L. C. Nehru, C. Sanjeeviraja
School of Physics, Alagappa University, Karaikudi, 630 004, India
Correspondence to: C. Sanjeeviraja, School of Physics, Alagappa University, Karaikudi, 630 004, India.
Email: |  |
Copyright © 2012 Scientific & Academic Publishing. All Rights Reserved.
Abstract
A facile and rapid microwave-assisted solution combustion method was developed to synthesis to produce nanocrystalline Zn2SnO4 powder using dissolution of zinc and tin nitrate (as the oxidant) and glycine (as fuel) as the starting materials and water as solvent, then heating the resulting solution in a microwave oven. The study suggested that application of microwave heating to produce the homogeneous porous Zn2SnO4 was achieved in a few minutes. The structure and morphology of the as-prepared combustion products were investigated by means of XRD and SEM. Fron the XRD results, confirmed the formation of face-centered cubic spinel structural and the SEM results indicated the spherical surface characteristic of the products. The as-prepared powders have larger band gap energy (3.5 eV) and are described here.
Keywords:
Nanoparticles, Zinc Stannate, Microwave-Assisted Combustion Synthesis, Optical Studies
Cite this paper: L. C. Nehru, C. Sanjeeviraja, Microwave-assisted Solution Combustion Synthesis of Nanostructured Zn2SnO4, Nanoscience and Nanotechnology, Vol. 3 No. 1, 2013, pp. 10-13. doi: 10.5923/j.nn.20130301.02.
1. Introduction
Considerable developments have been witnessed in many fields due to the implication of nanotechnology and also due to the deployment of novel materials with desired properties. The nanosized mixed metal oxides have much interest in cutting edge technologies due to their size and shape dependent properties, which are tuneable by varying the experimental parameters. In the recent years, a fabrication of transition conducting oxides (TCO) with nano structure has been the target of scientific interests because of their unique properties and fascinating applications in optoelectronic, devices and biomedical science. Among these zinc tin oxide (ZTO) is sometimes referred to as zinc stannate, has recently received attention as an alternative TCO materials and has attracted considerable interest in many areas of chemistry, physics and materials science. It drawn extra attention due to their broad range of applications in sensors[1], solar cells[2], transparent conductive oxides[3] and optoelectronic devices[4]. So the researchers are much effort continues to focus on investigating them for technological applications due to their unusual physical and chemical properties, which differ significantly from those of conventional bulk materials from the stand point of their extremely small size or large specific surface area. Generally nanoscale particles possess different physical, chemical properties, better sinter ability, larger catalytic activity and other unique properties may be expected because of their nano-sized particle are high surface area with different surface defect properties etc. So the technological importance of zinc stannate (Zn2SnO4) has motivated several studies on the synthesis of this material using various methods [5-12]. Few research accomplishments have been reported on the synthesis of zinc stannate by various synthesis methods. However there is no attempt has been made to study the Zn2SnO4 by microwave-assisted combustion synthesis (MACS) method to the best of our knowledge. Among the synthesis approaches MACS method is considered to be one of the best techniques to prepare nanomaterials because of its short reaction time, high purity and yield, better homogeneity, high surface area and producing small particle size in a single step. The aim of this study is to prepare Zn2SnO4 nanoparticles by microwave-assisted combustion method, explore its structure and electrical properties.
2. Experimental
All the chemicals and reagents used in the experiments were analytically pure and were purchased from MERCK Company, and were used as received without further purification. For the preparation of Zn2SnO4 nanoparticles, zinc nitrate, tin nitrate and glycine was used as starting materials. Deionized water was used for preparing solutions. Zn2SnO4 nanoparticles were prepared by MACS method, containing stoichiometric amount of corresponding metal nitrate and a suitable fuel. The stoichiometric composition of solution components (oxidizer to fuel) was calculated according to the principle of propellant chemistry. The stoichiometric amounts (2:1) of zinc nitrate and tin nitrate (oxidizer) were dissolved in a minimum amount of deionized water to get clear solution. Then, glycine was added in this solution and poured into a quartz container and could be mixed well by magnetic stirring for 1 h, which made them almost as homogeneous mixtures, which was placed in a domestic microwave-oven. Initially, the solution boils and undergoes dehydration followed by decomposition with the evolution of large amount of gases with white fumes occurs coming out from the exhaust opening provided on the top of the micro oven. After the solution reaches the point of spontaneous combustion, it begins burning and releases lots of heat, vaporizes all the solution instantly and becomes a foamy white solid powder. The synthesized Zn2SnO4 powders was identified the phase formation, structural and crystallite size estimation by X-ray diffraction method using a X-Pert PRO PANalytical diffractometer using nickel filtered Cu-Kα radiation (λ=0.15418 nm) as source and operated at 40 kV and 30 mA. The sample was scanned in the 2θ ranging from 10 to 80° in θ-θ scan mode. The observed peak positions were compared with the standard JCPDS data and Miller indices were assigned to the Bragg peaks. SEM micrographs of samples were obtained using Hitachi S4800 scanning electron microscope. Infrared spectra were recorded using Nicolet Avatar 360 FTIR spectrometer with KBr pellets and UV–Vis absorption spectra were recorded using Shimadzu UV-2550 spectrophotometer.
3. Results and Discussion
The structure of prepared Zn2SnO4 nanocrystallites has been investigated by XRD (Fig. 1) pattern of glycine as fuel - lean, stoichiometric and fuel - rich conditions. Zn2SnO4 belongs to the inverse spinel structure where the tetrahedral voids are occupied by the Zn atoms and the octahedral voids are occupied by equal numbers of Zn and Sn atoms. The reaction of the components completes and transform to a face-centered cubic spinel structural of fuel stoichiometric and fuel rich conditions. 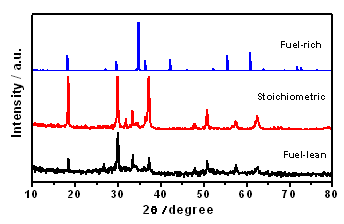 | Figure 1. The XRD patterns of glycine used fuel - lean, stoichiometric and fuel - rich conditions of Zn2SnO4 |
From this conditions, PXRD clearly depicts the peaks corresponding to the (1 1 1), (2 2 0), (3 1 1), (2 2 2), (4 0 0), (4 2 2), (5 1 1), (4 4 0), (5 3 1), (5 3 3), (6 2 2) and (4 4 4) Miller planes, which suggest the face-centered cubic spinel structural of Zn2SnO4 as per the JCPDS (File no. 74-2184). The lattice constant “a” was calculated using multiple peaks by least squares method which is consistent with the standard value. No impurities are detected from this pattern, indicating that the products are pure and high crystalline spinel oxide. This indicates that pure Zn2SnO4 was obtained under the present synthesis method. In addition, it is shown that the microwave-assisted solution combustion method is complete, and the reactionis accord with the proper proportion to react. It is also proved that microwave-assisted solution combustion method has excellent advantages toobtain high crystalline powders with narrow grain size-distribution and high purity without any treatment at high temperature. Figure 2 shows the SEM micrographs of glycine as fuel - lean, stoichiometric and fuel - rich conditions. The product is foamy and porous in nature formed by the escaping gases during the combustion process. The particle size of Zn2SnO4 was observed in stoichiometric condition, it is in nanometer scale compared to the other conditions. 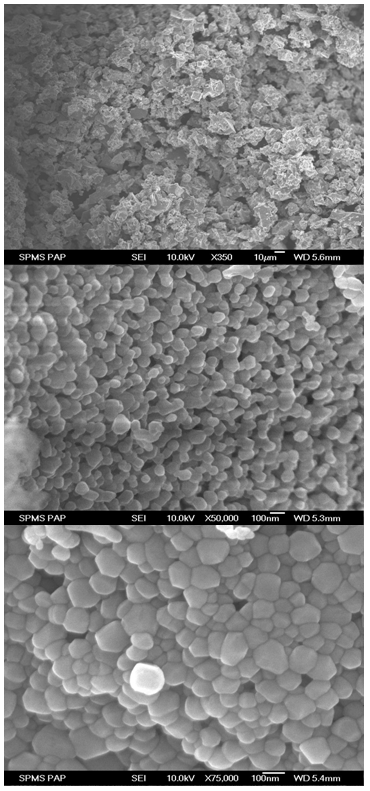 | Figure 2. SEM micrograph of fuel in lean, stoichiometric and rich condition of Zn2SnO4 powders |
Figure 3 shows the FTIR spectrum of the stoichiometric condition of Zn2SnO4, a broad absorption peak at ~572 and 555cm-1 is due to symmetric stretching vibration of ZnO and SnO2 groups respectively and this band could be assigned to the Sn-O-Zn bonding of the Zn2SnO4 inverse spinel. A absorption band at ~1630 and 3500 cm-1 indicates the presence of hydrogen bonds and absorption at ~1429 and 2350 cm−1 were assigned to C–H vibration modes attributed to the organic trace residuals. The peak at ~1740 cm-1 band is the possible bonding of Zn in ZnO and ~1219 cm-1 is attributed to Sn–O stretching vibrations group. The small absorption at ~1000 cm-1 is due to the deformation vibration of C=O. 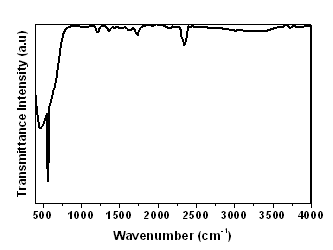 | Figure 3. FTIR spectra of Zn2SnO4 |
Diffuse reflectance spectroscopy is a useful tool to reveal the energy structures and optical properties of semiconductor nanocrystal, and the migration of the light-induced electrons and holes are the key factors to control photocatalytic reaction, which is related to the electronic structure characteristics of the materials. Fig. 4 shows the UV–visible diffuse reflectance spectrum of stoichiometric condition of Zn2SnO4 powders. It can be seen that the octahedron Zn2SnO4 microstructure has a steep absorption edge in visible range, indicating that the absorption relevant to the band gap is due to the intrinsic transition of the semiconductors rather than the transition from impurity levels. According to the equation α=2.303Aρ/IC where A is the absorbance of a sample; ρ is the density of Zn2SnO4 powder, C is the concentration of the particles; and I is the optical path length. The optical absorption coefficient (α) of a semiconductor is close to the band edge which is estimated from the Tauc's relationship (αhυ)2=A(hυ-Eg) where α is the absorption coefficient, h is the Planck's constant, υ is the frequency of the incident photon, Eg is the optical energy direct band gap and A is a constant. The exact value of the band gap is calculated by extrapolating the straight line portion of the (αhυ)2 versus hυ axis. Plotting (αhυ)2 as a function of photon energy and extrapolating the linear portion of the curve to the photon energy axis (absorption equal to zero) gives an absorption edge energy corresponding to Eg = 3.5 eV. This value accords with the value of bulk Zn2SnO4 (3.6 eV) as shown in insite of Fig. 4.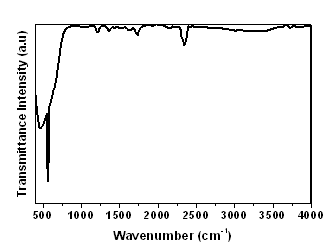 | Figure 4. UV–Vis spectra of Zn2SnO4 |
4. Conclusions
The spherical shape Zn2SnO4 powders are successfully synthesized by microwave-assisted combustion reaction by using glycine as a fuel. The structural parameters calculated from XRD pattern confirm Zn2SnO4 nano crystalline possessing face-centered cubic spinel structure. Nanocrystalline Zn2SnO4 powder was confirmed for the stoichiometric condition. SEM micrograph revealed the nano particles in the order of 50 to 60 nm, which was estimated from the gaussian distribution of particles graph. The chemical structure of the Zn2SnO4 powders was investigated by FTIR. This method presents a way for the controlled synthesis of multicomponent metal oxides.
ACKNOWLEDGEMENTS
One of the authors, Dr. L. C. Nehru gratefully acknowledges Council of Scientific and Industrial Research, India for the award of Research Associateship to carry out this research work at School of Physics, Alagappa University, Karaikudi – 630 004, INDIA.
References
[1] | Zhen-Hua Liang, Ying-Jie Zhu, Guo-Feng Cheng, Yue-Hong Huang, J Mater Sci , 42 (2007) 477. |
[2] | K. Matsubara, P. Fons, K. Iwata, A. Yamada, K. Sakurai, H. Tampo and S. Niki, Thin Solid Films, 431 (2003) 369. |
[3] | T. Minami, Materials Research Bulletin, 25 (8) (2000) 38. |
[4] | CJ. Lee, TJ. Lee, SC. Lyu, Y. Zhang, H. Ruh, HJ. Lee, Appl Phys Lett , 81 (2002) 3648. |
[5] | X.Y. Xue, Y.J. Chen, Y.G. Wang, T.H. Wang, Appl. Phys. Lett. 86 (2005) 233101. |
[6] | J. Xu, X. Jia, X. Lou, J. Shen, Solid-State Electron. 50 (2006) 504. |
[7] | X.Y. Xue, Y.J. Chen, Q.H. Li, Phys. Lett. 88 (2006) 182102. |
[8] | X.Y. Xue, P. Feng, Y.G. Wang, T.H. Wang, Appl. Phys. Lett. 91 (2007) 022111. |
[9] | Y. Inaguma, M. Yoshida, T. Katsumata, J. Am. Chem. Soc. 130 (2008) 6704. |
[10] | Ivetic, Z. Vukovic, M.V. Nikolic, V.B Pavlovic, J.R Nikolic, D. Minic M. M. Ristic Ceramics International 34 (2008) 639. |
[11] | Zhijun Yang, Linlin Lv, Yali Dai, Zhihui Xv, Dong Qian, Applied Surface Science 256 (2010) 2898. |
[12] | Huiyang Gou, Faming Gao, Jingwu Zhang, Computational Materials Science 49 (2010) 552. |