B. M. Olowe1, J. O. Oluyege1, O. Famurewa2, A. O. Ogunniran3, O. Adelegan4
1Department of Microbiology, Ekiti State University, Ado-Ekiti, Nigeria
2Department of Biological Sciences, Kings University, Odeomu, Nigeria
3Department of Science Laboratory Technology, Ekiti State University, Ado-Ekiti, Nigeria
4Department of Medical Microbiology and Parasitology, Afe Babalola University, Ado-Ekiti, Nigeria
Correspondence to: B. M. Olowe, Department of Microbiology, Ekiti State University, Ado-Ekiti, Nigeria.
Email: |  |
Copyright © 2017 Scientific & Academic Publishing. All Rights Reserved.
This work is licensed under the Creative Commons Attribution International License (CC BY).
http://creativecommons.org/licenses/by/4.0/

Abstract
Molecular identification of environmental isolates using 16S rRNA gene is very important when accurate and decisive bacterial identification is required. This study identified bacterial isolates (n = 9) from samples of different drinking water sources using 16S rRNA gene sequence. Bacterial 16S rRNA gene was amplified using suitable primers. The amplified 16S rRNA gene sequence was compared with the sequence in NCBI sequence database using Basic Local Alignment Search Tool (BLAST) search program. The BLAST results showed that isolates S71 was 91% similar to both E. coli strain S51 with accession number CP015995.1 and E. coli strain 268-78-1 with accession number CP014092.1, isolate S177 was 91% similar to both E. coli strain BACO64 with accession number KU161315.1 and E. coli strain BAC031 with accession number KU161312.1, S31 was 91% similar to E. coli strain 268-78-1 with accession number CP014092.1, isolates S89 and S34 were both 91% similar to E. coli strain OZK2 with accession number KT440880.1, isolate S152 was 89% similar to E. coli strain C-XIB with accession number KJ806504.1, isolates S33 and S35 were 91% similar to E. fergusonii strain CGS24 with accession number KX034239.1 while S30 was 91% similar to E. coli strain S51 with accession number CP015995.1. The phylogenetic tree showed two different clusters. The first cluster comprises S152, S177, S89, S71 which were identified as E. coli strain C-XIB, BAC064, OZK2, S51 respectively while the second cluster comprises S33, S31, S30, S34, S35 which were identified as E. fergusonii strain CGS24, E. coli strain 268-78-1, S51, OZK2, E. fergusonii strain CGS24 respectively. This study is believed to be the first study to provide a report which differentiates E. fergusonii from E. coli and on the incidence of E. fergusonii in drinking water sources in Nigeria.
Keywords:
Water sources, Escherichia coli, Escherichia fergusonii, 16S rRNA gene, BLAST, Phylogenetic tree
Cite this paper: B. M. Olowe, J. O. Oluyege, O. Famurewa, A. O. Ogunniran, O. Adelegan, Molecular Identification of Escherichia coli and New Emerging Enteropathogen, Escherichia fergusonii, From Drinking Water Sources in Ado-Ekiti, Ekiti State, Nigeria, Journal of Microbiology Research, Vol. 7 No. 3, 2017, pp. 45-54. doi: 10.5923/j.microbiology.20170703.01.
1. Introduction
Availability of safe drinking water is a key factor underpinning public health and development of any nation. Water sources available in Nigeria include: surface water such as lakes, streams, rivers, ponds and underground water such as springs, wells, borehole (Oluyege et al., 2009). There is always a link between environmental sanitation or sanitary practices of any community and the quality of water sources in that community. In Nigeria, overpopulation, increased urbanization, agricultural practices, industrialization, unhygienic practices among others has affected the quality of water sources (Olowe et al., 2016). Studies have revealed non-conformity of many water sources in Nigeria to World Health Organization (WHO) standard (Nwidu et al., 2008; Oyedeji et al., 2010; Kalpana et al., 2011; Ohanu et al., 2012; Gideon et al., 2013; Sunday and Innocent, 2014; Olowe et al., 2016). Some microorganisms of faecal origin have been implicitly implicated in contamination of water sources among which include the Escherichia species (Abdul, 2010).Escherichia species belong to the family Enterobacteriaceae and consists of six species which include: Escherichia coli, Escherichia fergusonii, Escherichia hermannii, Escherichia vulneris, Escherichia blattae and Escherichia albertii (Walk et al., 2009). Among these species, E. coli has been mostly studied and isolated from faecally contaminated water. Hence, it is being used as faecal indicator of choice in W.H.O. guidelines for drinking water quality (Rompre et al., 2002; WHO, 2006). In addition, several countries such as Europe and U.S.A. include this organism in their regulations as the primary indicator of faecal pollution (Odonkor and Ampofo, 2013).On the other hand, Escherichia fergusonii was proposed as a new emerging species within the genus Escherichia by Farmer et al. (1985). There are certain phenotypic differences which enabled it to be differentiated from other species within genus Escherichia, in particular, E. coli which was shown by DNA hybridization to be its closest relative (64% similarity) (Wragg et al., 2009). It can be differentiated from E. coli by being sorbitol and lactose negative but adonitol, amygdalin and cellobiose fermentation positive (Foster et al., 2010; Simmon et al., 2014). E. fergusonii has been isolated from a wide range of human clinical specimens, including blood, urine and faeces (Gaafar et al., 2015) at which time, the clinical significance of the species was not known. Occasional isolations of E. fergusonii from clinical samples have been reported in some countries like UK, France (Freney et al., 1987). In addition, it has been isolated from animals such as pigs, sheep, cattle, goats, horses, reindeer, ostriches, turkeys chickens (Oh et al., 2012; Gaafar et al., 2015) and water sources (Maheux et al., 2014) in some countries but to the best of our knowledge, its occurrence has not been reported in Nigeria. Furthermore, Chaudhury et al. (1999) has demonstrated the potential for E. fergusonii to cause enteric disease.Due to the close similarity between E. coli and E. fergusonii, molecular identification using 16S rRNA is very paramount. This is because the use of 16S rRNA gene sequences to study bacterial phylogeny and taxonomy has been by far the most common housekeeping genetic marker used for a number of reasons. These reasons include: its presence in almost all bacteria; often existing as a multigene family or operons; the function of the 16S rRNA gene over time has not changed, suggesting that random sequence changes are a more accurate measure of time (evolution) and the 16S rRNA gene (1,500 bp) is large enough for informatics purposes (Patel, 2001). Therefore, this study aimed to identify E. coli and E. fergusonii using 16S rRNA from water sources in Ado-Ekiti, Ekiti State, Nigeria.
2. Materials and Methods
2.1. Bacterial Culture
Nine bacterial isolates from different water sources: S30, S35, S71, S89 and S152, from well samples, S177 from stream sample, S31 and S33 from borehole samples and S34 from pipe-borne sample, which were identified culturally and biochemically as E. coli in a previous study (Olowe et al., 2015) were used for this study.
2.2. Extraction of DNA using CTAB Method
The bacterial isolates from different water sources were grown overnight in a liquid Nutrient Broth at 37°C. isolation of genomic DNA was carried out using CTAB method as described by Akinyemi and Oyelakin (2014). DNA concentration of each of the samples was measured on spectrophotometer at 260 nm and 280 nm and their genomic purity were determined.
2.3. DNA Electrophoresis
Agarose gel electrophoresis was used to determine the quality and integrity of the DNA by size fractionation on 1.0% agarose gels. Agarose gels were prepared by dissolving and boiling 1.0 g agarose in 100 mL 0.5X TBE buffer solution. The gels were allowed to cool down to about 45°C and 10 µL of 5 mg/mL ethidium bromide was added, mixed together before pouring it into an electrophoresis chamber set with the combs inserted. After the gel has solidified, 3 µL of the DNA with 5 µL sterile distilled water and 2 µL of 6X loading dye was mixed together and loaded in the well created. Electrophoresis was done at 80 V for 2 hours. The integrity of the DNA was visualized and photographed on UV light source. The extracted genomic DNA was used as template DNA for amplification of the 16S rRNA gene.
2.4. PCR Analysis using 16S Primer
PCR analysis was run with the universal primer for bacteria. The PCR mix comprised of 1 µL of 10 X buffer, 0.4 µL of 50 mM MgCl2, 0.5 µL of 2.5 mMdNTPs, 0.5 µL 5 mM forward and reverse primers, 0.05 µL of 5 units/µlTaq with 2 µL of template DNA and 5.05 µL of distilled water to make-up 10 µL reaction mix. The PCR profile used has an initial denaturation temperature of 94°C for 3 minutes, followed by 35 cycles of 94°C for 30 sec, 60°C for 40 sec, 72°C for 60 sec and the final extension temperature of 72°C for 5 minutes and the 10°C hold forever.
2.5. Purification of PCR Products
The amplicon was further purified before the sequencing, using 2 M sodium acetate wash techniques. To about 10 µL of the PCR product, 1 µL 2 M NaAct pH 5.2 was added followed by 20 µL absolute ethanol and kept at -20°C for 1 hour. It was then spun at 10,000 rpm for 10 minutes, washed with 70% ethanol and air dried. After which, was resuspended in 5 µL sterile distilled water and kept at 4°C for sequencing.
2.6. PCR for Sequencing
The primer used for the reaction was 16S. The PCR mix used included 0.5 µL of BigDye Terminator Mix, 1 µL of 5X sequencing buffer, 1 µL of M13 forward primer with 6.5 µL distilled water and 1 µL of the PCR product making a total of 10 µL. The PCR profile for Sequencing is a rapid profile, the initial Rapid thermal ramp to 96°C for 1 minute followed by 25 cycles of rapid thermal ramp to 96°C for 10 seconds, rapid thermal ramp to 50°C for 5 seconds and rapid thermal ramp to 60°C for 4 minutes, then followed by rapid thermal ramp to 4°C and hold forever.
2.7. Purification of PCR Sequencing Products
The PCR sequence product was also purified before sequencing running using 2M Sodium Acetate wash techniques. To 10 µL of the PCR product, 1 µL 2M NaAct pH 5.2 was added, then 20 µL absolute ethanol was added and kept at -20°C for 1hour. It was then spun at 10,000 rpm for 10 minutes, washed with 70% ethanol and air-dried. After which was re-suspend in 5 µL sterile distilled water and kept at 4°C for sequencing running.
2.8. Preparation of Sample for Gene Sequencer (ABI 3130xl Machine)
The Cocktail mix is a combination of 9 µL of Hi di formamide with 1 µL of purified sequence making a total of 10 µL. The samples were loaded on the machine and data in form Adenine, Cytosine, Thyamine, and Guanine were released.
3. Results
The genomic purity for all the DNA samples was between 1.8 –2.0. An absorbance quotient value of between 1.8 and 2.0 is considered to be good and purified DNA. A ratio of less than 1.8 is indicative of protein contamination, where as a ratio of greater than 2.0 is indicative of RNA contamination. The integrity of genomic DNA samples were confirmed on 1.0% agarose gel by electrophoresis (Figure 1).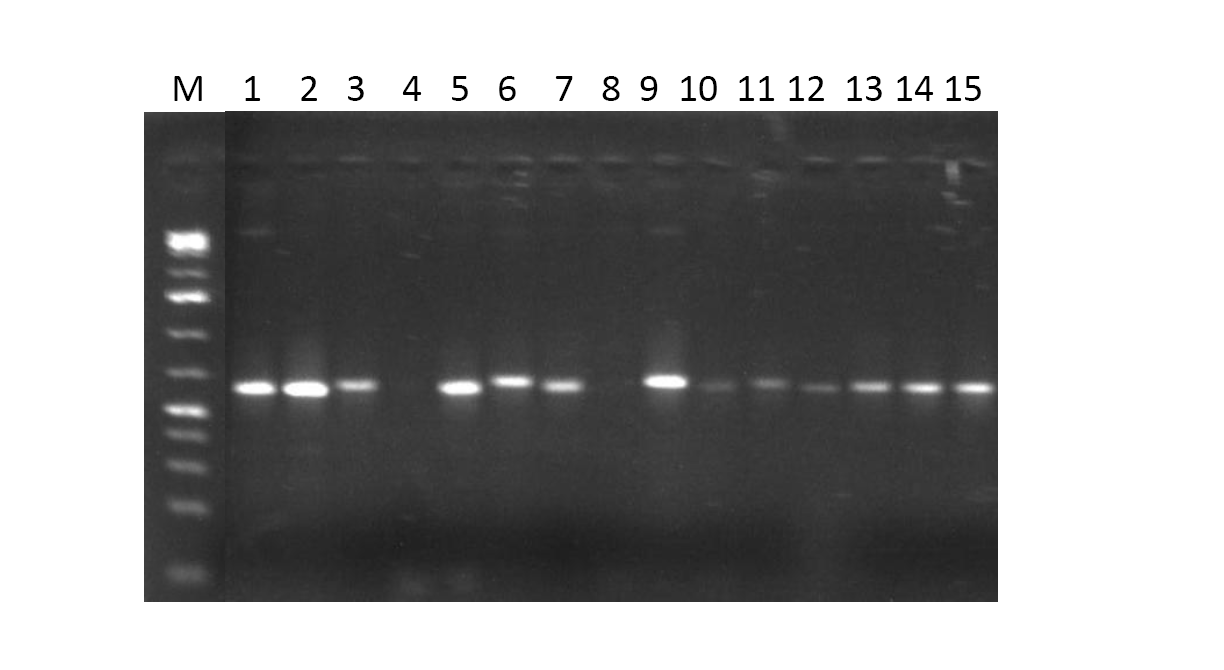 | Figure 1. 1.0 % Agarose gel electrophoresis of the bacterial genomic DNA samples |
The result in Figure 1 showed different band intensities. The DNA samples loaded in lane 4 and 8 had low intensity bands while other DNA samples had intense visible bands. The 16S rRNA gene of each of the bacterial isolates was amplified using suitable primer and the amplified 16S rRNA gene sequences (Figure 2-10) were then compared with the sequence in National Centre for Biotechnology Information (NCBI) gene bank database using Basic Local Alignment Search Tool (BLAST) search program. BLAST is a web based program that is able to align the search sequence to thousands of different sequences in a database and show the list of top matches. Figure 3 showed the alignment obtained from BLAST search program for the bacterial isolates. The alignment was performed by matching up each position of search sequence to each position of the sequences in the database. For each position, BLAST gave a positive score if the nucleotides matched, it also inserted gaps when performing the alignment. Each gap inserted has a negative effect on the alignment score, but with enough aligned nucleotides, the negative effect was overcome and the gap was accepted in the alignment (Figure 11). Table 1 showed the summary of the results of the sequences obtained. The BLAST result showed that isolates S71 was 91% similar to both E. coli strain S51 with accession number CP015995.1 and E. coli strain 268-78-1with accession number CP014092.1, isolate S177 was 91% similar to both E. coli strain BACO64 with accession number KU161315.1 and E. coli strain BAC031 with accession number KU161312.1, S31 was 91% similar to E. coli strain 268-78-1 with accession number CP014092.1, isolates S89 and S34 were both 91% similar to E. coli strain OZK2 with accession number KT440880.1, isolate S152 was 89% similar to E. coli strain C-XIB with accession number KJ806504.1, isolates S33 and S35 were 91% similar to E. fergusonii strain CGS24 with accession number KX034239.1 while S30 was 91% similar to E. coli strain S51 with accession number CP015995.1.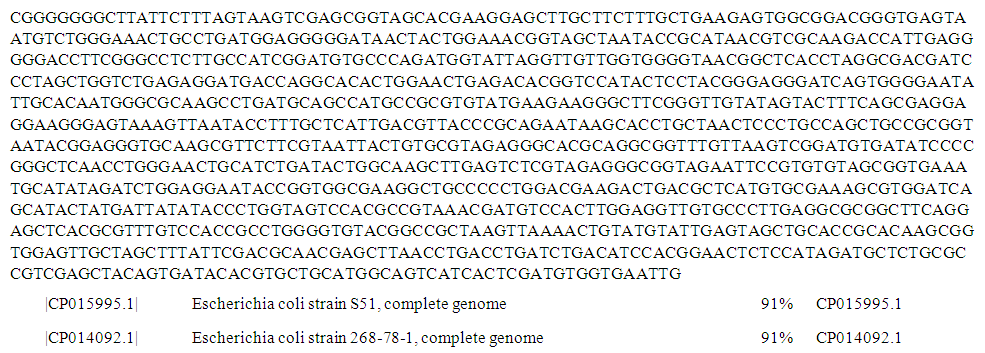 | Figure 2. Complete genome sequence of PCR product of 16S rRNA gene of isolate S71 from well water sample |
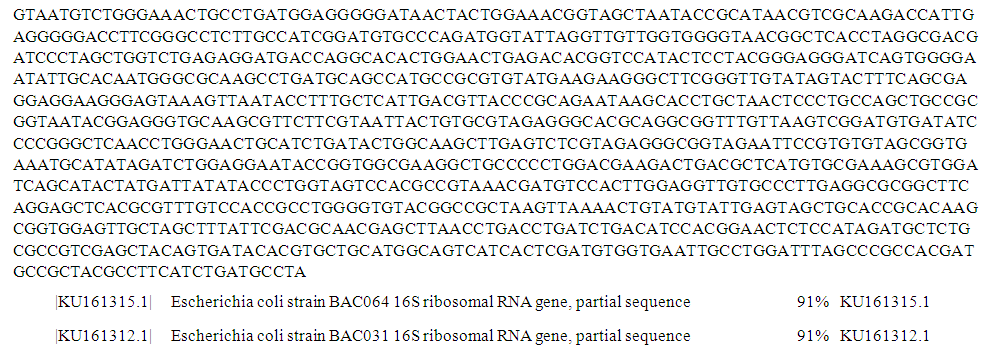 | Figure 3. Partial sequence of PCR product of 16S rRNA gene of isolate S177 from stream water sample |
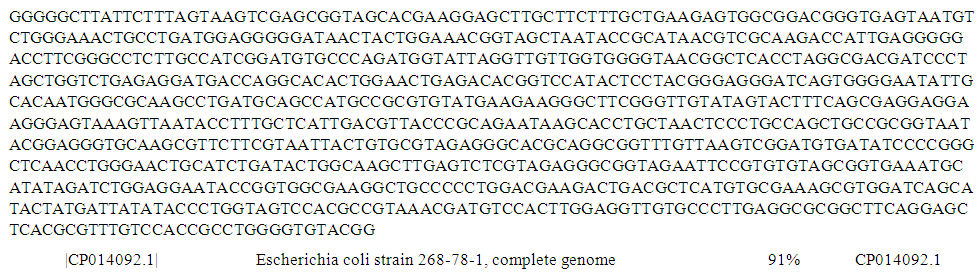 | Figure 4. Complete genome sequence of PCR product of 16S rRNA gene of isolate S31 from borehole water sample |
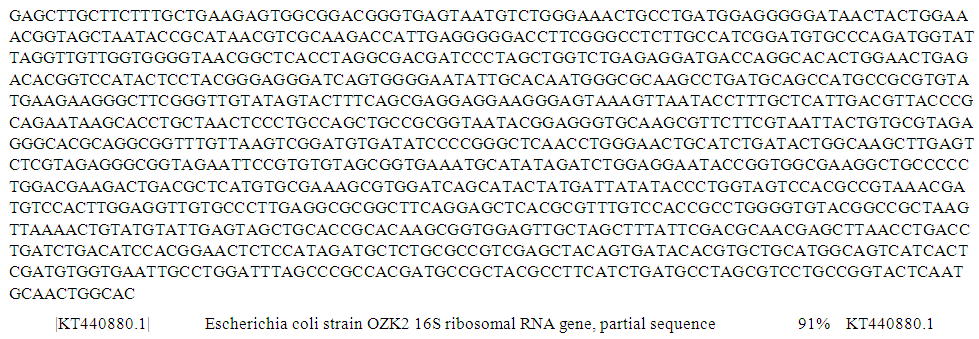 | Figure 5. Partial sequence of PCR product of 16S rRNA gene of isolate S89 from well water sample |
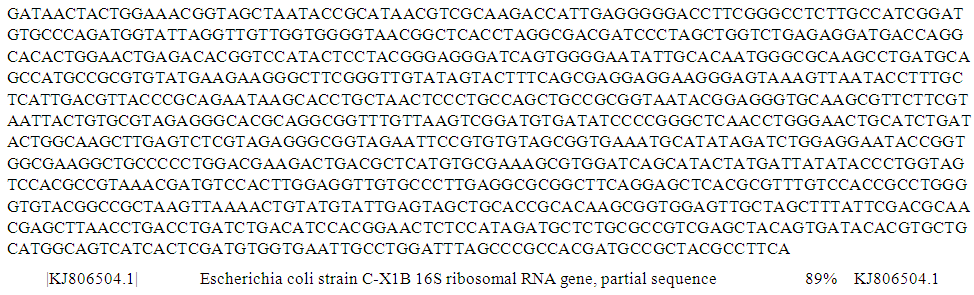 | Figure 6. Partial sequence of PCR product of 16S rRNA gene of isolate S152 from well water sample |
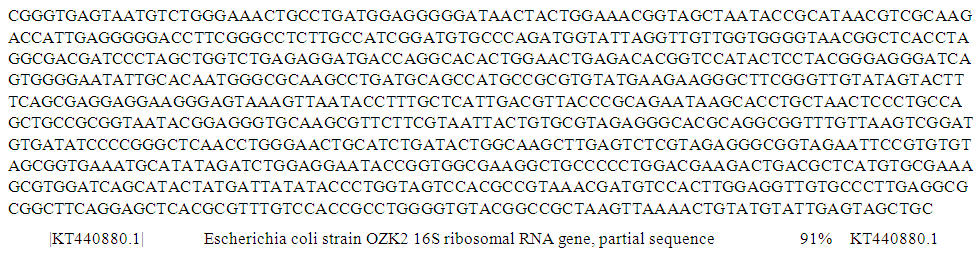 | Figure 7. Partial sequence of PCR product of 16S rRNA gene of isolate S34 from pipe-borne water sample |
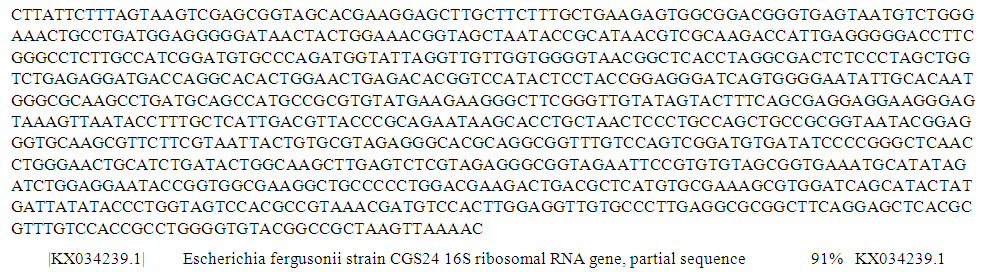 | Figure 8. Partial sequence of PCR product of 16S rRNA gene of isolate S33 from borehole water sample |
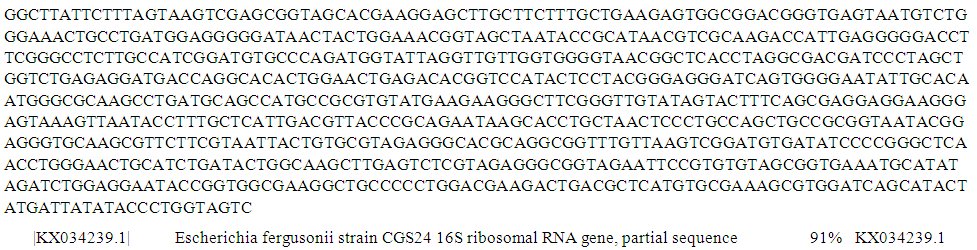 | Figure 9. Partial sequence of PCR product of 16S rRNA gene of isolate S35 from well water sample |
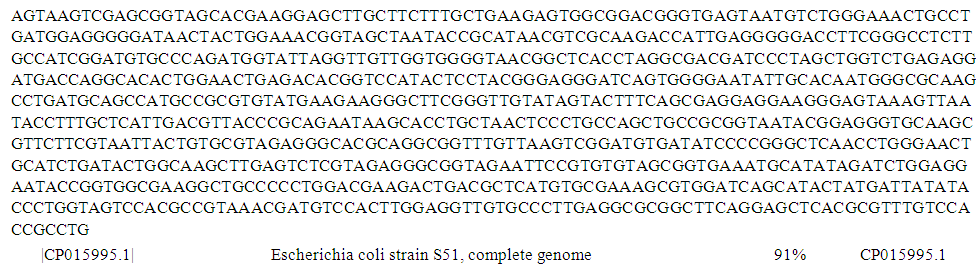 | Figure 10. Complete genome sequence of PCR product of 16S rRNA gene of isolate S30 from well water sample |
 | Figure 11. Alignment for the bacteria isolates |
Table 1. Molecular Identification of Bacterial Isolates from Drinking Water Samples in Ado-Ekiti and Environs using 16S rRNA 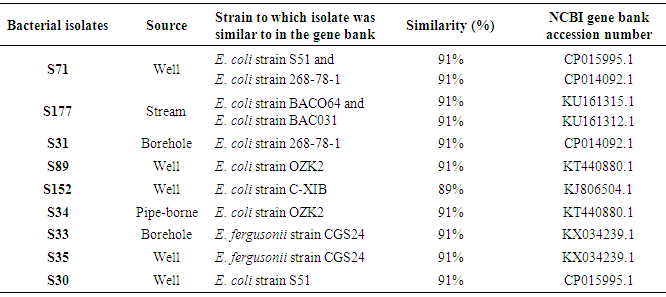 |
| |
|
Figure 12 showed the phylogenetic tree of the isolates which delineates the genetic relatedness of the different isolates from different drinking water sources. The phylogenetic tree showed two different clusters. The first cluster comprises S152, S177, S89, S71 which were identified as E. coli strain C-XIB, BAC064, OZK2, S51 respectively while the second cluster comprises S33, S31, S30, S34, S35 which were identified as E. fergusonii strain CGS24, E. coli strain 268-78-1, S51, OZK2, E. fergusonii strain CGS24 respectively. 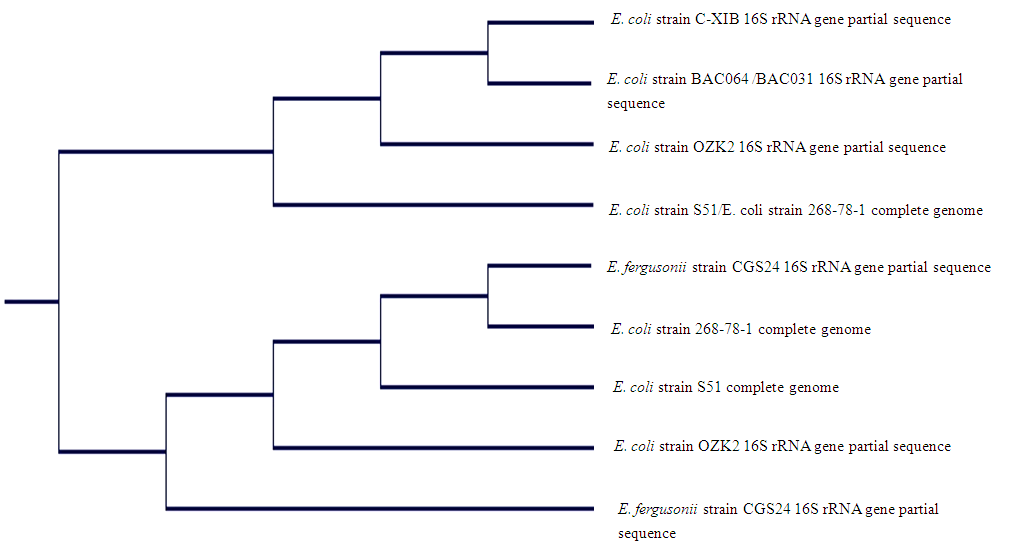 | Figure 12. Phylogenetic tree of identified isolates from drinking water sources in Ado-Ekiti and environs |
4. Discussion
The traditional identification of bacteria on the basis of phenotypic characteristics is not as reliable as recent identification which is based on genotypic methods. The ribosomal RNA sequence based analysis is an implicit and unique method to understand microbial diversity within and across a group and also to identify new strains (Magray et al., 2011). Bacterial species have at least one copy of the 16S rRNA gene containing highly conserved regions together with hyper variable regions, which is used for identification of new strains. The comparison of the bacterial 16S rRNA gene sequence with a known bacterial sequence in the database has emerged as a preferred genetic technique. Also, the sequence of the 16S rRNA gene has been widely used to estimate phylogenetic relationships among bacteria (Sujatha et al., 2012). The results of identification in this study showed an accurate and definitive identification of the isolates. This revealed that all the isolates were of Escherichia genus. While seven from nine isolates were identified as E. coli, two were identified as E. fergusonii. The study unveiled the shortcoming of traditional/conventional identification which is based on phenotypic characteristics. Furthermore, the inability to differentiate E. fergusonii from E. coli in the previous study also corroborates findings by Wragg et al. (2009). Their study showed that E. fergusonii isolates possess both some phenotypic and genotypic features linked to pathogenic E. coli and also support the evidence that strains of E. fergusonii may act as opportunistic pathogens. In another study by Walk et al. (2009), they also unveiled cryptic lineages of the genus Escherichia, which shared similar phenotypic traits, by using molecular tool.
5. Conclusions
To the best of our knowledge, this study reports the incidence of E. fergusonii in drinking water sources in Nigeria for the first time. Therefore, the use of 16S rRNA gene sequencing in this study has contributed immensely in giving a decisive differentiation and identification of similar bacterial isolates belonging to the same genus.
ACKNOWLEDGMENTS
We acknowledge the technical supports provided by Dr. O. O. Oyelakin of Department of Molecular Biology, Federal University of Agriculture, Abeokuta, Ogun State, Nigeria and the useful contributions of Dr. O. M. David, Microbiology Department, Ekiti State University, Ado-Ekiti.
References
[1] | H. S. Abdul (2010). Isolation and identification of pathogenic bacteria from drinking water of Khairpur, Sukkur and Rohri. Ph. D Thesis in Microbiology, Shah Abdul Latif University Khairpur. |
[2] | A. A. Akinyemi and O. O. Oyelakin (2014). Molecular characterization of bacteria isolates from farm-raised catfish Clarias garipinus (Burchell, 1822). British Microbiology Research Journal, 4(12), 1345-1352. |
[3] | A. Chaudhury, G. Nath, A. Tikoo and S. C. Sanyal (1999). Enteropathogenicity and antimicrobial susceptibility of new Escherichia spp. J. Diarrhoeal Dis. Res. 17, 85–87. |
[4] | J. J. Farmer, B. R. Davis, F. W. Hickman-Brenner, A. McWhorter, G. P. Huntley-Carter, M. A. Asbury, C. Riddle, H. G. Wathen-Grady, C. Elias, G. R. Fanning, A. G. Steigerwalt, C. M. O’Hara, G. K. Morris, P. B. Smith and D. J. Brenner (1985). Biochemical identification of new species and biogroups of Enterobacteriaceae isolated from clinical specimens. Journal of Clinical Microbiology, 21, 77-8 1. |
[5] | G. Foster, J. Evans, M. Tryland and S. Hollamby (2010). Use of citrate adonitol agar as a selective medium for the isolation of Escherichia fergusonii from a captive reindeer herd. Veterinary microbiology, 144(3), 484-486. |
[6] | J. Freney, F. Gavini, C. Ploton, H. Leclerc and J. Fleurtte (1987). Isolation of E.fergusonii from a patient with septicemia in France. Eur J Clin Microbiol Infect Dis 6, 78. |
[7] | A.Y. Gaafar, A. M. Younes, A. M. Kenawy, W. S. Solimanm and L. A. Mohamed (2015). Escherichia fergusonii: A New Emerging Bacterial Disease of Farmed Nile Tilapia (Oreochromis niloticus). Global Veterinaria, 14 (2), 268-273. |
[8] | Y. B. Gideon, F. B. Fatoye and J. I. Omada (2013). Quality assessment of physico-chemical characteristics of Okura River, Kogi State, Nigeria. International Journal of Science and Technology, 2(12), 891- 899. |
[9] | S. Kalpana, A. I. Bagudo and A. A. Aliero (2011). Microbiological analysis of sachet drinking water marketed at two sites in Aliero, Kebbi State, Nigeria. Continental Journal of Microbiology, 1(5), 29-36. |
[10] | M. S. Magray, A. Kumar, A. K. Rawat and S. Srivastava (2011). Identification of Escherichia coli through analysis of 16S rRNA and 16S-23S rRNA internal transcribed spacer region sequences. Bioinformation, 6(10), 370–371. |
[11] | A. F. Maheux, D. K. Boudreau, M. G. Bergeron and M. J. Rodriguez (2014). Characterization of Escherichia fergusonii and Escherichia albertii isolated from water. Journal of Applied Microbiology, 117(2), 1-15. |
[12] | L. L. Nwidu, B. Oveh, T. Okonye and N. A. Vakosen (2008). Assessment of the water quality and prevalence of water borne diseases in Amasoma, Niger Delta, Nigeria. African Journal of Biotechnology, 7(17), 2993-2997. |
[13] | S. T. Odonkor and J. K. Ampofo (2013). Escherichia coli as an indicator of bacteriological quality of water: an overview. Microbiology Research, 4(2), 5 – 11. |
[14] | J. Y. Oh, M. S. Ka ng, B. K. An, E. G. Shin, M. J. Kim, J. H. Kwon, and Y. K. Kwon (2012). Isolation and epidemiological characterization of heat-labile enterotoxin-producing Escherichia fergusonii from healthy chickens. Veterinary Microbiology. 160(1-2), 170-175. |
[15] | M. E. Ohanu, I. P. Udoh and C. I. Eleazer (2012). Microbiological Analysis of Sachet and Tap Water in Enugu State of Nigeria. Advances in Microbiology, 2, 547-551. |
[16] | B. M. Olowe, J. O. Oluyege and O. Famurewa (2015). Determination of multiple antibiotic resistance index of E. coli isolated from the drinking water sources in Ado-Ekiti and environs, Nigeria. International Journal of Scientific research and Engineering Studies, 2(10):56-62. |
[17] | B. M. Olowe, J. O. Oluyege and O. Famurewa (2016). Prevalence of waterborne diseases and microbial assessment of drinking water quality in Ado-Ekiti and its environs, Southwestern, Nigeria. British Microbiology Research Journal, 12 (2), 1-13. |
[18] | J. O. Oluyege, A. C. Dada and A. T. Odeyemi (2009). Incidence of multiple antibiotic resistant Gram-negative bacteria isolated from surface and underground water sources in south western region of Nigeria. Water Science and Technology, 59 (10). 1929–1936. |
[19] | O. Oyedeji, P. O. Olutiola and M. A. Moninuola (2010). Microbiological quality of packaged drinking water brands marketed in Ibadan metropolis and Ile-Ife city in South Western Nigeria. African Journal Microbiology Resources, 4, 96-102. |
[20] | J. B. Patel (2001). 16S rRNA gene sequencing for bacterial pathogen identification in the clinical laboratory. Mol. Diagn. 6, 313-321. |
[21] | A. Rompre, P. Servais, J. Baudart, M. deRoubin and P. Laurent (2002). Detection and enumeration of coliforms in drinking water: current methods and emerging approaches. Journal of Microbiology Methods, 49, 31-54. |
[22] | K. Simmons, H. Rempel, G. Block, V. Forgetta, R. Vaillancourt, Jr. F. Malouin, E. Topp, P. Delaquis and M. S. Diarra (2014). Duplex PCR methods for the molecular detection of Escherichia fergusonii isolates from broiler chickens. Appl Environ Microbiol, 80(6), 1941-1948. |
[23] | P. Sujatha, N. B. Kumar and V. Kalarani (2012). Isolation, characterization and molecular identification of bacteria from tannery effluent using 16S rRNA sequencing. Current Biotica 6(2), 198-207. |
[24] | O. E. Sunday and C. M. Innocent (2014). Physicochemical and microbiological analysis of water bodies in Uturu, Abia State-Nigeria. Asian Journal of Natural and Applied Sciences, 1 (4), 158-165. |
[25] | S. T. Walk, E. W. Alm, D. G. Gordon, J. L. Ram, G. A. Toranzos, J. M. Tiedje, and T. S. Whittam (2009). Cryptic lineages of the genus Escherichia. Applied and Environmental Microbiology, 75(20), 6534–6544. |
[26] | World Health Organisation (2006). Guidelines for drinking water quality. First addendum to third edition, volume 1 recommendations. http://www.who.int/water-sanitation-health/dwq/gdwq3rev/en/. |
[27] | P. Wragg, R. M. La Ragione, A. Best, R. Reichel, M. F. Anjum, M. Mafura and M. J. Woodward (2009). Characterisation of Escherichia fergusonii isolates from farm animals using an Escherichia coli virulence gene array and tissue culture adherence assays. Research in Veterinary Science, 86 (1), 27-35. |