T. Agarwal 1, K. Gupta 2, M. G. H. Zaidi 1, S. Alam 3
1Department of Chemistry, G.B.Pant University of Agriculture & Technology Pantnagar, Uttarakhand, 263145, India
2Department of Chemistry, Hindu PG College, Moradabad, 244001, India
3Polymer Division, Defense Materials Stores Research Development and Establishment, G.T. Road Kanpur, 208013, India
Correspondence to: M. G. H. Zaidi , Department of Chemistry, G.B.Pant University of Agriculture & Technology Pantnagar, Uttarakhand, 263145, India.
Email: |  |
Copyright © 2012 Scientific & Academic Publishing. All Rights Reserved.
Abstract
A microwave (MW) assisted green process to synthesize nanomagnetic polymer composites (NPCs) based on polyacrylonitrile (PAN) has been developed. The process involves 2,2-azobisisobutyronitrile initiated in-situ polymerization of acrylonitrile in presence of tween -20 stabilized ferrite nanoparticles (FNPs) under MW irradiation ranging 25 to 100 Watt over 10min. With MW power, the polymerization reaction has been progressed resulting in NPCs with enhanced rheoviscosity and yield over PAN. The formation of NPCs has been ascertained through Fourier transformed infra-red spectra, X ray diffraction and atomic force microscopy .Vibrating sample magnetometry and simultaneous thermogravimetric-differential thermal analysis-differential thermogravimetry reveals the formation of thermally stable NPCs with saturation magnetization 70.0 emu/g.
Keywords:
Microwave Synthesis, Polymer Nanocomposites, Spectra, Microscopy, Magnetometry, Thermal Analysis
1. Introduction
Nanomagnetic polymer composites (NPCs) based on polyacrylonitrile (PAN) and their copolymers offers wide spectrum of applications for dielectric ,microwave absorbing materials, data storage devices , sensor and biomedical applications[1-2]. Over past few years there is critical need for improved sustainability in the nanocomposite manufacturing through the development of green chemical approach. In this context, the significance of environmentally benign methods based on radiations and supercritical fluids as has recently been of increasing technological interest[3-4] .The physical characteristics of NPCs can be tailored through dispersing the FNPs into the polymer matrix either through reactive or non reactive routes in aqueous and organic phases. In the reactive process, the presence of FNPs induces acceleration of the free radical polymerization reactions under MW irradiation[5]. Reactive synthesis of NPCs has been conducted in presence of FNPs through in-situ polymerization[6].On the contrary, the non-reactive route involves the direct infusion of FNPs into the polymer matrix swollen in organic solvents. Literature survey reveals that NPCs based on PAN were synthesized through electrospinning of PAN in presence of FNPs in organic solvents[1,7-8]; no efforts are made to synthesize the proposed class of NPCs through MW assisted in-situ polymerization process.
2. Experimental
Acrylonitrile (sd Fine, Chemicals, ) was purified through distillation under reduced pressure. AIBN () and other solvents were used without further purification. FNPs were prepared according to the method reported earlier[9]. FNPs (5.0 phr) were dispersed in T -20 (0.6mL) in a glass viol (10mL) under vortex over 25 min. The composition was further treated with AN (15.17X10-3 mol) and AIBN (0.61 X10-3 mol), followed by vortex for 2 min. The contents were subjected to MW irradiation at 25W over 10 min. The synthesized NPCs was isolated and washed with methanol. PAN was synthesized under identical reaction conditions in the absence of FNPs and used as a control.
2.1. Measurements
FT-IR (KBr) spectra of samples were recorded on Galaxy 300 Mattson FT-IR spectrometer. Rheoviscosity were recoded at 27±1℃ over Nach Hoppler Viscometer at @ 1mg/mL in DMF.XRD pattern of samples was recorded at 25℃ over Rigaku-Geigerflex X-Ray diffractometer using Cu-Kα radiation (λ= 0.154056nm) in the 2θ range of 20º-70º at 40 KV and 20 mA with step size 0.019o. The crystallite size was evaluated through Debye-Scherrer equation. TEM images were recorded over JEOL 1011 at 80 kV. AFM images were recorded over NTEGRA Prima microscope under tapping mode using ultra sharp Si cantilevers having force constant of 48 N/m. VSM data were recorded over Princeton EG&G model 155 with maximum current 22.5 Ampere, scan time-900 seconds, VSM ranging 10 KOe to -10 K Oe at room temperature. Ferrite content of NPCs was deduced according to the relation % ferrite content =Ms of NPCs/Ms of FNPs X100, where Ms is saturation magnetization. Simultaneous TG-DTA-DTG was recorded over Perkin Elmer Pyris Diamond TA in air at the flow rate of 200mL/min up to 700℃/ 10℃/ min using alumina[9].
3. Result and Discussion
MW assisted polymerization of AN monomer in presence of AIBN and a ferrofluid comprising FNPs (5.0 phr) stabilized into tween-20 has afforded Polyacrylonitrile ferrite nanocomposites (NPCs). The process of polymerization has been executed under MW irradiation ranging 25 to 100 W over 10min. In order to have results to be comparable, the NPCs was synthesized under the conditions similar to the polymerization reactions of AN. In general, with MW power, the polymerization of AN was progressed resulting in PAN with %Y ranging 13.65-9.93 and corresponding η (mPa.sX102) ranging 0.79-0.10With MW power, a regular decrease in η and %Y of PAN and respective NPCs has been observed. In general, such decrease in η and %Y has been higher in PAN over respective NPCs. Polymerization of AN in presence of FNPs (5 phr) was progressed over 10 min resulting in NPCs with η ranging 1.25-1.02. With MW power, polymerization of AN in presence of FNPs (50 mg) was progressed over 10 min resulting in NPCs with %Y ranging 71.66-21.55. Above 25W, the low η and %Y may be due to the MW assisted degradation of the polymers from the NPCs[10]. 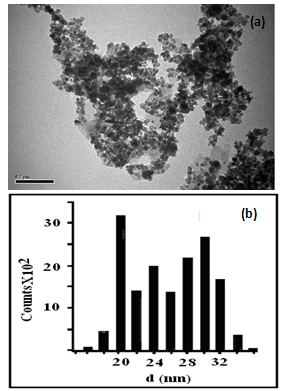 | Figure 1. Transmission electron micrograph (a) and particle size distribution (b) of FNPs |
FNPs has revealed characteristic FT-IR absorptions at 3443.47(νO-H), 2356.36 (νCO2, atmospheric), 1739.02 (νC=O), 1621.64 (δ O-H), and 583.26 (Fe-O, M-Otetra) 434.78-418.53 (Fe-O, M-Oocta).PAN has revealed a broad band at 3473.64 (ν O-H), 2939.76 (ν asym., CH2), 2865.02(ν sym, CH2), 2365.06 (νCO2, atmospheric), 2244.22 (ν C≡N), 1681.46 (δ O-H), 1454.57 (δ CH2) and 1227.08-1074.58 (C-N) due to the cyclization of nitrile groups. The wave number appeared at 578.25 corresponds to the presence of Fe-O in NPCs. The TEM images reveal agglomeration of FNPs (Figure.1a) with maximum counts corresponding to the particle size of 20 nm (Figure.1b).AFM of PAN and respective NPCs are shown at 2µm (Figure 2).At the indenter height of 140 nm, PAN shows average roughness of 19.56 nm with a matrix comprising non-spherical particles with grain size of 71.36 nm (Figure.2a). At the indenter height of 160 nm, the NPCs shows matrix with average roughness of 24.88 nm with nearly spherical grains comprising particle size 82.35 nm (Figure.2b).Such increase in the average roughness and grain size of NPCs over PAN indicates may be attributed to the presence of FNPs. 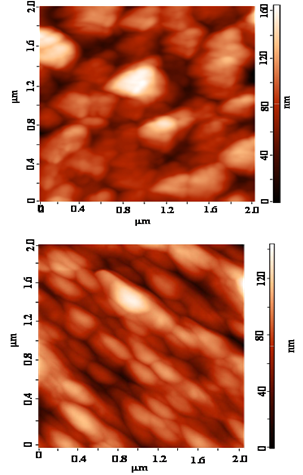 | Figure 2. AFM image of PAN (a) and NPCs (b) |
FNPs have revealed diffraction peaks indexed at 2θ=30.17, 35.50, 43.30, 57.16 and 62.77 with corresponding hkl values (220), (311), (400), (422 and 511) and (440) respectively. The experimental basal spacing results were in close agreement with ASTM data of Fe3O4. Debye-Scherrer equation has provided the particle size of FNPs ~18.20 nm (Figure.3a)[9]. The crystalline nature of PAN has been ascertained through XRD pattern with characteristic intense peaks at (2θ) 16.66, 19.22 and 29.33 (Figure 3 b)[11]. NPCs have also revealed the characteristic peak at 2θ=16.89 due to crystalline nature of PAN. The dispersion of FNPs into PAN matrix has been ascertained through a diffraction peak at (2θ) 35.50 (Figure.3c).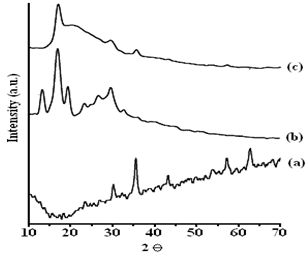 | Figure 3. XRD Spectra of FNPs (a), PAN (b) and NPCs (c) |
Figure 4 shows hysteresis curves of FNPs and NPCs. FNPs has reveals saturation magnetization (Ms) 70.0 emu/g (Figure.4a), whereas, in case of NPCs, the Ms has been decreased to 3.14 emu/g due to the surface effects caused by the entrapment of FNPs into the PAN matrix (Figure.4b). Calculations based on hysteresis data reveals 4.49%ferrite content of NPCs[12].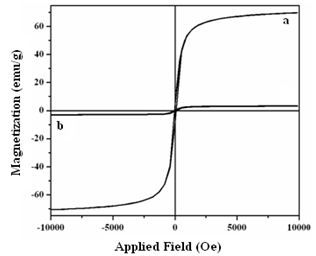 | Figure 4. Hysteresis curves of FNPs (a) and NPCs (b) |
The TGA curve of FNPs shows that the total weight loss is about 9% over the entire temperature range. A dramatic weight loss was observed between temperature ranging 200-295℃. This might be due to desorption of physicochemically bound water and solvents (Figure.5a). The first step decomposition of PAN was appeared at 224℃; it may be ascribed to the cyclization reaction of monomer during polymerization. The second step decomposition was appeared at 500℃ and it leveled off at 650- 700℃. The DTG curve peaked at 337℃ with highest rate of decomposition 0.74 mg/min. A remarkable exotherm at temperature ranging 270℃ (67.2 μV) to 424℃ (69.8 μV) with heat of degradation (-ΔH); 3490 mJ/mg was also reported. TGA of NPCs is marked by a late thermal degradation over its polymer (Figure.5b). 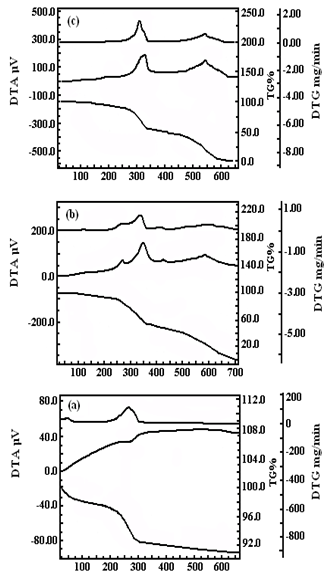 | Figure 5. TG-DTA-DTG of FNPs (a), PAN (b) and NPCs (c) |
The decomposition temperature of NPCs is shifted at 275℃. Prior to this temperature, 14.2% WL was recorded. The second step decomposition was reported at 500℃ and it was terminated at 657℃. The couple of the weight loss was assigned to the degradation of polymer chains. No significant weight loss was reported beyond 600℃. DTG peak shows highest rate of decomposition (1.52mg/min) at 308℃ followed by 0.66mg/min at 546℃. A DTA exotherm was reported at temperature ranging 316℃ (174.9μV) to 327℃ (184.2 μV) with the (-ΔH); 2550 mJ/mg. Another DTA exotherm was appeared at 547℃ (154.3 μV) with (-ΔH); 4310 mJ/mg (Figure.5c).
4. Conclusions
Present study describes the green synthesis of nanomagnetic polymer composites (NPCs) with enhanced thermal stability and saturation magnetization 70 emu/g through polymerization of acrylonitrile in T-20 stabilized ferrofluid under microwave irradiation ranging 25-100W. For this purpose ferrite nanoparticles (FNPs) with crystallite size ~18.20 nm were prepared using Fe (II) chloride as precursor. The formation of NPCs has been ascertained through spectra and microscopy.
ACKNOWLEDGEMENTS
The financial assistance granted by Department of Biotechnology, Government of India is duly acknowledged. The Authors are also grateful to Instrumentation Center, IIT Roorkee, India.
References
[1] | J. Guo, X. Ye, W. Liu, Q.Wu, H. Shen, K. Shu, “Preparation and characterization of poly(acrylonitrile-co-acrylic acid) nanofibrous composites with Fe3O4 magnetic nanoparticles,” Materials Letters, vol. 63, pp.1326-1328,2009. |
[2] | .L. Guo, G. Liu, R.Y. Hong, H.Z. Li, “Preparation and characterization of chitosan poly(acrylic acid) magnetic microspheres,” Marine Drugs ,vol. 8,pp. 2212-2222,2010. |
[3] | T.Erdmenger, C. R. Becer, R. Hoogenboom, U. S. Schubert, “Simplifying the free-radical polymerization of styrene: microwave-assisted high-temperature auto polymerizations,” Australian Journal of Chemistry, vol. 62, pp.58–63, 2009. |
[4] | K.Kempe, C.R.Becer, U.S. Schubert, “Microwave-Assisted Polymerizations: Recent Status and Future Perspectives,” Macromolecules, vol.44, pp. 5825–5842, 2011. |
[5] | M.Klink, U.Kolb, H. Ritter, “Microwave-assisted synthesis of channel-containing polymeric materials,” e-Polymers, vol. 069, pp. 1-6, 2005. |
[6] | F. Lan, K.X. Liu, W. Jiang, X.B. Zeng, Y.Wu, Z.W. Gu, “Facile synthesis of monodisperse superparamagnetic Fe3O4/PMMA composite nanospheres with high magnetization,” Nanotechnology, DOI: 10.1088/0957-4484/22/22/ 225604.2011. |
[7] | I.H.Chen, C. C. Wang, C.Y. Chen, “Fabrication and characterization of magnetic cobalt ferrite/ polyacrylonitrile and cobalt ferrite/carbon nanofibers by electrospinning,” Carbon, vol. 48, pp.604 –611, 2010. |
[8] | S.Cavaliere, V. Salles, A. Brioude, P.Monod, D. Cornu, P. Miele, “Elaboration and characterization of magnetic nanocomposite fibers by electrospinning,” Journal of Nanoparticle Research, vol. 12, pp.2735–2740, 2010. |
[9] | M. G. H. Zaidi, D.Sharma, V.Agarwal, S.Alam, A.K. Rai, R.P.Pant, “Synthesis of polyvinylpyridine ferrite nanocomposites in supercritical carbon dioxide,” Journal of Nanostructured Polymers Nanocomposites, vol. 6, pp.103-109, 2010. |
[10] | V.Singh, A. Tiwari, P. Kumari, A.K.Sharma, “Microwave accelerated synthesis and characterization ofpoly(acrylamide),” Journal of Applied Polymer Science, vol. 104, pp. 3702-3707,2007. |
[11] | J.S. Kim, H.J. Jeon, K.M. Lee, I.J.N. Im, J.H. Youk, “Dispersion polymerization of acrylonitrile using a poly (ethylene glycol)-b-polyacrylonitrile macro-RAFT agent,” Fibers and Polymers, vol. 11, pp.153-157, 2010. |
[12] | D.Zhang, A.B. Karki, D. Rutman, D.P.Young, A. Wang, D. Cocke, T.H. Ho , Z. Guo, “Electrospun polyacrylonitrile nanocomposite fibers reinforced with Fe3O4 nanoparticles: Fabrication and property analysis,” Polymer, vol 50, pp. 4189–4198,2009. |