Osaretin Albert Taiwo Ebuehi , Nosarieme Omoregie Abey
Department of Biochemistry, University of Lagos, Lagos, Nigeria
Correspondence to: Osaretin Albert Taiwo Ebuehi , Department of Biochemistry, University of Lagos, Lagos, Nigeria.
Email: |  |
Copyright © 2016 Scientific & Academic Publishing. All Rights Reserved.
This work is licensed under the Creative Commons Attribution International License (CC BY).
http://creativecommons.org/licenses/by/4.0/

Abstract
Background: Cannabis sativa is the most commonly abused drug in the world; its active ingredient tetrahydrocannabinol (THC) is linked to cognitive deficits. The underlying neurochemical change is just being explored. Objective: This study was set out to decipher some structural and chemical changes that underlies the cognitive Function displayed by Cannabis sativa administered rat. Methodology: Sprague-Dawley rats were fed diet containing 10% and 5% Cannabis sativa chow for a period of seven weeks served as model to evaluate the learning, memory and cognitive function, acetylcholine level/acetylcholinesterase activity, serotonin concentration, antioxidant capacity and histopathology of the brain. Results: The results indicate that Cannabis sativa administered rats exhibited cognitive deficit and impaired learning, decreased acetylcholine level, acetylcholinesterase activity, serotonin concentration and altered structural component of the brain all in dose and time dependent manner. Conclusions: Data of the study suggest that chronic Cannabis sativa users are at higher risk of cognitive deficit and memory impairment. The imbalance in the antioxidant, serotoninergic and cholinergic systems as observed in the study might correlate to the cognitive deficit and structural alteration of the rat’s brain.
Keywords:
Cognitive function, Cannabis sativa, Anti-oxidants, Serotoninergic, Serotoninergic and cholinergic systems, Cognitive function, Rat
Cite this paper: Osaretin Albert Taiwo Ebuehi , Nosarieme Omoregie Abey , Impact of Marijuana (Cannabis sativa) on Some Neurochemicals and Cognitive Function of Sprague-Dawley Rats, Research in Neuroscience , Vol. 5 No. 1, 2016, pp. 1-9. doi: 10.5923/j.neuroscience.20160501.01.
1. Introduction
Marijuana (Cannabis sativa) is the illicit substance commonly used in most Western societies [1]. In recent years, there has been noticeable increase in cannabis and its products consumption among teenagers and young adults [2], Marijuana is highly neuroactive. While cannabis is generally consumed for its psychotropic effects, its main psychoactive ingredient, Δ9-tetrahydrocannabinol (Δ9- THC), also affects a number of other neuronal systems, including those involved in motor control, sensorimotor learning and memory filter mechanisms [3]. Studies in humans and animals indicate that THC and other marijuana-related cannabinoids interfere with the brain’s chemical balance by acting on cannabinoid receptors which are found on neurons in many places in the brain [4]. Learning involves the brain processing information in complex networks of nerve cells. The cells communicate and excite one another through special connections, called synapses. Investigations have shown that THC suppresses neurons in the information-processing system of the hippocampus; most notably the two areas of motor control and memory are where the effects of cannabis are directly and irrefutably evident [5]. This study is set out to correlate Cannabis sativa use and underlying neurochemical changes that result in cognitive deficit, with emphasis on: serotoninergic system, acetylcholine and its enzyme activities, antioxidants capacity and histopathological examination of the exposed rat brain.
2. Materials and Methods
Twenty-one (21) male Sprague-Dawley rats were obtained from the Laboratory Animal Centre of the College of Medicine of the University of Lagos, Nigeria. The rats were handled in conformity with International Ethical Institutional guidelines for animal experimentation [6]. The rats were housed in groups in clean capacious metallic cages under standard laboratory conditions including good aerated room, good lighting, with suitable temperature (28°C ± 2°C) in a neat environment and at a 12-hour light/dark cycle. The rats were divided into 3 groups, Seven (7) per cage and acclimatized for two weeks, where they had access to standard rat chow and water ad libitum.Feed FormulationThe marijuana leaves were obtained and authenticated by the Department of Botany, University of Lagos, Lagos. The dried leaves were ground into powdered form and then used for the feed formulation of diet by blending with other rat chow components and pelletized. Three different diets were formulated; Diet1 (10kg) contains all rat chow components and 1kg of Cannabis sativa (comprising 10% of the entire feed), Diet 2 contains all rat chow components and 0.5kg (forming 5% cannabis diet). Cannabis sativa. Diet 3 contains all rat chow ingredients and no (0%) Cannabis sativa was added. Zero percent Cannabis diet was fed to control while 10% was fed to group 1, diet 2 fed to group 2 of test group. Body Weight The body weight and feed intake of rats were measured every other day for 7 weeks.Cognitive Function TestThe training apparatus of rats used for the study to assess short-term and long-term memory was the modified Shuttle box [6]. It consist of two wooden compartments of identical dimensions (28cm by 15cm). The two compartments were separated by a door in the middle part of the apparatus. of the two compartments, one was illuminated and the other was dark [6]. The door could be raised to permit entry of the rats into any of the two compartments. The floor consisted of 6mm diameter wire rods connected to a step down transformer with a regular dimmer which could be switched on and off to deliver an instant scrambled foot shock to the supposed compartment. The training task commenced after rats were fed with cannabis diet. The first training started on the fifth day of administration. All rats in each group were placed in the shuttle box and had access to the light or dark compartment for 1h. On day 2 of training, rats from each group were placed in the illuminated compartment and then, 30 sec later the door was raised. The dark compartment indicated a "safer" compartment in which the rat would not receive foot shock. To avoid foot shock and the light compartment, the rat had to cross into the safer compartment within 5s. Failing to do so resulted in foot shock. The inter-trial intervals were randomized at 30, 45, and 60s. For each rat, the daily training session consisted of 2 trials. Such training trials were conducted for about 5 days. The measure of acquisition was the number of avoidance responses per day and the number of rat making about 70% avoidances in a block of 2 trials. The learning procedure was repeated on days 3, 4 and 5. For short-term testing, 24 h after training (day 2), each rat from the 2 groups was placed in illuminated chamber and 30 sec later the door was raised, and the time spent in the light compartment before entering the dark compartment was recorded. Learning skills acquired on days 5 depicted long-term memory [6].Sample PreparationAt the end of the seven weeks exposure, rats from each group were sacrificed by decapitation. Brains were excised, wiped dry of blood, weighed and thoroughly perfused in ice-cold phosphate buffered saline, pH 7.4, until further analysis, while the brain sample for histopathology was stored in formalin. One gram of each rat brain is homogenized with 5ml of ice cold 0.05M phosphates buffer pH 7.0. The homogenate was centrifuged at 3000g for 15min and the supernatant obtained was stored for further analysis [7]. This process was repeated for the remaining rat in each group after seven weeks of feeding.Determination of Acetylcholine concentration and acetylcholine esterase activity in Brain The assay was carried out using the Enzylite Acetylcholine Assay Kit by Assay Biotech Company USA. Assay involved acetylcholinesterase catalyzing the reaction of acetylcholine to choline. Acetyl cholinesterase activity in the brain homogenate was assayed as described by Ellman et al., [8].Determination of Brain SerotoninThe method of Ebuehi et al. [9] was used to determine brain serotonin concentration in the rat. The brain serotonin concentration was determined using high performance liquid chromatography (HPLC), Agilent 100 series with VWD detector degasser, Quat Pump, Col Com and a manual injector system. Tissue samples were prepared for injection by liquid phase extraction using chilled acetonitrile, to deproteinize the proteins. The mixture was centrifuged at 10,000g for 10min and the supernatant was collected and injected into the column. A gradient HPLC method was used to separate and quantify the brain serotonin, and L tryptophan. Chromatographic separations were carried out on a Hypersil ODS C18 reverse phase column (250 x4.0mm) packed with 5mm particles. Determination of Reduced Gluthatione (GSH)The determination of reduced glutathione is based on the formation of a yellow colour after reacting with 5,5'dithiobis- 2-nitrobenzoic acid (DTNB), which is then read at 412nm [10].Determination of Catalase Activity (CAT)The activity of the enzyme catalase was analysed according to Cohen et al, [11] which measures the initial rate of hydrogen peroxide (50mM) decomposition, where one unit is the amount of enzyme that hydrolyses 1 mol. of H2O2 per minute.Determination of Super Oxide Dismutase ActivityThe super oxide dismutase (SOD) activity was determined according to the modified method by Sun and Zigma[12]. The SOD enzyme assay is determined by difference between superoxide anion decomposition and production, that is, its ability to inhibit the autoxidation of epinephrine, which was determined by the increase in absorbance at 320nm [13].Determination of Lipid PeroxidationMalondialdehyde (MDA), formed from the breakdown of polyunsaturated fatty acids, serve as a convenient index for determining the extent of lipid peroxidation that reacts with thiobarbituric acid to give a red specie absorbing at 535nm [14].Histopathological ExaminationTissues were fixed in formaldehyde, haematoxylin and eosin–stained. 5-mm thick sections were used and then examined by light microscope [15]. Reports were obtained and Photomicrographs (magnification: x400) were then developed.
3. Results
The results show a significant loss in body weight (Fig 1) and a decline in the cognitive performance (presented as the time spent in the light compartment before entering the dark compartment) of the higher dosed rats which became prominent in all groups as feeding continues during the learning and memory tasks (Fig 2).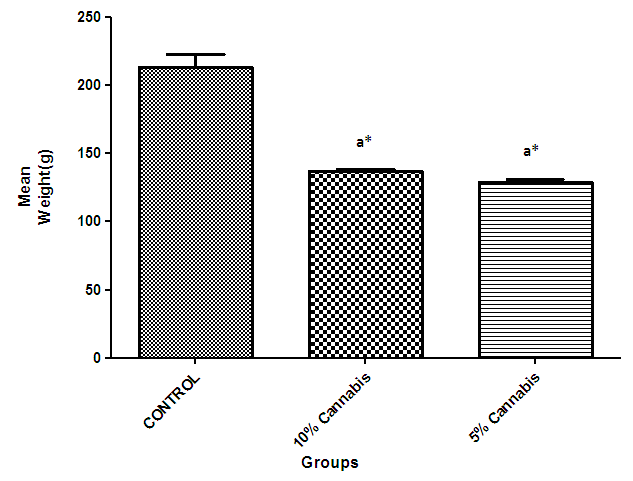 | Figure 1. The mean weight (grams) during the period of cannabis administration for 7weeks |
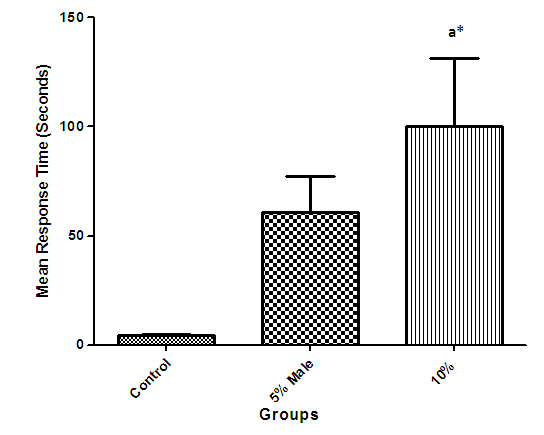 | Figure 2. The mean cognitive test response time of different groups (mean time required to move from light to dark compartment) |
The C.sativa fed group had reduced brain serotonin content (Fig 3) which is more chronic in the higher dosed group (10%). There was a significant (p<0.01) decrease in the brain acetylcholine levels and the activity of the acetylcholine esterase, presented in Fig 4 and 5 respectively. Fig 6 shows a significant decrease in the reduced Glutathione (GSH) level of the 10% percent test group which is insignificant in the 5% test group. Fig 7 sows that increase Catalase activity was not significant for the 5% test group, but rather significant decrease in 10% test group. A significant difference between the antioxidant capacity of 5% cannabis test group and the 10% test group was also observed. There was a no significant difference in the superoxide dismutase (SOD) concentration across the group (fig 8). The Malondialdehyde (MDA) in Fig 9 had a significant shoot up in the 10% test group only, as compared to the control group. 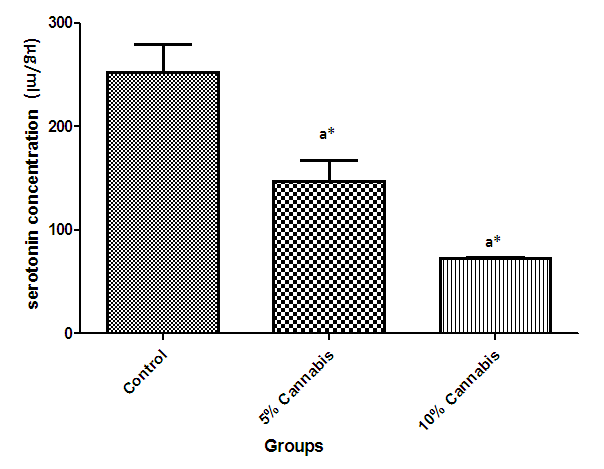 | Figure 3. The mean serotonin concentration (µg/ml) of brain of different exposed groups of rats |
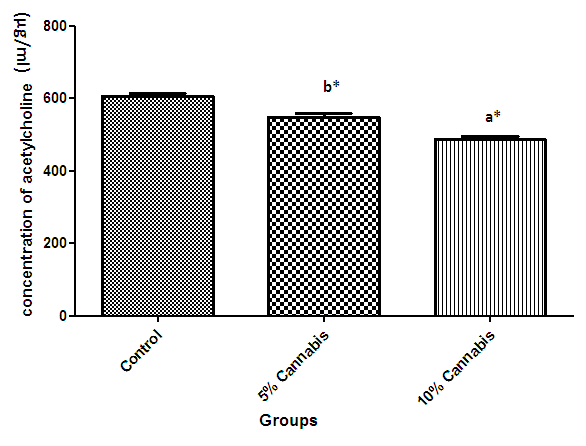 | Figure 4. The mean concentration of brain Acetylcholine of different exposed groups (µg/ml) of rats |
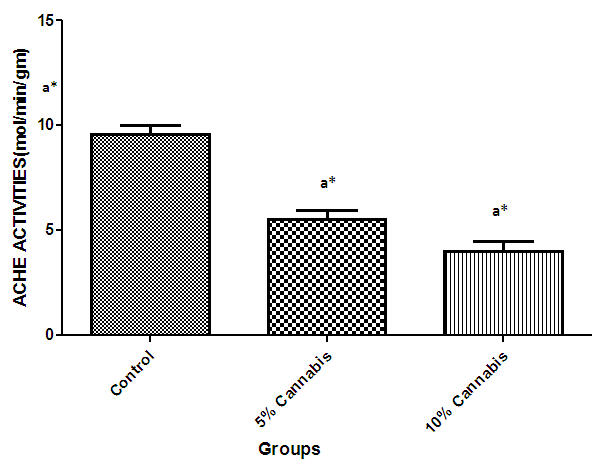 | Figure 5. The mean Acetylcholine Esterase (ACHE) activity (mol/min/gm) in the brain of different exposed groups of rats |
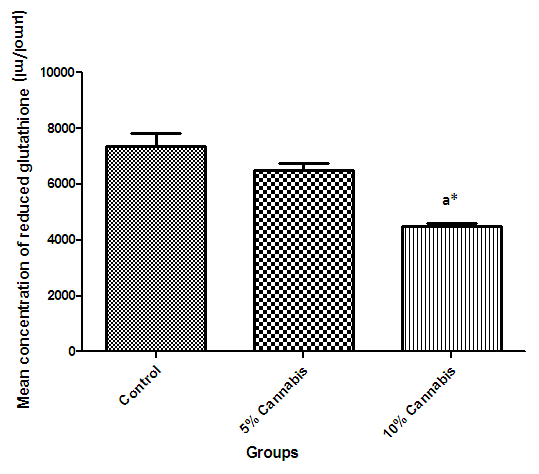 | Figure 6. The mean concentration (µmol/ml) of reduced Glutathione in brain of different exposed groups of rats |
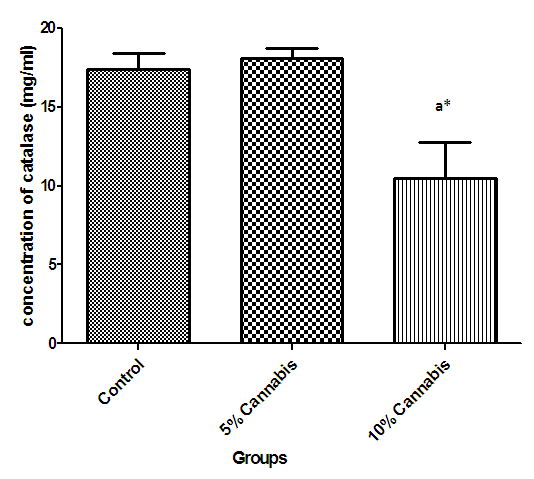 | Figure 7. The mean concentration (mg/ml) of Catalase in brain of different exposed groups of rats |
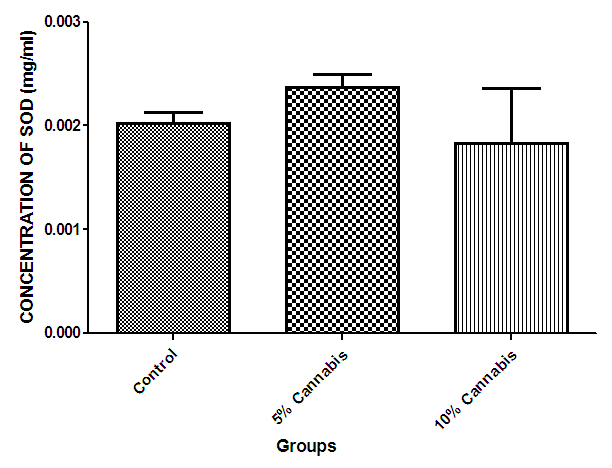 | Figure 8. The mean concentration (mg/ml) of Super Oxide Dismutase (SOD) in brain of different exposed groups of rats |
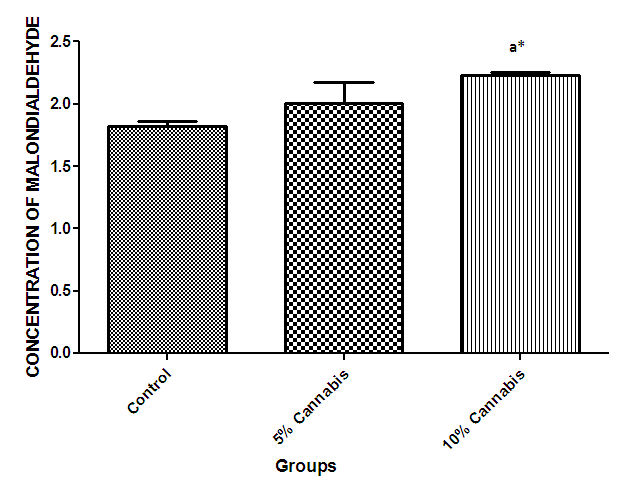 | Figure 9. The mean concentration (nmol/ml) of Malondialdehyde (MDA) in brain of different exposed groups of rats |
Statistical AnalysisData were analyzed for significance by Analysis of Variance (ANOVA), followed by Post HOC to compare significance between groups. Results were expressed as mean ± Standard error (SE) shown as error bar. Values were considered significant at P<0.05.a: significantly different from control groupb: significantly different from the 10% exposed groupAsterisks indicate the level of significance (*P<0.05; **P< 0.01) Table 1. Showing the histopathology report on the effect of different cannabis % administration on rat’s brain structure after seven (7) weeks 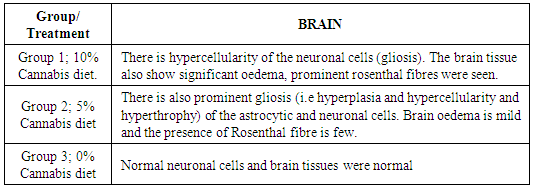 |
| |
|
4. Discussion
The duration of time spent in the light compartment of the shuttle box by the rats before entering the dark compartment was dose dependent on administration of C.sativa. There was significant (P<0.05) difference between the time spent in the light compartment by rats administered C.sativa and control. This confirms that there is a deficit in the learning ability and cognitive functions of the test animals. This can be linked to the action of Tetrahydrocannabinol on the Cannabinoid1 (CB1) receptors found in many areas of the brain involved in memory, as earlier reported by Agranoff, [16]. The Acetylcholinesterase (AchE) activity is reduced in both 5% and 10% test groups, in a dose dependent manner, supporting the fact that decrease in AchE activity is associated with loss of cognitive function [17]. Several studies have implicated satisfactory activity of Acetylcholine esterase in learning, memory and cognitive performance, this further explain the neurochemical mechanism with which Cannabis sativa causes decline in cognitive function. Eubank [18] study also revealed that certain components of Cannabis sativa is capable of inhibiting the enzyme AchE, this is therefore linked with the decrease in activity observed. The decreased level of brain acetylcholine concur with earlier report by Katona et al. [3] Certain cannabis components are known to cause a form of slowing down to the acetylcholine system [19], this could have led to the decrease in neuronal activity in that region that may result from damages in cells which produce and utilize acetylcholine such that there is a reduced amount available to carry messages required in long term potentiation (LTP) involved in learning and memory, the reduced level of acetylcholine slows down neuronal signalling leading to Attention Deficit (AD) as observed in the shuttle box training.The test group had a lowered level of brain serotonin concentration. The reduction in the brain level of serotonin could be linked to the ability of Tetrahydrocannabinol (THC) to cause degeneration in the serotonergic neurons, leading to weaker communications between specific brain regions (amygdala and frontal lobes) compared to that under normal serotonin level [20]. Low level of serotonin in the brain is implicated in increased susceptibility loss of cognitive function.Oxidative stress occurs due to the increase in generation of free radical or the decrease in capacity to scavenge free radicals. Pool of antioxidant enzyme decreased after a period of seven weeks in the 10% test group, whereas, the 5% test group had no statistically significant difference in the level of catalase and superoxide dismutase, after seven weeks of administration. The level of reduced glutathione (GSH) reduced drastically in the 10% test group but insignificant in the 5% group. The MDA undergo shoot up in 10% group, there was also no observable significant increase in MDA of the 5% test group. In all, there were significant difference between the 10% cannabis test group and the 5% cannabis test group. This shows that the antioxidant property of the brain was gradually outweighed by the free radical generating components of the consumed cannabis in a dose dependent manner. Although it has been reported that Cannabis has certain antioxidant capacity, confirmed in the 5% group at early stage of administration, the effect of its free radical generating components may have led to the decrease in the endogenous pool. This effect could be linked to increased metabolic activity caused by Cannabis sativa, increase in the electron leakage from the mitochondrial transport system causing an increase in oxidative stress and generation of the free radicals with increased vulnerability to cellular damage, as explained by Muller, [17]. The cellular damage by the oxidative stress has a resultant effect on loss of cognition and altered neurotransmitter system [21].The histopath report reveal altered structure of the brain neuronal and glial cells, as increased cellularity, hypertrophy of glial cells and hyperplasia in the brain following administration of Cannabis sativa compared to the control group. This altered architecture explains the lowered cognitive and learning activity exhibited by the experimental rats.
5. Conclusions
The study emphasizes that chronic Cannabis sativa users are at higher risk of cognitive deficit and memory impairment, the imbalance in the antioxidant, serotoninergic and cholinergic system might correlate to the cognitive deficit and structural alteration of the rat’s brain observed. Cannabis sativa is implicated in a dose-dependent manner in certain deleterious defect in cognitive function of the brain learning ability in the sprague-dawley rats with reference to altered functional level of the neurotransmitter system and redox status serving as the underlying neurochemical change that led to cognition deficit which can be extrapolated to humans, presenting an opportunity for pharmacological intervention. This experiment therefore represents a significant contribution to the knowledge on mechanism of action involved in cognitive deficit by Cannabis sativa. The result support therapeutic potential targeting serotonin pathway and cholinergic system to treat Cannabis sativa induced neurobehavioral and cognitive deficits which affects the long term quality of the individual’s life.
ACKNOWLEDGEMENTS
The authors are grateful to Mr Duncan Ota and Chizokie. O, of the Department of Physiology and Pharmacology respectively, College of medicine, University of Lagos, Lagos, for their technical assistance in the study.
References
[1] | SAMHSA 2011. Results from the 2010 National Survey on Drug Use and Health: Summary of National Findings. Rockville, MD. 1-877-SAMHSA-7 (1-877-726-4727). |
[2] | Guxens M, Nebot M, Ariza C, Ochoa D 2007. Factors associated with the onset of cannabis use: a systematic review of cohort studies. (3):252-60. |
[3] | Katona I, Rancz EA, ACSANDY L, et al Distribution of CBI cannabinoids receptors in amygdale and their role in the control of GABAergic transmission. J. Neurosci; 21: 9506-18, 2001. |
[4] | Steckler, T., Sahgal, A., 1995, Behav. Brain Res. 165-199. Sun M., Zigman S., 1978 An improved spectrophotometric assay for superoxide dismutase based on epinephrine autoxidation. Anal. Biochem,. 90: 81-89. |
[5] | Ameri A (1999) The effects of cannabinoids on the brain. Prog Neurobiol 58:315–348. |
[6] | Ebuehi O, Kannone C, Balogun A, Akiwande A, Famuyiwa O (2009)., effect of administration of sertraline, chlozapine, amitriptyline and imipramine on brain serotonin, Liver enzymes and blood chemistry of rabbits, in journal of biological chemical science, 3(1) 85-94 |
[7] | Ebuehi, O.A.T., Akinwande A.T., Famuyiwa O.O., Adebayo O.A., Uzo Dinma., E.O., Onwumere O.A., Masade O.N and Anesimojubi 2005. Effect of marijuana smoking on blood chemistry and serum biogenic concentration in Human Nig Journal of Health & biochemical science vol 4, No1 pg 20-24. |
[8] | Ellman G., Tissue sulfuhydryl groups. 1978. Arch Biochem. Biophys., 82(1):70-7. |
[9] | Ebuehi O.A.T and Adenrele, A.A 1999. Effect of Marijuana and caffeine consumption in brain acetylcholine esterase alkaline phosphatase in rats. West African J. Pharmacol & drug research, vol 15, No. (1&2) 136-43 |
[10] | Ellman C.L, Courtney, D, Andres V, and Featherstone R 1991. A new and rapid colorimetric determination of acetyl cholinesterase activity. Neuroscience 32: 601- 602. |
[11] | Cohen G., Kim M., and Ogwa V., 1996 A modified catalase assay suitable for a plate reader 56 |
[12] | Hammond AC 1994, Animal well being, in pharmacology and toxicology research. J. Anim sci; 72: 523-527. |
[13] | Moelker, Wendy 2008. "How does Marijuana Affect Your Body? What are the Marijuana Physical Effects review of cohort studies. Gac Saint 21: 252–260. |
[14] | Buege J and Aust S. 1978. Microsomal lipid peroxidation. In Fleisher S., Packer L, (Eds) Methods in enzymol.vol.52. Academic Press Inc., New York, pp 302-310. |
[15] | Shiojiri N. 1984 Analysis of differentiation of hepatocytes and bile duct cells in developing mouse liver by albumin immunofluorescence. Develop Growth Differ; 26: 555-561. |
[16] | Agranoff B. 2002. Learning and memory. Approaches to correlating behaviour. In Basic Neurochemistry (Albers RW. Siegel G. J (eds). Little Brown, Boston USA p 765-781. |
[17] | Muller, D.P., 1994 Vitamin E and Other Antioxidants in Neurological Function and Disease, ch. 19, p. 535-565. |
[18] | Eubanks, Lisa M, Rogers, Claude J, Beuscher, 4th: Koob, George F, Olson, Arthur J, Dickerson, Tobin J, Janda, Kim D, “A molecular link between the active component of marijuana and alzhemier’s Disease Pathology” Molecular pharmaceutics 3 (6): 773-7, 2006. |
[19] | Steckler, T., Sahgal, A., 1995, Behav. Brain Res. 165-199. |
[20] | Iversen L 2003. Cannabis and the brain. Brain 126: 1252–1270. |
[21] | Solowij N, Stephens R, Roffman RA, Babor T. 2002. "Does Marijuana Use Cause Long-term Cognitive Deficits?". JAMA 287 (20): 2653–4. |