Olufowora Olorunleke Yinka1, 2, Akinwande Sinmiloluwa O.2, 3
1Microbiology Department, Ekiti State University, Ado-Ekiti, Nigeria
2Environmental and Petroleum Technology Management Department, South America University, Delaware
3Microbiology Department, Obafemi Awolowo University, Ile-Ife Osun State, Nigeria
Correspondence to: Olufowora Olorunleke Yinka, Microbiology Department, Ekiti State University, Ado-Ekiti, Nigeria.
Email: |  |
Copyright © 2021 The Author(s). Published by Scientific & Academic Publishing.
This work is licensed under the Creative Commons Attribution International License (CC BY).
http://creativecommons.org/licenses/by/4.0/

Abstract
In many developing countries, the role of Microbiologically Influenced Corrosion (MIC) is still limited especially in transporting pipelines because an abiotic mechanism can be invoked to explain the observed corrosion. A research on the role of microorganisms in oil transporting pipelines in Escravos gas plant, Delta state, Nigeria was conducted. The microbial count was assayed using pour plate method after which the identification of sulfate-reducing bacteria (SRB), total heterotrophic bacterial, and fungal counts were also carried out. The corrosion rates were determined by the weight loss method. The sulfate-reducing bacteria, total heterotrophic bacterial and fungal counts of the biofilm samples ranged from 4.81 × 103 to 8.56 × 103 CFU/ml, 1.22 × 104 to 2.60 × 104 CFU/ml, and 4.14 × 103 to 8.52 × 103 CFU/ml respectively. The results revealed Desulfovibrio magneticus, Desulfobulbus propionicus, and Desulfuromonas acetoxidans as sulfate-reducing bacteria present. The bacteria species identified isolates include gram-positive Bacillus subtilis, Bacillus cerus, Pseudomonas aeruginosa, Staphylococcus aureus, Staphylococcus epidermidis, and Halomonas subglaciescola while the identified gram negative organisms include Klebsiella oxytoca and Seratia marcescen. The fungal isolates include members of the genus Microsporum, Saccharomyces, Aspergillus, Fusarium, and Verticillium. The weight-loss method was used to determine the rate of corrosion and the mean values of corrosion rates in each pipeline ST1, ST2, ST3, ST4, and ST5 had corrosion rates of 4.47, 3.28, 4.22, 2.56, and 3.19mpy respectively. These results will therefore alert oil companies, the government, and other agencies of the possible microbiologically influenced corroding effects on pipelines.
Keywords:
Sulphur reducing bacteria, Oil pipelines, Microbial Corrosion, Escravos Gas Plant
Cite this paper: Olufowora Olorunleke Yinka, Akinwande Sinmiloluwa O., Analyses of Microbial Effect Corroding Oil Pipelines in Escravos Gas Plant, Nigeria, Journal of Microbiology Research, Vol. 11 No. 1, 2021, pp. 1-7. doi: 10.5923/j.microbiology.20211101.01.
1. Introduction
Oil and gas pipelines play an essential role in delivering the energy resources required to power communities all over the world. However, pipeline networks are affected by diverse corrosion deterioration mechanisms, as well as Microbiologically Influenced Corrosion (MIC) derived from accelerated deterioration caused by various microbial activities found within the hydrocarbon systems (Muthukumar, 2014). When pipelines become exposed to water, corrosion happens immediately. Corrosion is an electrochemical process that undergoes two partial reactions, an anodic reaction that corrodes metals and a cathodic reaction that causes the reduction of some species. In some cases, corrosion reaction is affected by the presence of microbes by producing biofilms on the metal surface (Płaza and Achal, 2020). Microbiologically Influenced Corrosion (MIC) is capable of accelerating the mechanical failure of metals in various environments ranging from oil and water pipelines and machinery to biomedical devices. However, this mode of environmentally aided corrosion has no single definition. MIC includes corrosion in the presence of microorganism species as well as bacterium and the biofilms made by those bacteria (George et al., 2012).Microbial corrosion mostly leads to a type of corrosion that manifests pitting, crevice corrosion, under-deposit corrosion, cracking enhanced erosion-corrosion, and dealloying (Tingyue, 2011). Microbial activities have been reported to cause about 75% of the corrosion in oil wells and more than 50% of pipeline system failures (Kwan et al., 2013).Hydrocarbon acts as a major source of substrate for many microorganisms in the pipeline, which also contains sulphur, nitrogen, carbon, phosphorus and many other elements which may serve as important substrates for pipeline microbes. The main type of microorganisms associated with metals in pipeline systems are sulfate-reducing and Sulfate-oxidizing bacteria, iron and CO2 reducing bacteria, iron and manganese oxidizing bacteria, and acid-producing fungi (Manafi et al., 2013). Sulfate-reducing bacteria creates complications when metal structures and sulfate-containing water get exposed to each other. This exposure or interaction causes a layer of molecular hydrogen on the metal surface, which then leads to the oxidation of the hydrogen by sulfate-reducing bacteria thereby creating hydrogen sulfide, which contributes to corrosion (Biljana, 2012).The aim of this analysis work is thus targeted on the impact of microorganisms on oil transporting pipelines by analysing the biofilm samples from pipeline at Escravos dittany, Delta state, Nigeria.
2. Materials and Method
The biofilm samples were attained by placing ten mild steel coupons (surface area 4cm2 and density 7.57g/cm3) at the inner surface of the pipelines, through the access value at five different pipelines made up of the same materials and then exposed for 10 weeks (70 days) to the flow of petroleum. The involved pipelines were located at the Escravos gas plant, Warri, Delta State, Nigeria, where crude oil and gas are extracted. The coupons were detached from the inner region of oil pipelines and the biofilms formed on the surfaces of each coupon were removed with sterile razor blade and collected into sterile bottle with 20ml phosphate-buffered saline, pH7 (Sambroak and Rusell, 2001). The biofilm samples were named as A, B, C, D and E, corresponding to each oil pipeline. Corrosion Rate:After the removal of the biofilms, the coupons were washed in acetone, dried and the final weight taken using
0.0001 accuracy electric balance and the differences in weight (weight loss) used to determine corrosion rate using the formula.The weight loss of a corrosion coupon after exposure to a corrosive environment, expressed as mils (thousandths of an inch) per year penetration. Corrosion rate is calculated assuming uniform corrosion over the entire surface of the coupon. (ASTM, 2012)mpy = (weight loss in grams) X (22,300)/(Adt)mpy = corrosion rate (mils per year penetration)A = area of coupon (sq. in.)d = metal density of coupon (g/cm3)t = time of exposure in corrosive environment (days).Preparation of potato dextrose agar (PDA) and nutrient agar (NA)About 39 g of potato dextrose agar (PDA) was accurately weighed and dissolved in 1000 mL of deionized water. About 28 g of nutrient agar was accurately weighed and dissolved in 1000 mL of distilled water. To prevent bacterial and fungal contaminations, 2 mL of tetracycline and nystatin was added. The media were prepared, mixed thoroughly and sterilized by autoclaving at 121°C for 15 min, as specified by Barnet and Hunter, 1987.Sulfate-reducing Bacteria Medium.The Postgate standard medium was used for the growth of Sulfate-reducing bacteria (SRB). The composition of the Postgate B medium are KH2PO4 (0.5g/ml), NH4Cl (1.0g), Na2SO4 (1.0g), CaCl2 .6H2O (0.1g), MgSO4 7H2O (2.0g), Sodium Lactate (60-70%) 5ml, Yeast extract (1.0g), Ascorbic acid (0.1g), thioglycelic acid (0.1g), FeSO4 7H2O (0.5g), NaCl (26.0g); (Postgate 1984). All the components were dissolved in 1 litre of distilled water and the pH was adjusted to 7.5–8.0 using 5M Hcl. After this, the solution was homogenized by agitation and later sterilized at 121°C for 30 min.Isolation of fungi and bacteria from the collected samples.The microorganisms, fungi and bacteria were isolated by serial dilution plate method. About 1 g of the sample was accurately weighed and suspended in 9 mL of double distilled water to make microbial suspensions (10-¹ to 10-³). Dilutions of 10-² and 10-³ were used to isolate both fungi and bacteria. 1 mL of microbial suspension of each concentration were added to sterile Petri dishes (triplicate of each dilution) containing 15 mL of sterile PDA and NA for bacteria and fungi isolation and incubated for four days at 4°C (bacterial) and 37°C for 24 h (fungal). The growth of the organisms were counted using colony counter and the microbial loads were calculated in CFU/mL although no growth was observed on fungal plates after 4 days of incubation. Therefore, only bacterial colonies were sub-cultured by streaking on new solidified nutrient agar plates to obtain pure cultures. After pure isolates have been obtained, they were stored in slant bottles for characterizations as specified by Barnet and Hunter, (1987).Characterization of the bacterial isolatesColonial characteristics of the bacterial isolates were determined using parameters such as size, elevation, pigment, surface, opacity, edge and shape (Holt et al., 1994). Cellular characteristics of the isolates were determined by gram staining, spore staining, motility test, oxidase test, catalase test, methyl red test and indole test.
3. Results
Five biofilm samples obtained from five different pipeline locations at the Escravos gas plant, Warri, Delta State, Nigeria, were microbiologically analysed. The pipeline stations were labelled Station 1 (ST1), Station 2 (ST2), Station 3 (ST3), Station 4 (ST4) and Station 5 (ST5). The microbial load of fifteen samples collected from the pipelines are shown in (Fig 1, supplementary table 1). The average sulfate-reducing bacteria count (SRB) of biofilm samples on ST1, ST2, ST3, ST4 and ST5 estimated ranged from 8.01 × 103 CFU/ml, 4.81× 103 CFU/ml, 8.56 × 103 CFU/ml, 5.91× 103 CFU/ml and 4.81 × 103 CFU/ml, respectively; The range of estimated average total heterotrophic bacteria (THBC) of biofilm samples on the pipelines were between 2.60 × 104 CFU/ml, 1.40 × 104 CFU/ml, 2.15 × 104 CFU/ml, 2.55 × 104 CFU/ml and 1.22 × 104 CFU/ml, respectively; while Total fungal counts (TFC) estimate ranged from 8.52 × 103 CFU/ml, 7.63 × 103 CFU/ml, 4.55 × 103 CFU/ml, 4.14 × 103 CFU/ml and 7.46 × 103 CFU/ml respectively (Fig 1, supplementary table 1).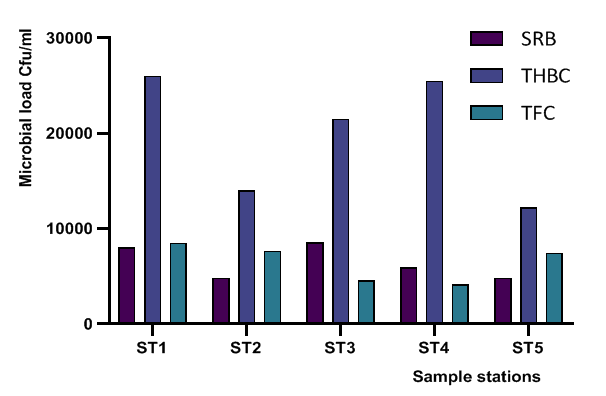 | Figure 1. Microbial Load of Samples Isolated from station one (ST1) to station five (ST5) in the oil pipelines (CFU/ml) |
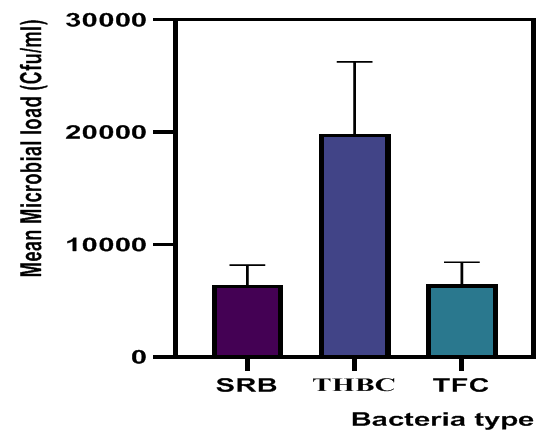 | Figure 2. Average Microbial Load of Sulfate-reducing bacteria (SRB), Heterotrophic bacteria (THBC) and Fungal counts (TFC) (CFU/ml) |
Figure 3 shows the identified sulfate-reducing bacteria isolated from the oil pipelines. The isolates includes Desulfovibrio magneticus, Desulfobulbus propionicus, and Desulfuromonas acetoxidans. The identified heterotrophic bacteria isolated from the oil pipelines are shown on (Fig 4, supplementary table 3). The isolates includes gram positive Bacillus subtilis, Bacillus cerus, Pseudomonas aeruginosa, Staphylococcus aureus, Staphylococcus epidermidis and Halomonas subglaciescola while the identified gram negative organisms includes Klebsiella oxytoca and Seratia marcescen. 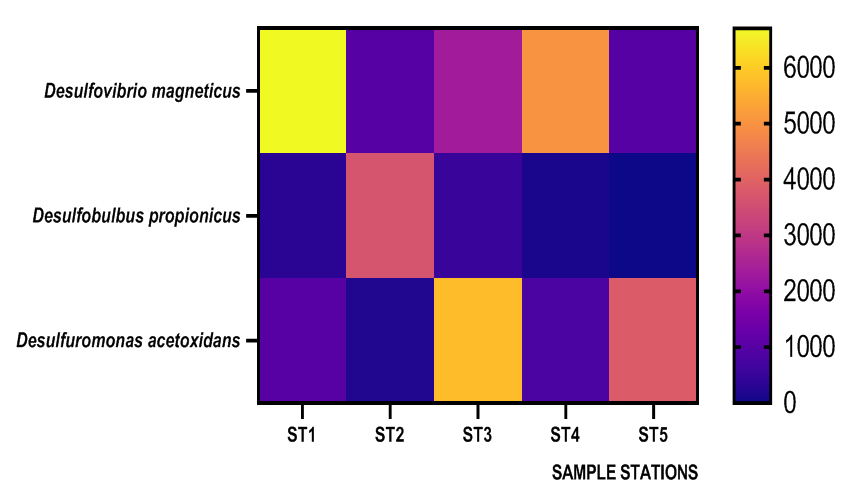 | Figure 3. Heat Map representation of sulfate-reducing bacteria (SRB) Isolated from the five stations |
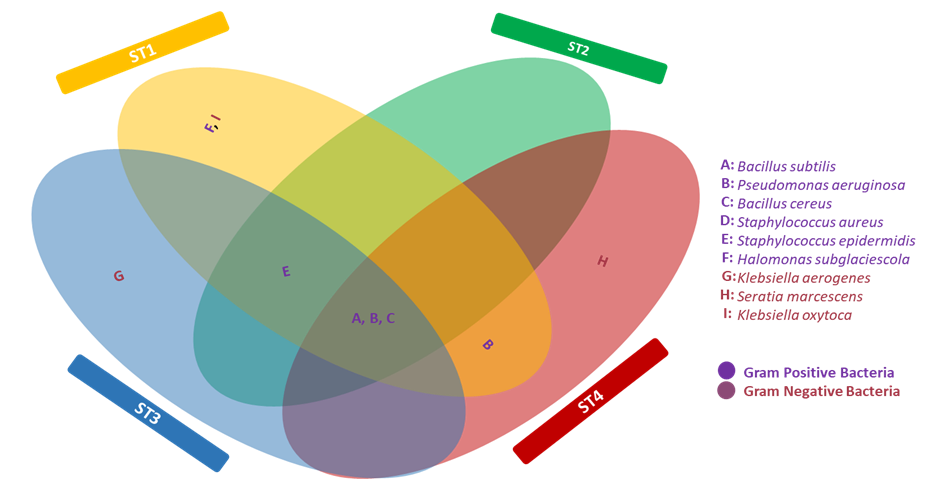 | Figure 4. Distribution of Heterotrophic Gram positive and Gram Negative Bacteria Count Isolated from Oil Pipelines |
The fungal isolates were also identified and most of them belongs to the genera Microsporum, Saccharomyces, Aspergillus, Fusarium and Verticillium. These organisms are shown on Table 1. The rates of corrosion for the pipelines were also carried out with ST1, ST2, ST3, ST4 and ST5 had corrosion rates of 4.47, 3.28, 4.22, 2.56 and 3.19 mpy respectively (Fig 5, supplementary table 4).Table 1. Total Fungal Count Isolated from Oil Pipelines 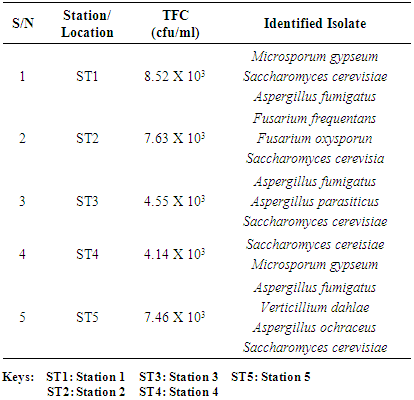 |
| |
|
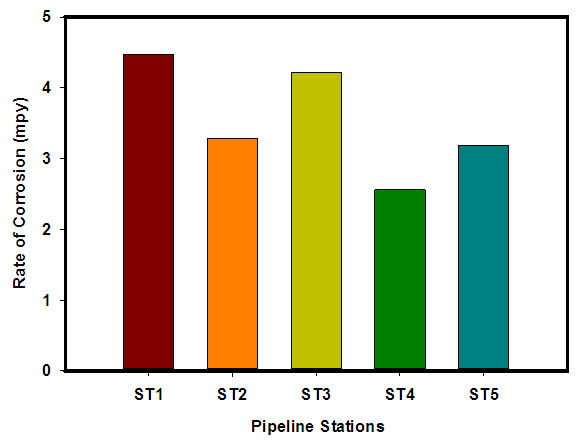 | Figure 5. The rate of Corrosion for the five stations in the oil pipeline |
4. Discussion
Researches on the role of MIC especially in transporting pipelines are rarely reported and often ignored if an abiotic mechanism can be invoked to explain the observed corrosion. MIC includes corrosion in the presence of microorganism species as well as bacterium and the biofilms made by those bacteria (Płaza and Achal, 2020). The microbiological assessment of five biofilm samples obtained from five different pipeline stations at the Escravos gas plant, Warri, Delta State, Nigeria, were carried out. The total microbial load of all stations analysed revealed the presence of sulphur reducing bacteria, heterotrophic bacteria and fungi (Fig 1, supplementary table 1); Similar result have previously been reported (Queiroz et al., 2018; Akpan and Edet, 2016). In our study, the total heterotrophic bacteria count (THBC) had the highest population in all stations tested especially in stations 1, 3 and 4; while sulphur reducing bacteria (SRB) and total fungal count (TFC) had similar average colony forming units across all stations. Stations 1 and 3 recorded the highest domination of SRB (Fig 1, Fig 2, supplementary table 1). The SRB community in this study includes Desulfovibrio magneticus, Desulfobulbus propionicus, Desulfuromonas acetoxidans (Fig 3, supplementary table 2). The presence of sulfate-reducing bacteria D. propionicus and D. acetoxidans is in conformation with the report of Akpan and Effiong (2013), which stated the presence of Desulfuromonaes acetoxidans, Desulfobulbus propionicus and Desulfosarcina variabilis as sulfate-reducing bacteria isolated in the study. Desulfosarcina variabilis was not noticed in this study but Desulfovibrio magneticus (which in fact had the highest presence amongst all SRBs in this study) identified which is in agreement with a report by Kiani et al., (2019). In his study, the presence of SRB particularly Desulfovibrio sp. was the major cause of corrosion on oil pipeline. In this study, the identification of sulfate-reducing bacteria (SRB) indicates the role of particular bacteria in the process of corrosion in pipelines and is chiefly due to the presence of products which degrades hydrocarbon and provides the sulfate-reducing bacteria a good source of energy for their physiological activities.Heterotrophic isolates in this study includes gram positive Bacillus subtilis, Bacillus cerus, Pseudomonas aeruginosa, Staphylococcus aureus, Staphylococcus epidermidis and Halomonas subglaciescola while the identified gram negative organisms includes Klebsiella oxytoca, Klebsiella aerogenes and Seratia marcescen. All heterotrophic bacteria isolated during this investigation have also been reported in a similar study by Maruthamuthu et al., (2016) which were all present in the corrosion products collected in their study. Higher ratio of the heterotrophic organisms analysed where gram positive while a lesser ratio where found to be gram negative. This was also observed previously by Roblero et al., (2004).In this analysis of biofilms in pipelines, the presence of Klebsiella oxytoca and Klebsiella aerogenes alludes to the fact that bacteria that produce acid may have a tangible contribution to corrosion. This is also revealed with high corrosion rate on ST1 and ST3 having the presence of either of the gram negative Klebsiella sp. (Fig. 4). Klebsiella oxytoca which was present in the pipeline microbial analysis is usually present in soil and water and responsible for nitrogen fixation under anaerobic or microaerobic condition, produces nitrates and/or nitric acid that may contribute to metal corrosion (Temme et al., 2012; Akpan and Edet, 2016). Psesudomonas aeruginosa and Klebsiella oxytoca isolated have minimal nutritional requirement and often present in aquatic environments that are rich in organic pollutants such as gasoline and solvents (Odeyemi et al., 2016). In addition, Pseudomonas aeruginosa contributes to biofilm formation by producing exopolysaccharides and facilitating the attachment of other microorganisms (Akpan et al., 2013, Odeyemi et al., 2016) and hence accelerates the corrosion processes (Batista et al., 2000). The strains such as Pseudomonas aeruginosa, Bacillus cereus and Bacillus subtilis gain energy from the oxidation of Fe2+ to Fe3+ and use ferrous ions as electron donors (Akpan et al., 2013b). The oxidation of ferrous to ferric ion by Klebsiella oxytoca, Pseudomonas aeruginosa, Bacillus cereus and Bacillus subtilis in this study indicates that the bacteria helps in the formation of ferric at low pH. Similar report have been made by (Kiani et al., 2019). The presence of ferric oxide indicates the role of iron oxidizing bacteria in the formation of corrosion products/sludge in oil pipelines revealed the presence of ferrous and ferric sulfate in the biofilm, which indicated the role of iron and manganese bacteria, while acid producers caused the formation of sulfate in water and biofilm (Queiroz et al., 2018). On the inner wall of the pipelines severe corrosion was evident on the pits on the coupons used in this research in addition, Halomonas subglaciescola was detected. This chemoorganotrophic bacterium, frequently isolated from marine and saline environments, produce organic acids from sugars (Andrea et al., 2001, Mata et al., 2002) and have been associated with biofilms or used as models in adhesion studies to solid substrates (Miguel et al., 2006).In this report, fungal isolates which are known for their ability to dwell in oil were found in the biofilm samples collected from the oil pipelines. Some of these organisms include Fusarium oxysporum, Microsporum gypseum and Aspergillus fumigates. They can grow successfully on oil component and are good producers of carboxylic acids which cause corrosion on iron. Acids like formic, citric and acetic acids are frequently produced by Fungi and these acids cause damage to metals including pipelines (Płaza and Achal, 2020).The weight loss method was used in determining the rate of corrosion in this investigation for each pipeline. The result for the rate of corrosion in this study correlates with the amount of sulphur reducing bacteria which had the highest in ST1 and ST3 for each analyses (Fig 1, Fig 5). We therefore suggest that the prevalence of corrosion in the pipelines may be due to the presence of sulphur reducing bacteria, acid producing bacteria, fungi and spore forming bacteria. Also, the rate of corrosion may probably be traced to the fact that resistance against the biocides and inhibitors used by the oil company might have been developed by the microbes in the pipelines and instead of inhibiting them, they are converted and used as substrate for their exponential growth (Akpan and Edet, 2016).
5. Conclusions
This study revealed that microorganisms are invariably present in oil pipelines and contribute immensely to the rate of corrosion in oil pipelines. From the research work, it is evident that the microbial load of the biofilm detected in the pipeline is high and mainly comprised of Sulphur-reducing bacteria, heterotrophic bacteria and fungi which has a great effects on the rate of corrosion of the pipeline. There is therefore an urgent need for awareness to be created about the present situation of microbiologically influenced corrosion to alert oil companies, the government, and other agencies; of the possible effects on corroding pipelines especially in developing countries. Further research should also be carried out to investigate the main reason and major source of resistance to biocides and corrosion inhibitors by these microorganisms. Meanwhile, a need for the development of new biocides and corrosion inhibitors to combat the corrosion effect caused by existing microbes should be considered.
Supplementary
Supplementary Table 1. Microbial Load of Samples Isolated from the Pipelines (CFU/ml) 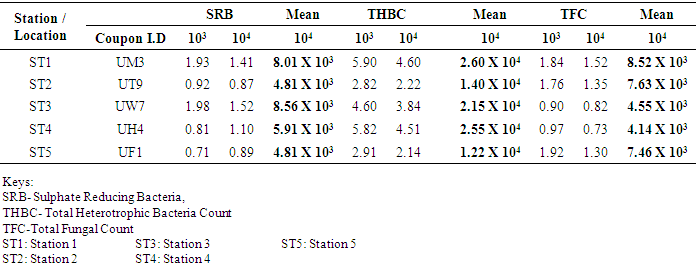 |
| |
|
Supplementary Table 2. Sulphate Reducing Bacteria Isolated from Oil Pipelines 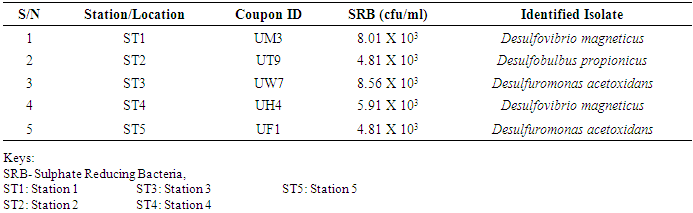 |
| |
|
Supplementary Table 3. Total Heterotrophic Bacteria Count Isolated from Oil Pipelines 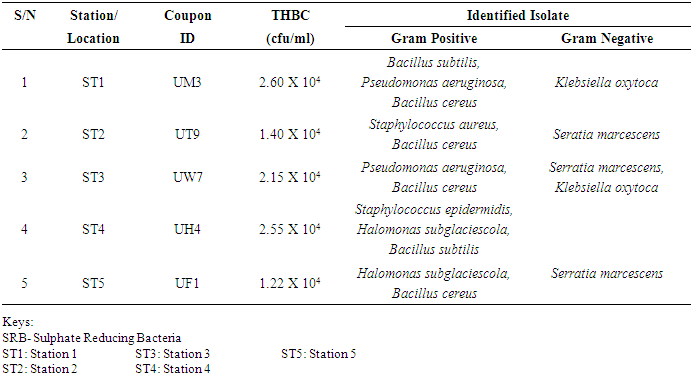 |
| |
|
Supplementary Table 4. Rate of Corrosion for the Oil Pipelines 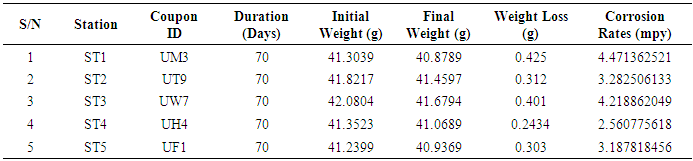 |
| |
|
References
[1] | Akpan, G. U. and Edet, I. A study on the impact of microbes on oil transporting pipelines in obiafun/obrikom, rivers state, Niger delta region, Nigeria. International Journal of Environment and Pollution Research. Vol.4, No.3, pp.62-72, July 2016. |
[2] | Akpan, G. U. and Effiong, G. S. (2013a). Evaluation of Chemical Properties of Produced water and its Potential Effects on the Rivers and Delta State Environment, Niger Delta. Research Journal of Science and IT Management. Vol. 2 (10) 16 – 26. |
[3] | ASTM (2012) Standard Guide for Determining Corrosivity of Crude Oils. West Conshohocken, PA: ASTM International. |
[4] | Barnet H.L. and B.B. Hunters, Illustrated Genera of imperfect Fungi. 4th Edition. U.S.A: Macmillan Publishing Company. 1987. |
[5] | Biljana S. Maluckov. (2012). Corrosion of steels induced by Microorganisms. Metall. Mater. Eng. 18 (3): 223-231. |
[6] | George, R. (2012). Current understanding and future approaches for controlling microbially influenced concrete corrosion: A review. Concr. Res. Lett., 3(3): 23-39. |
[7] | Gu, Tingyue. (2011). Understanding Microbiologically Influenced Corrosion (MIC) Mechanisms. The University of Akron, Akron. |
[8] | Holt, J.G. Kieg, N.R. Sneath, P.H.A. Staley J.T. and Williams. S.T. Bergey’s Manual of Determinative Bacteriology. 9th Edition. Baltimore, U.S.A: Williams and Wilkins Publishers, 1994. |
[9] | Kiani Khouzani, M.; Bahrami, A.; Hosseini-Abari, A.; Khandouzi, M.; Taheri, P. Microbiologically Influenced Corrosion of a Pipeline in a Petrochemical Plant. Metals 2019, 9, 459. |
[10] | Kwan, L., Matthew, W. and Krystyn, J. V. (2013). Beating the bugs: Roles of microbial biofilms in corrosion. Corrosion. 8: 38–45. |
[11] | Madigan, M. (2009). Brock Biology of Microorganisms, 12th Edn. Pearson Benjamin Cummings, San Francisco. U.S.A. |
[12] | Manafi, Z.; M. Hashemi, M. Abdollahi and G. Olson. (2013). Bio-corrosion of Water Pipeline by Sulfate-Reducing Bacteria in a Mining Environment. African Journal of Biotechnology, 12(46): 6504 – 6516. |
[13] | Maruthamuthu, S., Mohanan, S., Rajasekar, A., Muthukumar, N., Ponmarippan, S., Subramanian, P., Palaniswamy, N. (2016). Role of corrosion inhibitor on bacterial corrosion in petroleum product pipeline. Indian J. Chem. Technol. 12: 567–575. |
[14] | Miguel A. López, F. Javier Zavala Díaz de la Serna, Janet Jan-Roblero, Juan M. Romero, César Hernández-Rodríguez, Phylogenetic analysis of a biofilm bacterial population in a water pipeline in the Gulf of Mexico, FEMS Microbiology Ecology, Volume 58, Issue 1, October 2006, Pages 145–154. |
[15] | Muthukumar N. (2014). Petroleum products transporting pipeline corrosion - A Review. Amsterdam: Netherlands, Elsevier B.V. 2014; 528-567. |
[16] | Odeyemi, A. T., Olufowora, O. Y. and Fasuan, O. S. (2016). Plasmid Profile of Multiple Antibiotics Resistant (MAR) Bacteria Isolated from Leachate Samples in Ebira Communities of Ekiti Central and Ekiti South, Ekiti State, Nigeria. Int.J. Curr. Microbiol. App. Sci. 5(10): 478-493. |
[17] | Płaza, G. and Achal, (2020). V. Biosurfactants: Eco-Friendly and Innovative Biocides against Biocorrosion. Int. J. Mol. Sci. 21, 2152. |
[18] | Postgate J. R. (1984). The Sulfate – New York: Cambridge University Press. |
[19] | Queiroz, G.A.D.; Andrade, H.S.; Malta, T.B.S.; Vinhas, G.; Lima, M.A.G.D.A. Bioflm Formation and Corrosion on Carbon Steel API 5LX60 in Clayey Soil. Mater. Res. 2018, 21, e20170338. |
[20] | Sambroak J.F. and Russell, D.W. ed., Cold Spring Harbor Laboratory Press, 2001, 2100 pp., soft cover. |
[21] | Temme, K.; Zhao, D.; Voigt, C.A. Refactoring the nitrogen fixation gene cluster from Klebsiella oxytoca. Proc. Natl Acad. Sci. USA 2012, 109, 7085–7090. |