Hana Kubatova1, Eva Zemanova1, Karel Klouda1, Karel Bilek2, Jana Kadukova3
1State Office for Nuclear Safety, Senovazne namesti 9, 110 00 Praha 1, Czech Republic
2National Institute for Nuclear, Chemical and Biological Protection, Kamenna 71, 262 31 Milin, Czech Republic
3Technical University of Kosice, Faculty of Metallurgy, Letna 9, 042 00 Kosice, Slovak Republic
Correspondence to: Eva Zemanova, State Office for Nuclear Safety, Senovazne namesti 9, 110 00 Praha 1, Czech Republic.
Email: |  |
Copyright © 2012 Scientific & Academic Publishing. All Rights Reserved.
Abstract
The contribution deals with preparation of C60 fullerene derivatives (oxo derivative, bromo derivative, hydrolyzed bromo derivative, bromo-chloro derivative), their identification and pilot testing of their biological effects on unicellular organisms. The contribution describes effects of C60 fullerene derivatives, both on prokaryotic organisms (bacteria, cyanobacteria) and eukaryotic organisms (algae) and assesses their potential use as biocides.
Keywords:
Fullerene C60, Fullerene Derivatives, Antimicrobial, Bacteria, Cyanobacteria, Algae, Biocide Effect
Cite this paper: Hana Kubatova, Eva Zemanova, Karel Klouda, Karel Bilek, Jana Kadukova, Effects of C60 Fullerene and its Derivatives on Selected Microorganisms, Journal of Microbiology Research, Vol. 3 No. 4, 2013, pp. 152-162. doi: 10.5923/j.microbiology.20130304.05.
1. Introduction
Microorganisms represent an indispensable component of all ecosystems. They are at the beginning of food chains, participate in degradation of biomass and formation of soils and play an important role in biogeochemical cycles. As shown by recent research, microorganisms may be significantly influenced by nanomaterials. In addition to antimicrobial effects of nanoparticles of silver, titanium dioxide or zinc oxide, an ever increasing attention has been paid to fullerenes and their derivatives.Thanks to nanotechnologies, buckminsterfullerenes (C60) are becoming more and more available. They are offered on the market as strong antioxidant agents, they are applied in cosmetics, sunscreens and lotions[1, 2]. From those preparations they may get into the environment and influence microorganisms. Although fullerene C60 is only little soluble in water, it may form water suspensions with stable nano-aggregates nC60. Solubility of C60 fullerene in water is strongly affected by its derivatization[3], at the same time, the derivatization also influences its toxicity or antimicrobial properties[4, 5].The effects of water suspensions of C60 and its derivatives on microorganisms were investigated in numerous studies. Antibacterial effects were reported for C60[3, 6], itshydroxylated derivatives[4]; amino-derivatives[5] orcarboxyfullerenes[5]. The most frequently investigated bacteria were E. coli and B. subtilis[4, 5, 6, 7, 8, 9]. Results of the studies indicate that the level of antimicrobial effects depends on a specific derivative of C60 fullerene, on a selected testing organism and on environmental conditions (pH, electric charge, salinity).Negative effects of C60 fullerene and its derivatives on specific groups of living organisms could be used for biocide purposes in water treatment[10] or decontamination. Therefore we have conducted experiments in which we wanted to evaluate potential biocide effects of C60 fullerene and its derivatives on selected microorganisms. The model organisms included both prokaryotic and eukaryotic microorganisms. All the selected microorganisms have cell wall of various compositions on their surfaces. From among the factors that may influence antimicrobial effects of fullerenes, we have focused on concentration and on incubation time. Neither the size nor the structure of the generated nanoparticles or their aggregates were measured or characterized.
2. Materials and Methods
2.1. Preparation of Fullerene Derivatives (Simplified Description)
The pristine fullerene C60 with 99.5% purity was obtained from SES Research, Houston, USA.The oxoderivative of fullerene – C60oxiIt was prepared by a reaction of 500 mg of C60 fullerene with 600 ml of 35 % peracetic acid[11]. After 30 minutes of continual stirring at the laboratory temperature we obtained a dark brown dispersion that was subsequently evaporated by heating to ca. 100 ml. Clayey modified fullerene was separated by centrifugation (5 min, 3 500 rev./min) and then washed with small quantities of water until the neural pH was achieved. The presence of hydroxyl and carboxyl groups was demonstrated by FTIR spectrometry.The bromoderivative of fullerenIt was prepared by a reaction of 27.5 ml (85.3g) of liquid bromine with 4 g of C60 fullerene[12]. The obtained mixture was agitated at the laboratory temperature for 72 hours. The excess bromine was subsequently removed by drying at 75℃ until a constant weight was achieved (ca. 24 hours).The resulting product was 9.9 g of a green-brown substance. Based on the weight increase its anticipated average composition was C60Br14. This composition was confirmed by elemental analysis of bromine. The hydrolyzed bromoderivative of fullerenIt was subsequently prepared from the bromoderivative for experiments on green algae, and obtained by exposure of the bromoderivative to distilled water for 24 hours and by its subsequent drying.The bromo-chloro derivative of fullerenIt was obtained by a mixing of 300 ml of tetrachloromethane and 5g of the prepared bromo derivative C60Br14[12]. The mixture was percolated with gaseous chlorine released from a pressure cylinder. The mixture was in kept for three days (during the incubation time additional 100 ml of tetrachloromethane was replenished). After evaporating the tetrachloromethane in a rotation evaporator the resulting red-brown powder was dried at 75℃ until a constant weight was achieved, while the total product was 4.65 g. Based on a calculation and elemental analysis the summary formula was determined as C60Br10Cl4 .
2.2. Effects of Fullerenes on Prokaryotes
2.2.1. Effects on Bacteria
In order to obtain preliminary information about the effects of C60 fullerene and its derivatives on selected bacterial strains a one-off pilot measurement was performed with three types of bacteria, Escherichia coli (gram-negative), Staphylococcus aureus (gram positive) and Bacillus cereus (gram positive, sporulating). 100 μg of C60 fullerene or its derivatives (C60oxi, C60Br14, C60Br10Cl4) were weighted into test tubes with 10 ml of bacterial suspension in physiological solution with the concentration ca. 5x104 CFU/ml (measured by standard counting on Petri dishes); the resulting concentration of fullerenes was 10 mg/l). The mixture was stirred and left to incubate at the laboratory temperature, while the content of the test tubes was stirred. After 30 and 90 minutes of exposure to fullerenes a standard counting on Petri dishes was used to determine concentration of the bacterial suspension/viable cells.The preliminary results shown in Table 1 indicate that an antibacterial effect may be expected for bromoderivatives and bromo-chloro derivatives (results marked by grey fields). Other experiments followed based on the obtained preliminary results, using all the previously used types of bacteria plus Yersinia enterocolitica (gram negative).All the selected types of bacteria were cultivated on TSA agar (Oxoid, United Kingdom) at 37°C for 24 hours. The obtained colonies or spores were scraped off with a sterile inoculation loop and suspended in physiological solution. Sporulation of B. cereus was performed on BBLTM AK Agar #2 (Sporulating agar) (Becton Dickinson, France) at the room temperature for 14 days. During the preparation of spores the remaining vegetative cells were destructed by heating to 62.5°C for 15 minutes. The concentration of viable cells and spores (in CFU/ml) was determined by standard counting on Petri dishes with TSA agar after the cultivation at 37°C for 24 hours (Fig. 1). The obtained bacteria were diluted with physiological solution to the resulting concentration 1x105 CFU/ml and subsequently exposed to experimental conditions.Table 1. The resulting values in cfu/ml after the incubation with C60 fullerene and its derivatives 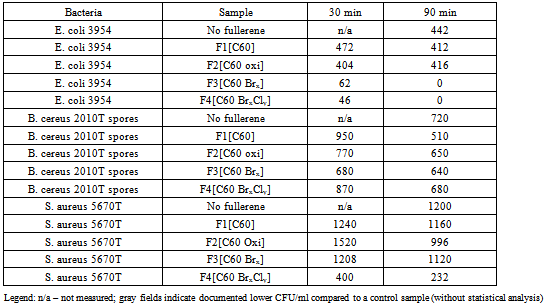 |
| |
|
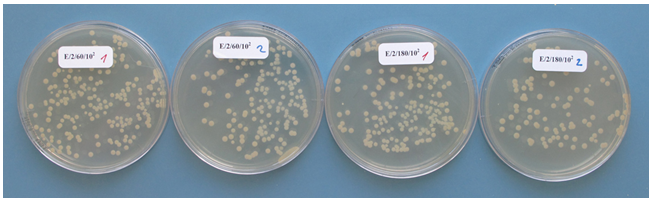 | Figure 1. Examples of growing colonies of viable cell on TSA agar |
In agreement with the conditions to which the bacteria were exposed in the preliminary tests and in agreement with data in the published works[9, 13] the concentrations of fullerenes used for the experiments were 1 mg/l and 10 mg/l. The bacteria were exposed to those fullerene concentrations for 60 min and 180 min. Since C60 fullerene and some of its derivatives are very little soluble in water the test tubes were in the course of incubation placed on a stirrer. After 60 and 180 minutes 100 μl of sample was taken from each test tube. Each sample was diluted three times and 100 μl was subsequently spread on a Petri dish with TSA agar. The samples were then cultivated at 37°C for 24 hours. The resulting concentration of viable cells was determined by standard counting on Petri dishes (CFU/ml).The experiments were performed three times for all the concentrations and all the times. The individual measurements were statistically evaluated using the Student’s t-test program with the confidence interval 95% (the confidence interval is shown in the diagrams as a line segment) - Fig. 2-7. A suspension of the respective bacteria in physiological solution was used as a control sample.
2.2.2. Effects on Cyanobacteria (Blue-green Algae)
Testing water was from a fire reservoir in the municipality Milin near Pribram. The water contained mostly blue-green algae, particularly the Microcystis genus (Microcystisflos-aquae, Microcystis aeruginosa, Microcystis wesenbergii; the concentration of 1 213 333 cells /ml was determined by an accredited method under ČSN 75 77171), the Anabaena genus was less abundant (its concentration was 84 490 cells /ml). The sample also contained green algae in small quantities (Chlorophyta). The quantity of blue-green algae (or phytoplankton) in the water sample was determined at the beginning of testing with an accredited method under ČSN ISO 102602 as a concentration of chlorophyll-a at 702.5 µg/l.100 ml water samples were placed into bottles. Quantities of C60 fullerene or its derivatives (C60oxi, C60Br14, C60Br10Cl4) added to the samples were determined to achieve the resulting concentrations in the samples of 5, 10, 50, 100 and 500 mg/l. Two bottles were used as control samples without treatment. The samples were left exposed to light at 23.5 – 25.9°C. Concentration of chlorophyll-a was measured in the samples 24 hours later.
2.2.3. Effects of Fullerenes on Eukaryotic Organisms
Green microscopic algae Chlorella kessleri was selected as a representative of eukaryotic organisms. The first experiment investigated effects of concentration of the oxo derivative on individual phases of algae growth (adaptation phase, exponential growth phase, stationary phase and death phase). Solutions were prepared with concentrations of 13.3 mg/l, 26.7 mg/l and 40 mg/l. The solutions were inoculated with the algae culture and cells in 1 ml of solutions with selected concentrations were counted during the following 10 days.
3. Results and Discussion
3.1. Effects on Bacteria
Diagrams in Fig. 2 – 5 indicate that the individual types of bacteria are affected by presence of fullerenes differently. The effect of fullerenes was the lowest in case of Y. enterocolitica and spores of B. cereus; on the contrary, the effect was the highest on E. coli, which is e.g. for C60oxi in conflict with some published results[5]. Since fullerene derivatives influenced representatives of both gram positive (S. aureus) and gram negative (E. coli) bacteria the effect was not dependent on the cellular wall structure. According to[9], water suspensions of C60 fullerene do not disturb integrity of the bacterial cell but they cause oxidizing stress, loss of membrane potential and they prevent respiration. Based on the obtained, it possible to assume that different C60 derivatives influence membrane proteins responsible for membrane potential with different intensities.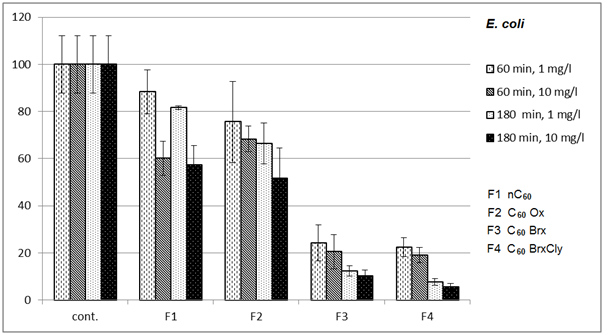 | Figure 2. Evaluation of effects of C60 fullerene and its derivatives on E. coli at 37°C; for exposure times and fullerene concentrations; y axis - concentrations reading in CFU/ml recalculated to percents. (cont. = control samples) |
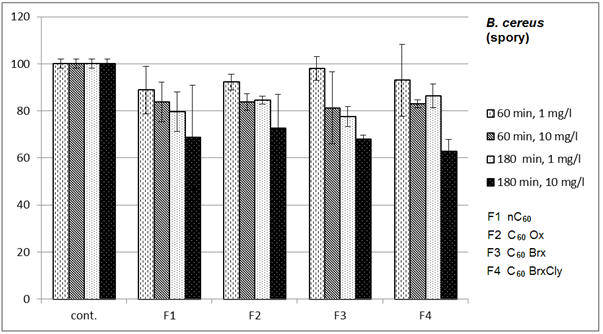 | Figure 3. Evaluation of effects of C60 fullerene and its derivatives on spores of B. cereus at 37°C; for exposure times and fullerene concentrations; y axis - concentrations reading in CFU/ml recalculated to percents. (cont. = control samples) |
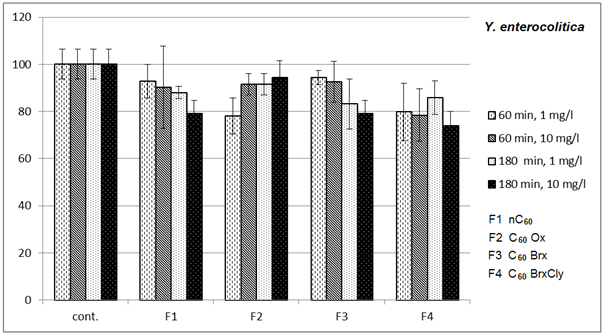 | Figure 4. Evaluation of effects of C60 fullerene and its derivatives on Y. enterocolitica at 37°C; for exposure times and fullerene concentrations; y axis - concentrations reading in CFU/ml recalculated to percents. (cont. = control samples) |
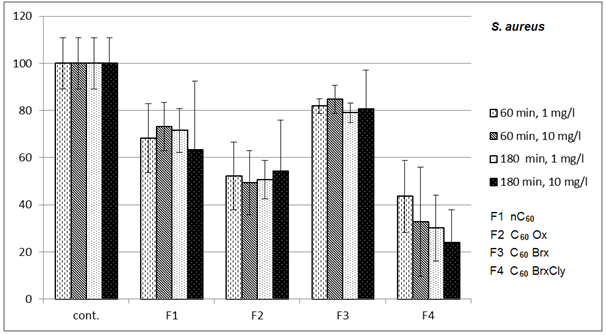 | Figure 5. Evaluation of effects of C60 fullerene and its derivatives on S. aureus at 37°C; for exposure times and fullerene concentrations; y axis - concentrations reading in CFU/ml recalculated to percents. (cont. = control samples) |
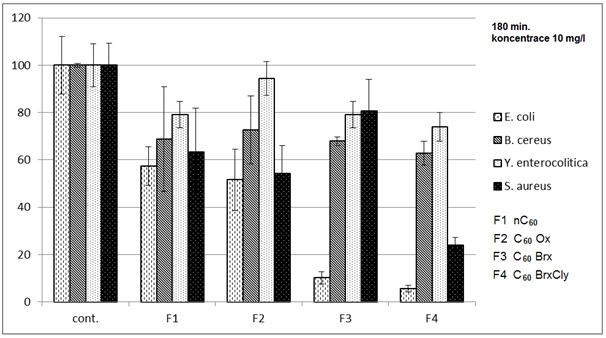 | Figure 6. Comparison of effects of fullerenes with the concentration of 10 mg/l on individual types of bacteria for 80 min at 37°C; y axis - concentrations reading in CFU/ml recalculated to percents. (cont. = control samples) |
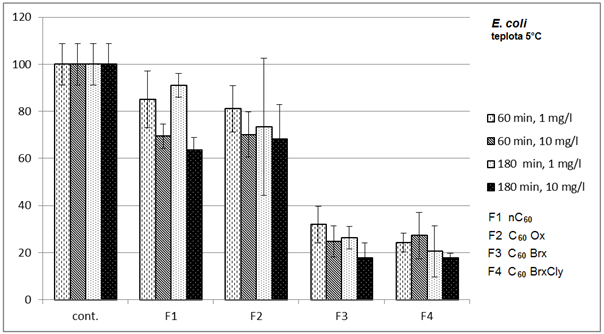 | Figure 7. Comparison of effects of both the selected concentrations of fullerenes on E.coli at 5°C for 60 min and 180 min; y axis - concentrations reading in CFU/ml recalculated to percents. (cont. = control samples) |
3.2. Effects on Cyanobacteria (Blue-green Algae)
A significant decrease of concentration of chlorophyll-a was measured in the sample containing 500 mg/l of bromoderivative (C60Br14) and in the sample containing 500 mg/l of bromo-chloro derivative (C60Br10Cl4). Microcystis sp. cells were counted in those two samples and the values were compared with the control sample. (Fig. 8 and 9). The counting failed to demonstrate a significant decrease of the number of blue-green algae cells. The fluctuation of value may be attributed only to the uncertainty of the method. Microcystis sp. cells were inspected under optical microscope with magnification 200 x, 400 x and 1000 x. In comparison with the control sample no deformations or other significant differences were found. Therefore the results failed to provide a reliable evidence of whether the decrease of chlorophyll-a concentration was caused by a decrease of algae or blue-green algae or both.Chlorophyll-a concentration was measured after 72 hours in the samples with the highest concentrations of C60 fullerene and its derivatives (100 mg/l and 500 mg/l) and the obtained values were compared with the control sample. A decreased content of chlorophyll-a was measured again only in the two above mentioned samples (Fig. 10 and 11).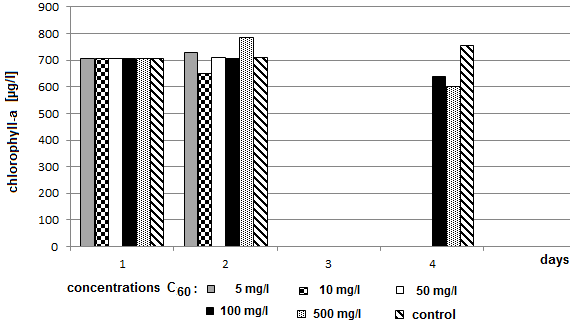 | Figure 8. Dependence of concentration of chlorophyll-a on the exposure time and on concentrations of fullerene C60 (axis y: chlorophyll-a; axis x: days) |
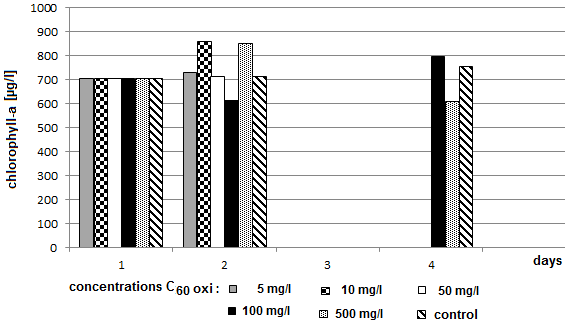 | Figure 9. Dependence of concentration of chlorophyll-a on the exposure time and on concentrations of fullerene derivative C60oxi (axis y: chlorophyll-a; axis x: days) |
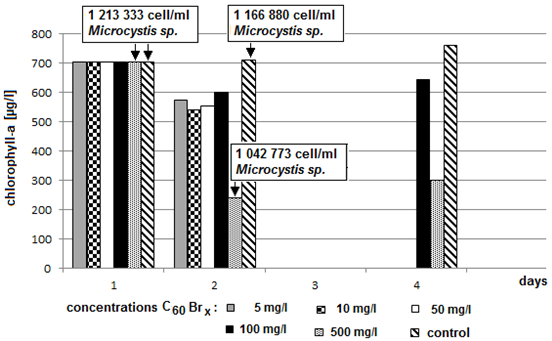 | Figure 10. Dependence of concentration of chlorophyll-a on the exposure time and on concentrations of fullerene derivative C60Br14 (axis y: chlorophyll-a; axis x: days) |
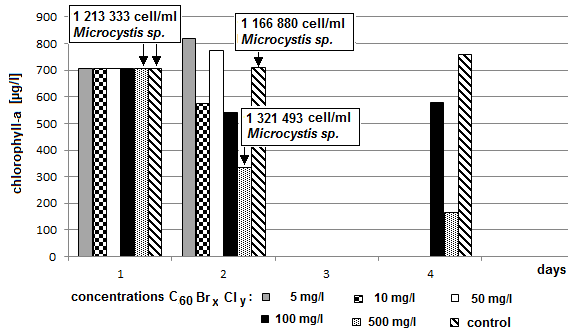 | Figure 11. Dependence of concentration of chlorophyll-a on the exposure time and on concentrations of fullerene derivative C60BrxCly (axis y: chlorophyll-a; axis x: days) |
3.3. Effects of Fullerenes on Eukaryotic Organisms
The diagram in Fig. 12 indicates that all the three selected concentrations of the oxo derivative had a negative effect on the algae growth. For concentrations of 13.3 mg/l and 40 mg/l the phase of adaptation extended by one day. On the contrary, the phase of exponential growth was shorter for both the higher concentrations - for algae cultivated in the concentration of 26.7 mg/l the exponential growth phase lasted two days, for the concentration of 40 mg/l it lasted only one day and subsequently the culture entered a stationary phase. The algae culture cultivated in the concentration of 26.7 mg/l demonstrated a fast onset of the death phase. Quite surprising was the negative effect of the concentration of 26.7 mg/l, in comparison with the concentration of 40 mg/l, which appeared after the fourth day of cultivation.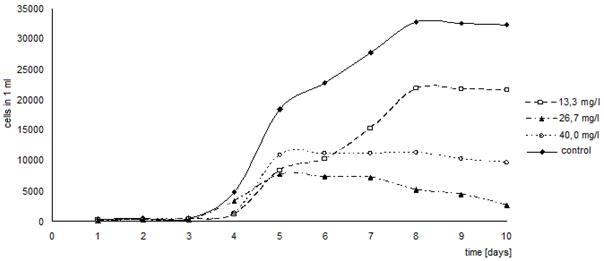 | Figure 12. Effects of the oxoderivative on the growth of Chlorella kessleri algae. (axis y: number of cells in 1 ml; axis x: time (days)) |
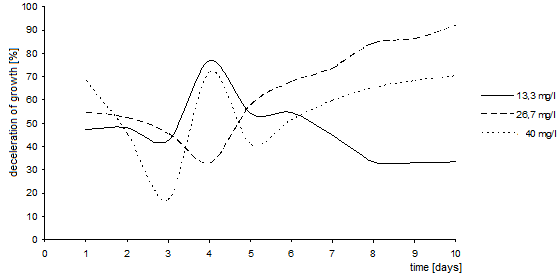 | Figure 13. Deceleration of growth of Chlorella kessleri algae caused by various concentrations of the oxoderivative. (axis y: growth deceleration (%); axis x: time (days)) |
Negative effects of the oxoderivative on the growth of Chlorella kessleri algae may be expressed also with a dependence of the growth deceleration (in percents) on the exposure time. The diagram in Fig.13 indicates that the concentrations of 26.7, 40 and 13.3 mg/l deceleration the growth 91.9%, 70.2% and 33.3% respectively.Adaptation of the cells probably occurred in the lowest concentration of the oxoderivative (13.3 mg/l) because after the 4th day the decrease of the algae growth slowed down. On the contrary, both the higher concentrations (26.7 and 40 mg/l) inhibited the growth of algae with the advancing time ever more and no stabilization was observed, not even after 10 days. Therefore it is possible that the entire culture may die out after a sufficiently long period of time.Subsequently, experiments were performed to compare effects of the same concentrations of various C60 fullerene derivatives on algae growth. Solutions of the oxoderivative, bromoderivative and hydrolyzed bromoderivative were separately prepared with the concentration of 10 mg/l.The diagram in Fig. 14 indicates that the algae growth was significantly affected with the bromoderivative (76.7%) more than hydrolyzed bromoderivative (22.5%) and oxoderivative (13.4%). Negative effects of thebromoderivative on the algae growth was demonstrated also by a change of the cell color: during the first 4 days of cultivation the cells were pink-brown and only subsequently they turned green which suggests a partial adaptation of the cells to presence of the bromoderivative. The adaptation of the cells to presence of the bromoderivative is also evidenced by the result showed that after the 4th day the growth did not slow down any further (Fig.14 and 15).Algae cells cultivated in presence of the oxoderivative and the hydrolyzed bromoderivative did not demonstrate any changes in the coloring of the culture, showing only the green chlorophyll color. The diagram in Fig. 15 indicates algae cells adapted to presence of the oxoderivative and the hydrolyzed bromoderivative, as early as in the first day of the cultivation.Effects of C60 fullerene derivatives were investigated also by means of tests on Petri dishes. Individual derivatives in crystalline form were placed on Petri dishes with nutrient agar and inoculated algae. The algae demonstrated no growth in the proximity of crystals of the derivatives (Fig. 16A). It is interesting to note that when we dissolved crystals of the derivatives in water and add the solution to the agar, no change of the algae growth was observed (Fig. 16B).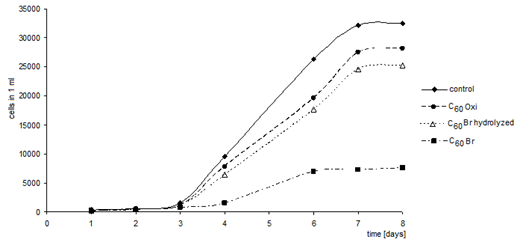 | Figure 14. Growth curves of Chlorella kessleri algae in presence of C60 fullerene derivatives. (axis y: number of cells in 1 ml; axis x: time (days)) |
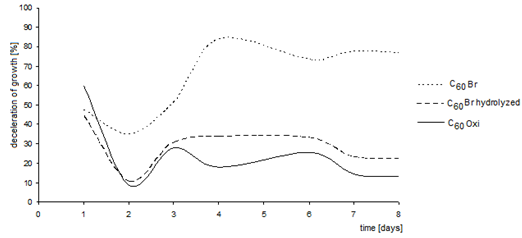 | Figure 15. Inhibition of the algae growth in presence of various C60 fullerene derivatives. (axis y: growth deceleration (%); axis x: time (days)) |
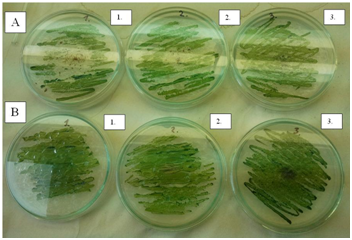 | Figure 16. Effect of C60 derivatives on algae inoculated into nutrient agar. Results of the tests on agar: A – after adding derivatives in crystalline form, B – after adding suspension of crystals of the derivatives in distilled water (1 - bromoderivative, 2 – hydrolyzed bromoderivative, 3 – oxoderivative) |
4. Conclusions
The described experiments demonstrate that, despite the diverse methods, all the investigated C60 fullerene derivatives had negative effects on the selected groups of unicellular organisms. The most noticeable negative effect was found for the bromoderivative, while the oxoderivative had the smallest effect. This fact suggests an opportunity to use the bromoderivative as a biocide in laboratories, however, its release into the environment shall be prevented or it shall be degraded.
Notes
1. ČSN 75 7717 (757717) Water quality - Determination of planctonic cyanobacteria2. ČSN ISO 10260 (757575) Water quality - Measurement of biochemical parameters - Spectrometric determination of the chlorophyll-a concentration
References
[1] | Usenko C. Y., Harper S. L., Simonich M. T., Tanguay R. L.: Fullerene C60 Toxicology. Handbook of Nanophysics: Nanomedicine and Nanorobotics, 2010, CRC Press, ISBN: 978-1-4200-7549-6 |
[2] | Boxall A. B., Tiede K., Chaudhry Q.: Engineered nanomaterials in soils and water: how do they behave and could they pose a risk to human health? Nanomedicine (Lond). 2007 Dec; 2(6):919-27. |
[3] | Fortner J. D., Lyon D. Y., Sayes C. M., Boyd A. M., Falkner J. C., Hotze E. M., Alemany L. B., Tao Y. J., Guo W., Ausman K. D., Colvin V. L., Hughes J. B.: C60 in water: nanocrystal formation and microbial response. Environ Sci Technol. 2005 Jun 1;39(11):4307-16. |
[4] | Aoshima H., Kokubo K., Shirakawa S., Ito M., Yamana S., Oshima T.: Antimicrobial activity of fullerenes and their hydroxylated derivatives. Biocontrol Sci. 2009 Jun; 14(2):69-72. |
[5] | Tang Y. J., Ashcroft J. M., Chen D., Min G., Kim C. H., Murkhejee B., Larabell C., Keasling J. D., Chen F. F.: Charge-associated effects of fullerene derivatives on microbial structural integrity and central metabolism. Nano Lett. 2007 Mar; 7(3):754-60. |
[6] | Tsao N., Luh T. Y., Chou C. K., Chang T. Y., Wu J. J., Liu C. C., Lei H. Y.: In vitro action of carboxyfullerene. J Antimicrob Chemother. 2002 Apr; 49(4):641-9. |
[7] | Kang S., Mauter M. S., Elimelech M.: Microbial cytotoxicity of carbon-based nanomaterials: implications for river water and wastewater effluent. Environ Sci Technol. 2009 Apr 1; 43(7):2648-53. |
[8] | Lyon D. Y., Brunet L., Hinkal G. W., Wiesner M. R., Alvarez P. J.: Antibacterial activity of fullerene water suspensions (nC60) is not due to ROS-mediated damage. Nano Lett. 2008 May; 8(5):1539-43. doi: 10.1021/nl0726398. |
[9] | Lyon D. Y., Alvarez P. J.: Fullerene water suspension (nC60) exerts antibacterial effects via ROS-independent protein oxidation. Environ Sci Technol. 2008 Nov 1;42(21):8127-32. |
[10] | Lyon D. Y, Brown D. A., Alvarez P. J.: Implications and potential applications of bactericidal fullerene water suspensions: effect of nC60 concentration, exposure conditions and shelf life. Water Sci Technol. 2008; 57(10):1533-8. |
[11] | Zemanova E., Klouda K.: Preparation of Water–Soluble Fullerene Derivative by Reaction with Peracetic Acid and its Nanoparticle Size Distribution. Journal of Materials Science and Engineering A2 (1),(2012) 2 (1) 86-97. |
[12] | Zemanova E., Klouda K., Kostakova E.: Fullerene derivatives, preparation, identification and use. Accepted for publication. |
[13] | Tsao N., Kanakamma P.P., Luh T.-Y, Chou Ch.-K., Lei H.-Y.: Inhibition of Escherichia coli-Induced Meningitis by Carboxyfullerence. Antimicrob. Agents Chemother. (1999) 43(9) 2273-7 |