E. A. Gomaa, H. M. Abu El-Nader, Sh. E. Rashed
Chemistry Department, Faculty of Science, Mansoura University, 33516 Mansoura, Egypt
Correspondence to: E. A. Gomaa, Chemistry Department, Faculty of Science, Mansoura University, 33516 Mansoura, Egypt.
Email: |  |
Copyright © 2012 Scientific & Academic Publishing. All Rights Reserved.
Abstract
the association constants, formation constants and Gibbs free energies of solvation are calculated from the conductometric titration curves of CoCl2 with (E)-1-phenyl-2-(2-(4-((E)-phenyldiazenyl)phenyl)hydrazono)-2- (phenylsulfonyl) ethanone (L) in absolute ethanol at 293.15, 298.15, 303.15 and 308.15 K. On drawing the relation between molar conductance and the ratio of metal to ligand concentrations, different lines are obtained indicating the formation of 1: 2, 1:1 and 2:1 (M:L) stoichiometric complexes. The formation constants and Gibbs free energies of these different complexes in absolute ethanol at 293.15, 298.15, 303.15 and 308.15 K follow the order: Kf (2:1) > Kf (1:1) > Kf (1:2) for (M:L); and ∆Gf (2:1) > ∆Gf (1:1) > ∆Gf (1:2) for (M:L).
Keywords:
Association constant , formation constant , Gibbs free energy of solvation
1. Introduction
The long range ion–ion interactions due to columbic forces are the most important features of electrolyte in solutions. This act together with shorter – ranged forces between the solvent molecules and between the solvent molecules and ion. Electrical conductivity (EC) is a measure of solution to conduct electric current and depends on : concentration of the ions, solvent, ligand and temperature. Current is carried out by both cations and anions, but to different degree. The conductivity due to divalent cations is more impotant than that of monovalent cations, it is not true for anions. Metal cations with do noble gas electron configuration (alkali and alkaline earth) metal ions together with the inert molecular ions like tetraalkylammonium, -phosphonium, -arsonium, and trialkylsulfonium ions exhibit properties mainly determined by their charge and size[1]. Solvation of such cations in protic and polar solvents is due essentially to electrostatic ion-dipole and ion induced dipole interactions.Metal cations with filled d-orbitals, the d10 cations,exhibits partially covalent character in their interactions, their properties depend The synthesis of transition metal complexes with Schiff bases of nitrogen, oxygen and sulfur donor has stimulated interest due to their vast variety of biological activities ranging from pharmacological, antitumor, fungicide, bactericide, anti-inflammatory, and antiviral activities[4,5]. Cobalt is the active centre of called , the most common example of which is . As such it is an essential trace for all animals. Cobalt also is an active nutrient for bacteria, and [6,7]. The aim of this work is the evaluation of the non-covalent behaviour of CoCl2 in presence of (E) -1- phenyl -2- (2- (4-((E) -phenyldiazenyl)phenyl)hydrazono)-2-(phenylsulfonyl)ethanone (L1) in absolute ethanol solutions at 293.15, 298.15, 303.15 and 308.15 K. these non-covalent interactions can help us for analysis of salts role in bodies and environment. This work provides the biological analyst data can help him for deterring the concentration of CoCl2 in blood and different solutions.
2. Experimental
2.1. Material and methods
The cobalt chloride and ethanol were provided from Merck Co.
2.2. The Ligand
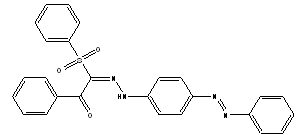 | Scheme 1. (E)-1-phenyl-2-(2-(4-((E)-phenyldiazenyl)phenyl)hydrazono)- 2-(phenylsulfonyl)ethanone |
2.3. Conductometric Titration
The conductometric titration of the ligand (1x10-3) mole/L against CoCl2 (1x 10-4) mole/L in absolute ethanol was performed with 0.5 ml interval additions from CoCl2 solution. The specific conductance values were recorded using conductivity bridge HANNA, H1 8819N with a cell constant equal to 1. The conductometer was conducted with a thermostat of the type the Kottermann 4130 ultrathermostat. The temperature was adjusted at 293.15, 298.15, 303.15 and 308.15 K.
3. Results and Discussion
The specific conductance values (Ks) of different concentrations of CoCl2 in absolute ethanol were measured experimentally in absence and in presence of ligand at 293.15, 298.15, 303.15 and 308.15 K.The molar conductance (
m) values were calculated[8] using equation  | (1) |
Where Ks and Ksolv is the specific conductance of solution and the solvent, respectively; Kcell is the cell constant and C is the molar concentration of the CoCl2 solutions.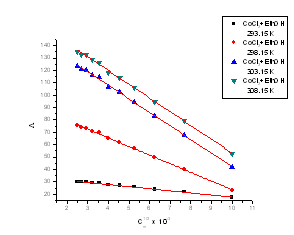 | Figure 1. The relation between molar conductance (M) and (√C) of CoCl2 alone in absolute ethanol at 293.15, 298.15, 303.15 and 308.15 K |
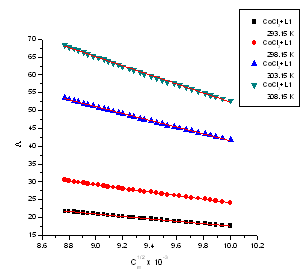 | Figure 2. The relation between molar conductance (M) and (√C) of CoCl2 in the presence of ligand (L) in absolute ethanol at 293.15, 298.15, 303.15 and 308.15 K |
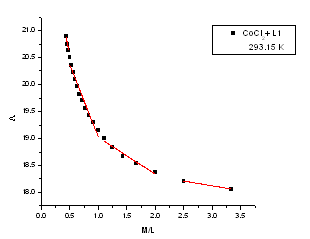 | Figure 3. The relation between molar conductance (M) and the molar ratio (M/L) of CoCl2 in presence of (L1) in absolute ethanol in 293.15 K |
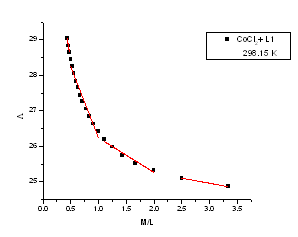 | Figure 4. The relation between molar conductance (M) and the molar ratio (M/L) of CoCl2 in presence of (L1) in absolute ethanol in 298.15 K |
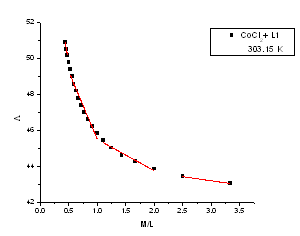 | Figure 5. The relation between molar conductance (M) and the molar ratio (M/L) of CoCl2 in presence of (L1) in absolute ethanol in 303.15 K |
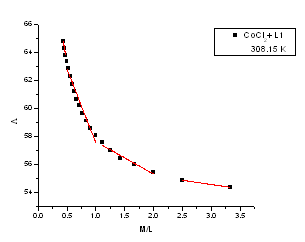 | Figure 6. The relation between molar conductance ( M) and the molar ratio (M/L) of CoCl2 in presence of (L1) in absolute ethanol in 308.15 K |
The limiting molar conductance (
₀) at infinite dilutions were estimated for CoCl2 in absolute ethanol alone(Fig. 1) and in the presence of the ligand (L 1)(Fig. 2) by extrapolating the relation between (
m) and Cm1/2 to zero concentration. By drawing the relation between molar conductance (
m) and the molar ratio of metal to ligand (M/L) concentrations (Fig. 3- 6), different lines are obtained with sharp breaks indicating the formation of 1:2, 1:1 and 2:1 (M : L) stoichiometric complexes. The experimental data of (
m) and (
₀) were analysed for the determination of association and formation constants for each type of the stoichiometric complexes.The association constants of CoCl2 in the presence of the ligand (L1) in absolute ethanol at 293.15, 298.15, 303.15 and 308.15 K for asymmetric electrolytes were calculated[9,10] by using equation: | (2) |
Where (
m,
₀) are the molar and limiting molar conductance, respectively of CoCl2; Cm is molar concentration of CoCl2, S(Z) is Fuoss-Shedlovsky factor, equal with unity for strong electrolytes[11]. The calculated association constants are shown in table 1.The Gibbs free energies of association (∆GA) were calculated from the association constant[12,13] by applying equation :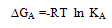 | (3) |
Where R is the gas constant (8.341 J) and T is the absolute temperatures (293.15, 298.15, 303.15 and 308.15 K). The calculated Gibbs free energies were presented in tables (1-4).The association free energies evaluated for CoCl2 – ligand complexes are small and spontaneous indicating electrostatic attraction. The formation constants (Kf) for CoCl2 complexes were calculated for each type of complexes (1:2), (1:1) and (2:1) (K:L) by using equatin[14,15]: | (4) |
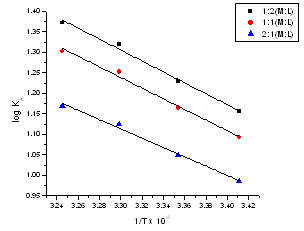 | Figure 7. The relation between log (KA) for (2:1), (1:1) and (1:2) stoichiometric complexes against 1/T |
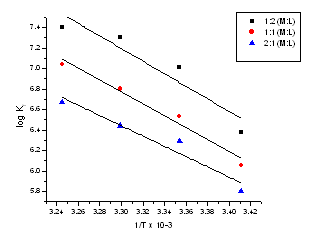 | Figure 8. The relation between log(Kf) for (2:1), (1:1) and (1:2) stoichiometric complexes against 1/T |
Where
m is the molar conductance of CoCl2 alone,
obs is the molar conductance of the solution during titration and
ML is the molar conductance of the complex.Table 1. Association constants and Gibbs free energies of association for CoCl2 with L1 in ethanol at 293.15 K 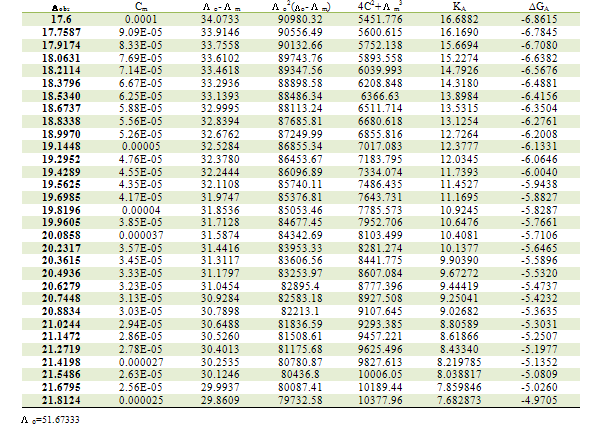 |
| |
|
Table 2. Association constants and Gibbs free energies of association for CoCl2 with L1 in ethanol at 298.15K 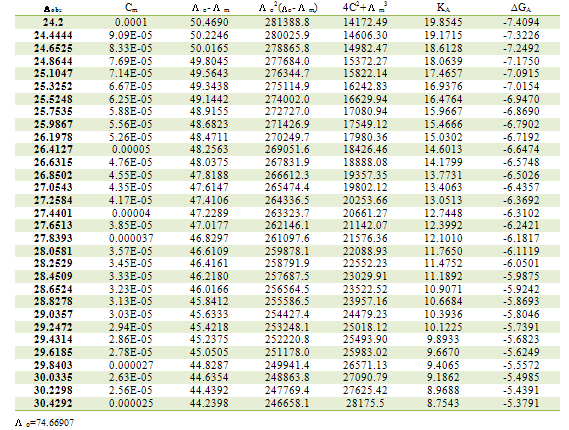 |
| |
|
Table 3. Association constants and Gibbs free energies of association for CoCl2 with L1 in ethanol at 303.15K 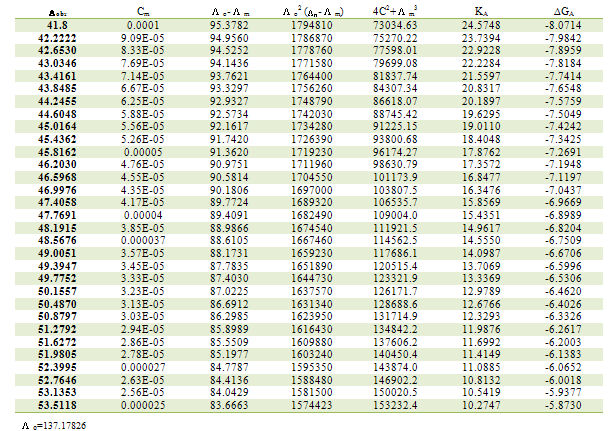 |
| |
|
Table 4. Association constants and Gibbs free energies of association for CoCl2 with L1 in ethanol at 308.15K 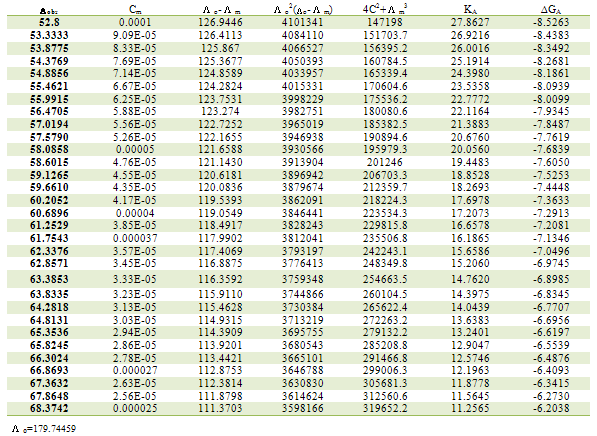 |
| |
|
Table 5. Formation constants and Gibbs free energies of formation for 1:2 (M/L) CoCl2-L1 complexes in ethanol at 293.15 K 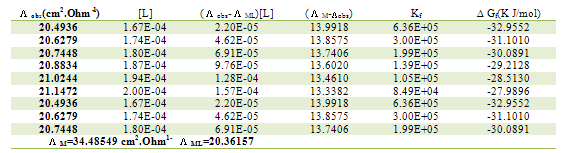 |
| |
|
Table 6. Formation constants and Gibbs free energies of formation for 1:1 (M/L) CoCl2-L1 complexes in ethanol at 293.15 K 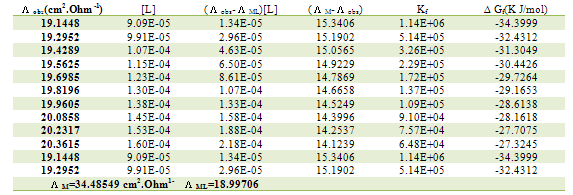 |
| |
|
Table 7. Formation constants and Gibbs free energies of formation for 2:1 (M/L) CoCl2-L1 complexes in ethanol at 293.15 K  |
| |
|
Table 8. Formation constants and Gibbs free energies of formation for 1:2 (M/L) CoCl2-L1 complexes in ethanol at 298.15 K 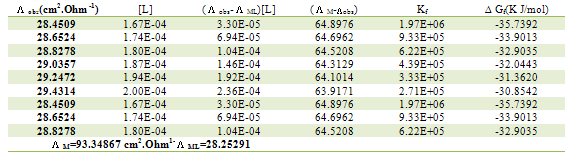 |
| |
|
Table 9. Formation constants and Gibbs free energies of formation for 1:1 (M/L) CoCl2-L1 complexes in ethanol at 298.15 K 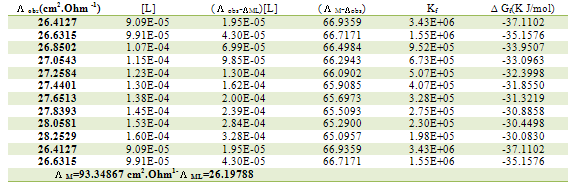 |
| |
|
Table 10. Formation constants and Gibbs free energies of formation for 2:1 (M/L) CoCl2-L1 complexes in ethanol at 298.15 K  |
| |
|
Table 11. Formation constants and Gibbs free energies of formation for 1:2 (M/L) CoCl2-L1 complexes in ethanol at 303.15 K 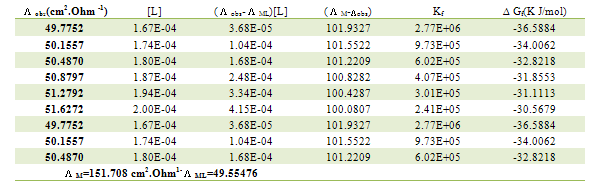 |
| |
|
Table 12. Formation constants and Gibbs free energies of formation for 1:1 (M/L) CoCl2-L1 complexes in ethanol at 303.15 K 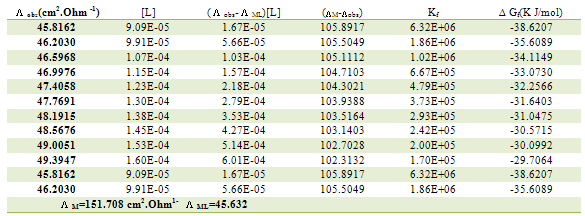 |
| |
|
Table 13. Formation constants and Gibbs free energies of formation for 2:1 (M/L) CoCl2-L1 complexes in ethanol at 303.15 K  |
| |
|
Table 14. Formation constants and Gibbs free energies of formation for 1:2 (M/L) CoCl2-L1 complexes in ethanol at 308.15 K 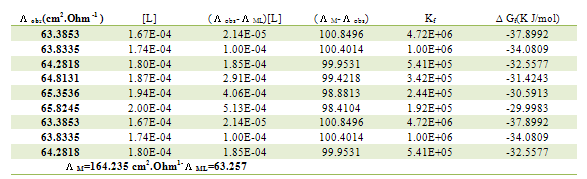 |
| |
|
Table 15. Formation constants and Gibbs free energies of formation for 1:1 (M/L) CoCl2-L1 complexes in ethanol at 308.15 K 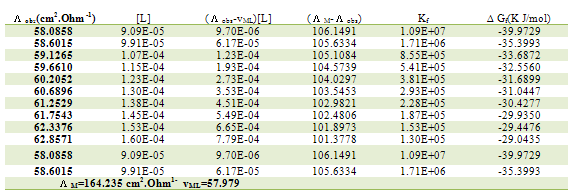 |
| |
|
Table 16. Formation constants and Gibbs free energies of formation for 2:1 (M/L) CoCl2-L1 complexes in ethanol at 308.15 K  |
| |
|
Table 17. The Entropy changes (T∆S) of CoCl2 in absolute ethanol at 293.15, 298.15, 303.15 and 308.15 K were calculated by applying the equation; ∆G = ∆H - T∆S 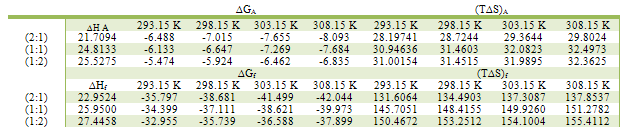 |
| |
|
The obtained values (Kf) for CoCL2-ligand stoichiometric complexes are presented in tables 2,3. The Gibbs free energies of formation for each stoichiometric complexes were calculated by using the equation:
The calculated ∆Gf values are presented in tables (5-16) .The enthalpy change(∆H) and the entropy change(T∆S) evaluated for CoCl2-ligand complexes indicate that (L1) may act as flexidentate ligand.The Enthalpy change of association (∆HA), and the Enthalpy change of formation (∆Hf) of CoCl2 in absolute ethanol at 293.15, 298.15, 303.15 and 308.15 K estimated by plotting log KA and log Kf for different M/L concentrations for (2:1), (1:1) and (1:2) stoichiometric complexes against 1/T are represented in(figs. 7,8).
4. Conclusions
This work concentrated on the behaviour of CoCl2 with the ligand conductometrically .The main target is to discuss the complexation between the metal and ligand for evaluating different concentrations from the metal ion in different solutions.
References
[1] | Robison, R.A. and Stokes, R.H.,(2002), “Electolyte solutions”, Butterworth &Co.(Publishers) Ltd, Newton Abbot,Devon. |
[2] | Barthel, J,M.G., Krienke, H.and Kunz,W., (1998),”Physical chemistry of electrlolyte solutions”, Springer - Verlag, Darmstadt, new York. |
[3] | Trevor M.Letcher,(2007),” Developments an applications in solubility”., The Royal Society of Chemistry,Cambridge. |
[4] | Chaudhary, A., Phor, A., and Singh, R.V. (2005) Bioinorganic Chemistry and Applications 3, 161. |
[5] | Prasad, S. and Agarwal, R.K., (2007) Transition Metal Chemistry 32, 143. |
[6] | Kumar, M., Qiu, D., Spiro, T.G. and Regsdale, S.W. (1995) Science 270, 628. |
[7] | Kyritsis, P., Dennison. C., Kalverda, A.P., Canters, G.W. and Sykes, A.G. (1994)J. Chem. Soc. Dalton Trans. 3017. |
[8] | Gryzybkowski, W. and Pastewski, R. (1980) Electrochimica Acta 25, 279. |
[9] | El-Shishtawi, N.A, Hamada, M.A. and Gomaa, E.A.(2010)J. Chem. Eng. Data 55, 5422. |
[10] | Hamada, M.A., El-Sheshtawi, N.A. and Gomaa, E.A. (2009) South. Braz. J.Chem. 17, 33. |
[11] | Gomaa, E.A. (1987), Thermochimica Acta 120, 183. |
[12] | Gomaa, E.A. (1988), Thermochimica Acta 128, 99. |
[13] | El-Dousski, F.I. (2008) Journal of molecular Liquids 142, 53. |
[14] | Takeda, Y. (1983), Bul. Chem. Soc. Jpn 56, 3600. |
[15] | Rahmi-Nasrabadi, M., Ahmedi, F., Pourmor-tazari, S.M., Ganjal, M.R. and Alizadeh, K. (2009) Journal of Molecular Liquids 144, 97. |