. |