Shahroz Khan 1, Faizul Haq 2, Fariha Hasan 1, Kausar Saeed 3, Rahat Ullah 3
1Department of Microbiology, Quaid-i-Azam University, Islamabad, Pakistan
2Department of Botany, Hazara University, Mansehra, Pakistan
3Department of Zoology, Hazara University, Mansehra, Pakistan
Correspondence to: Faizul Haq , Department of Botany, Hazara University, Mansehra, Pakistan.
Email: |  |
Copyright © 2012 Scientific & Academic Publishing. All Rights Reserved.
Abstract
Although much of the research work has been done on Acidithiobacillus thiooxidans in the rest of the world, unfortunately significant study has not been reported in Pakistan. The principal objective of the present investigations was to isolate and characterized the acidophilic sulphur oxidizing Acidithiobacillus thiooxidans from black shale and to grow the isolates on solid and liquid medium. Acidithiobacillus thiooxidans were Gram-negative, motile, and rod-shaped bacteria. Acidithiobacillus thiooxidans oxidized sulfur and reduced sulfur compounds to sulfuric acid through its metabolic activity. Different biochemical activities like starch hydolysis, gelatin hydrolysis, hydrogen sulphide production, catalyse reaction, urease test, indole production, methyl red test, voges proskaur, citrate utilization and triple sugar iron test of the isolates were performed.
Keywords:
Pakistan, Biochemical Activities, Sulfur, Isolates
1. Introduction
Black shales are dark, as a result of being especially rich in unoxidized carbon, common in some Palaeozoic and Mesozoic strata. Shales may also contain concretions[1]. Bioleaching refers to the mobilization of metal ions from insoluble ores by biological oxidation[2]. The application of bacterial leaching to metal recovery from mineral ores has progressed steadily in the last 20 years[3]. It is assumed that heterotrophic leaching should provides better extraction of metals ions from organometallic compounds in shale ores. Bioleaching is carried out by astonishing diverse groups of bacteria. At least 11 putative prokaryote divisions can be related to this phenomenon[2]. The most common microorganisms belong to the genera Acidithiobacillus and Leptosprillium which are mesophile, acidophilic and chemolithoautotroph. They obtain energy from oxidation of either ferrous ion to ferric or reduction of sulfur compounds to sulphuric acid[4].There are certain important iron and sulfur oxidizing chemolithrophic bacteria and archaea that are involved in the biooxidation of minerals and are responsible for producing ferric iron and sulphuric acid required for the bioleaching reactions[5]. These microbes have a number of features in common. They grow autotrophically by fixing CO2 from theatmosphere, obtain their energy by using either ferrous iron or reduced inorganic sulfur compounds as an electron donor, and generally use oxygen as the electron acceptor, they are acidophilus and grow in low pH environments (pH.1.4 to 1.6 is typical), and are remarkably tolerant to a wide range of metal ions[6].The advantageous characteristic of mineral biooxidation operations is that they are usually not subject to contamination by unwanted microorganisms. In the case of continuous-flow tank leaching processes, the continual wash-out of mineral together with their attached microbes as well as the organisms in suspension provides strong selection for improved microorganisms[5]. Acidithiobacillus ferrooxidans and Acidithiobacillus thiooxidans are used in a mining technique called bioleaching whereby metals are extracted from their ores through oxidation, where these bacteria are used as catalysts[7]. In direct bioleaching the microbes are kept together with the valuable metal-bearing material. In indirect bioleaching the microbes are kept in a pond external to the valuable metal-bearing material and provide the leaching chemicals at a distance. An indirect mechanism might play a critical role in the microbial leaching process[8]. | (1) |
 | (2) |
 | (3) |
The role of the microorganisms is to generate the leaching chemicals and to create the space in which the leaching reactions take place. Microorganisms typically form an exopolysaccharide (EPS) layer when they adhere to the surface of a mineral and EPS serves as the reaction space[2]. The role of the microorganisms in the solubilisation of metal sulphides is, therefore, to provide sulphuric acid for a proton attack and to keep the iron in the oxidized ferric state for an oxidative attack on the mineral[4]. These microorganisms gain energy by breaking down minerals into their constituent elements. The company simply collects the ions out of the solution after the bacteria have finished[3].Despite the advantages with bioleaching it is not always easy to choose among the different methods of metal extraction in order to explore a potential mine. The Techno- Economic factors of a resource need to be evaluated from case to case[4]. This procedure was introduced in studies on leaching of polymetallic shale ore, called Polkowice black shale. Tube bioreactors designed by our research team were used in these bioleaching experiments[9].Bioleaching of heavy metals from contaminated soil was carried out using indigenous sulfur oxidizing bacterium Acidithiobacillus thiooxidans. Experiments were carried out by varying sulfur/soil ratio from 0.03 to 0.33 to evaluate the optimum ratio for efficient bioleaching of heavy metals from soil. The influence of sulfur/soil ratio on the bioleaching efficiency was assessed based on decrease in pH, increase in oxidation–reduction potential, sulphate production and solubilisation of heavy metals from the soil[3]. Decrease in pH, increase in oxidation–reduction potential and sulphate production was found to be better with the increase in sulfur/soil ratio. While the final pH of the system with different sulfur/soil ratio was in the range of 4.1–0.7, oxidation reduction potential varied from 230 to 629 mV; sulphate production was in the range of 2,786– 8,872 mg/l. Solubilisation of chromium, zinc, copper, lead and cadmium from the contaminated soil was in the range of 11–99%[10]. The bioleaching of organometallic ores has to be done with heterotrophic bacteria, which can grow on organic material and degrade compounds such as proteins, fats and carbohydrates. Studies showed that some heterotrophic bacteria in the absence of complex organic compounds can utilize even simple hydrocarbons. Recently the genomic study of different strains of At. thiooxidans were performed by different scientists[11],[12]. The objectives of present study were: To evaluate bioleachability of Polkowice black shale ore, to establish reliable lab scale pilot operations of process, to optimize configuration and settings and to study their biochemical activities and optical density on different media.
2. Materials and Methods
Present study was conducted at Microbiology Research Laboratory, Department of Microbiology, Quaid-i-Azam University Islamabad to isolate and characterized the acidiphilic sulphur and iron oxidizing bacteria from black shale collected from Tarbela, Pakistan.
2.1. Isolation of Microorganisms from Soil and Water Samples
Serial dilution of the samples were done, 10 screw capped tubes were used for serial dilution. About 9 ml of sterilized saline was taken in each tube. One gram of soil (black shale) was added to sterilized saline. After shaking it well, 1 ml of the suspension was transferred to tube 1 aseptically. The tube was shaken and from this tube, 1 ml of the dilution was then transferred to tube 2. Similarly, the 10th dilution was prepared by transferring 1 ml to the next tube, under aseptic conditions. Same process was repeated for all other samples. After serial dilutions 0.1 ml was taken from tube 5 of water sample and 0.1 ml from soil samples and spread on the growth medium plates. The plates were incubated on 300C for 24 hours.
2.2. Microbiological Growth Media
For the growth of bacterial strains, iron Liquid medium (9kFe2+), Sulfur medium (9KS°), Glucose medium were used as liquid media.Thiosulphate solid medium and Glucose medium (Agarose-Glucose medium) was used for isolation and enumeration of At. thiooxidans during present study.
2.3. Isolation and Enumeration of Acidithiobacillus Thiooxidans
One ml aliquot of each liquid sample was inoculated into liquid sulfur medium (9KS°) of pH 2.5 and incubated at 30℃ and 100 rpm. The presence of sulfur-oxidizing bacteria (At. thiooxidans and At. ferrooxidans) in liquid sulfur media was indicated by a drop in pH of the medium due to the production of sulphuric acid. When pH of the medium dropped to less than 1.0, an aliquot of 1.0 g was taken and subcultured into fresh sulfur liquid medium. Finally, after 5-6 subculturing for 5-6, it was centrifuged at 10,000 rpm in a 40℃ for 10 minutes. The pellet was re-suspended in 20 ml of sterilized distilled water, pH 4 adjusted with 1M H2SO4. Tenfold serial dilution of isolated culture was prepared using sterile saline water as diluents. About 0.1ml amounts of each dilution was spread on solid tetrathionate plates and incubated at 30℃. To avoid drying-up the plates were kept in a sealed polyvinyl bag. Gram’s staining was performed as described before. Photographs of the slides were taken and also plates were examined with naked eye to record morphological features of colonies, such as size, shape, and color. Single colonies were picked from the plates by using a steriled loop and inoculated separately into 25 ml vials containing 10 ml tetrathionate liquid media of pH 4.0. All cultures were incubated at 30℃ until the medium became milky and pH dropped to less than 1.0 due to oxidation of tetrathionate by sulfur-oxidizing bacteria. To check the purity of isolated strains, cells growing in liquid tetrathionate medium were spread on solid Gelrite-FeSO4 and glucose plates and observed after 5-7 days of incubation, for the presence of any iron-oxidizing (At. ferooxidans) or glucose-oxidizing (At. acidophilus) bacteria, respectively. They were picked and streaked onto solid tetrathionate, glucose and Gelrite-FeSO4, media to check its growth on glucose and ferrous iron. Single colonies were also subcultured into ferrous iron, tetrathionate, and glucose liquid media. The slides were prepared by Gram’s staining and observed under microscope.
2.4. Biochemical Characteristics
The biochemical characteristics were determined by extra cellular enzymes activities like Starch hydrolysis, Gelatin hydrolysis, while the Intra cellular Enzymes activities were determined by Hydrogen sulphide production, Catalase reaction, Urease test, Indole production test, Methyl red test, Voges Proskauer test, Citrate utilization and Triple sugar- iron test.
2.5. Effect of Carbon Sources on Growth of Bacteria
The carbon sources that were used for the growth of Acidithiobacillus thiooxidans are Glucose, Sucrose, Fructose, Raffinose, D-sorbitol, Galactose, Lactose, Maltose, Rhammanose and Mannose. The optical density of the above carbon sources was taken at 440 nm, after 24, 48, 72, and 96 and 120 hours, to check the growth of isolates.
3. Results
3.1. Isolation and Characterization of Acidithiobacillus thiooxidans
For the isolation of acidophilic sulfur-oxidizing bacteria (At. thiooxidans) from soil and water samples. An appropriate amount (100 µL) of liquid sample was streaked onto solid Agarose-S203 medium. After 12-15 days of incubation at 30oC. Some colonies were off-white, circular in shape and relatively medium in size. This became pale-yellow in color after 3-4 weeks of incubation. A characteristics smell of elemental sulfur (S°) was observed from these colonies. While some were milky white, circular shape and relatively small in size. Each type of single colony was picked and inoculated separately into liquid sulfur (9KSo) and glucose media for further screening. The flasks were incubated on a shaking incubator at 30℃. After 5-7 days of incubation, the sulfur medium became turbid and milky white exhibiting the growth of microbial populations.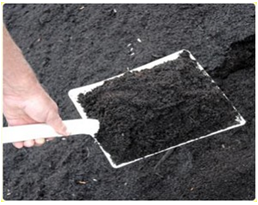 | Figure 1. Black shale in soil form |
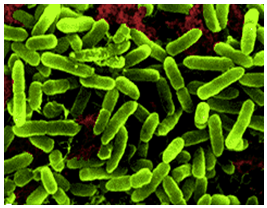 | Figure 2. Electron microscope picture of sulfur oxidizing Acidithiobacillus thiooxidans |
Table 1. Biochemical activities for both Thio+ and Glu+ strains |
| Biochemical activities | Thio+ strain | Glu+ strain | Medium | Result | Medium | Result | Starch hydrolysis | color was blue-black, | Negative | clear zone | Positive | Gelatin hydrolysis | remain solid | Negative | Remain solid | Negative | Hydrogen sulphide | no precipitate with ferrous ammonium sulphate | Negative | insoluble black ferrous sulphide precipitate | Positive | Catalase test | bubbles of free oxygen gas | Positive | bubbles of free oxygen gas | Positive | Urease test | phenol red did not turn to a deep pink | Negative | phenol red did not turn to a deep pink | Negative | Indole production | absence of red coloration | Negative | absence of red coloration | Negative | Methyl red test | Yellow | Negative | Red | Positive | Voges proskauer | deep rose color | Positive | deep rose color | Positive | Citrate utilization | color was not changed | Negative | blue coloration | Positive | Triple sugar iron | color changed | Positive | color was not changed | Negative |
|
|
Table 2. Growth of Acidithiobacillus thiooxidans (Glu+and Thio+) on 1% carbon sources as an energy source at 440 nm optical density after 24 hours interval during five consecutive days |
| Carbon source | Strains | Growth at 440 nm optical density | Maximum growth at | 24 | 48 | 72 | 96 | 120 | Glucose | Glu+ | 0.001316 | 0.004997 | 0.003512 | 0.002841 | 0.004193 | 48h | Thio+ | 0 | 0.0034235 | 0.0072867 | 0.037241 | 0.19134 | After 120h | Sucrose | Glu+ | 0 | 0.001704 | 0 | 0.002196 | 0.23639 | Up to 120h | Thio+ | 0.001713 | 0.14264 | 0.18097 | 0.28433 | 0.26996 | After 72h | Fructose | Glu+ | 0 | 0 | 0 | 0.000254 | 0 | Up to 72h | Thio+ | 0.000 | 0.0008698 | 0.0014500 | 0.00316 | 0.12966 | After 72h | Raffinose | Glu+ | 0.000734 | 0.000375 | 0.10697 | 0.11545 | 0.12358 | After 72h | Thio+ | 0.14922 | 0.00687 | 0.002738 | 0.004638 | 0.008871 | After 120h | D-sorbitol | Glu+ | 0.21647 | 0.02142 | 0.5661 | 0.15344 | 0.12259 | Up to 120h | Thio+ | 0.23211 | 0.26231 | 0.2558 | 0.20217 | 0.1629 | After 48h | Galactose | Glu+ | 0.19135 | 0.17611 | 0.35102 | 0.38479 | 0.64757 | After 24h | Thio+ | 0.007190 | 0.001846 | 0.11681 | 0.009810 | 0.007098 | After 96h | Lactose | Glu+ | 0.0070912 | 0.0024145 | 0.005698 | 0.003151 | 0.008390 | Thio+ | 0.18805 | 0.006727 | 0.005663 | 0.005480 | 0.000 | After 24h | Maltose | Glu+ | 0.0074301 | 0.0063735 | 0.085993 | 0.69364 | 3.33500 | After 120h | Thio+ | 0.18928 | 0.0084773 | 0.18231 | 0.21245 | 0.19117 | After 72h | Rahammanose | Glu+ | 0.22787 | 0.22433 | 0.33156 | 0.57322 | 1.2788 | After 48h | Thio+ | 0.11206 | 0.0074445 | 0.0094829 | 0.009584 | 0.18174 | Different | Mannose | Glu+ | 0.12652 | 0.11039 | 0.74809 | 0.14488 | 1.55176 | After 48h | Thio+ | 0.13461 | 0.0086341 | 0.14731 | 0.30430 | 0.15634 |
|
|
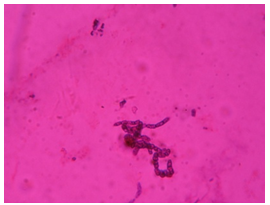 | Figure 3. Glu+ bacteria after gram’s staining |
3.2. Cell Morphology and Characterization of Isolated Strains
The compound microscopic observations of isolated strains of At. thiooxidans revealed that these strains were Gram-negative, motile, and single rod-shaped bacteria. Acidithiobacillus thiooxidans oxidized only sulfur and reduced sulfur compounds to sulphuric acid through its metabolic activity.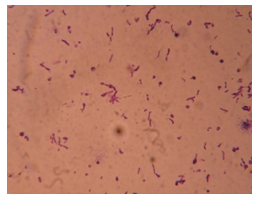 | Figure 4. Thio+ bacteria after gram’s staining |
3.3. Growth Studies of Acidithiobacillus thiooxidans
After the gram’s staining different biochemical activities were analysed for the both Glu+ and Thio+. These activities were represented in table 1. The effect of carbon source on the growth and optical density of both Thio+ and Glu+ were presented in table 2.
4. Discussion
Indigenous Acidithiobacillus were isolated from black shale and water samples. In the tailing of soil and water samples microbial populations of At. ferrooxidans, At. thiooxidans and glucose-oxidizing heterotrophs were detected. Different solid media have been used for the isolation and enumeration of Acidithiobacillus including a new efficient Gelrite-FeSO4 solid medium[3].Dark reddish-brown and circular colonies were developed on Gelrite-FeSO4 medium within 72-96 hours. The cells of At. thiooxidans were motile, rod-shaped. The colonies developed on thiosulphate medium, with a characteristic smell of elemental sulfur.Both the strains of At. ferrooxidans and At. thiooxidans were found in abundance in tailings of water and liquid samples. Elemental sulphur can be utilized as an energy source by iron and sulphur oxidizing bacteria to produce H2SO4 resulting in a pH drop of the tailings residue[13]. Sulphuric acid thus produced can change the physical and chemical characteristics of tailing residues[14]. The presence of glucose-oxidizing heterotrophs was also noted in black shale liquid, microbial leach liquors and solid samples obtained from columns and heap. Microbial leaching is a biochemical process involving enzymes as catalyst by which insoluble inorganic substrate is oxidized to a soluble form[3]. Metals are released from sulphide minerals directly through oxidative metabolism of microorganisms or solubilised indirectly by chemical oxidants such as ferric sulphate or sulphuric acid produced as metabolic products of microorganisms[11]. Incomplete oxidation of the sulphide entity commonly occurs in the acid leaching process which results in the formation of polythionates and the precipitation of elemental sulphur[12]. The latter effectively coats the metal sulphides and prevents their further oxidation until the sulfur is removed by bacterial oxidation. The microbial degradation of silicate minerals requires the availability of external energy substrates. Different biochemical activities of the isolates were performed. Starch hydrolysis test was performed in the case of Thio+ strain, where as Glu+ hydrolysed the starch and indicated a positive result. This showed that Glu+ was better hydrolyser of starch than Thio+. Both the isolates were not able to hydrolysed the gelatin. Glu+ showed the production of hydrogen sulphide, by forming an insoluble black ferrous sulphide while in case of Thio+, the absence of precipitate was the sign of negative result. Both isolates were catalase positive by producing bubbles of free oxygen gas. Both the isolates were urease negative. Isolates did not produce a red reagent in both the cases of Thio+ and Glu+. The absence of red coloration demonstrated that the substrate tryptophan was not hydrolysed and indicated an indole negative reaction. In methyl red test, the Glu+ strain by the presence of acid indicated positive result. While the Thio+ result was negative. In both Thio+ and Glu+ a deep rose color developed which indicated the presence of acetyl-methylcarbinol and represented a positive result for voges proskauer test. Citrate-positive Glu+ was indicated by the presence of growth on the surface of the slant. Which was accompanied by blue coloration and showed that Glu+ has used citrate as a carbon source. While in case of Thio+ slant color, was not changed, which showed that the result was negative. In case of Thio+, the color change showed the carbohydrate fermentation activity and the result was positive while in case of Glu+ the color was not changed showing that the carbohydrate fermentation has not taken place and the result was negative in triple sugar iron. The growth pattern of Glu+ in the presence of 1% glucose in 9k medium indicated that glucose was utilized as carbon source and growth of the isolate was maximum at 48 hours of incubation. Where as the strain Thio+, on 1% glucose (9k medium) started the growth after 72 hours of incubation and after the 96 hours the growth was at peak. For Thio+ the glucose was found to be good energy source as compared to that in case of Glu+ strain. The result indicated that the Glu+ has started growth in the presence of 1% sucrose (9k medium) after 24 hours of incubation. The growth of Thio+ on the same medium, after the incubation of 48 hours showed that for both the sucrose was good for growth[15].In the presence of 1% fructose (9k medium) the Glu+ was grow after 72 hours incubation. Where as Thio+ strain on 1% fructose (9k medium) after the 96 hours of incubation, the growth was at peak. For Thio+ the Fructose was found to be good energy source as compared to that in case of Glu+ strain. The strain Glu+ growth in the presence of 1% raffinose (mineral salt medium) after 48 hours of incubation was at peak. Where as Thio+ has started growth after the incubation of 120 hours on same above medium. On comparison the growth of Thio+ was found to be better on raffinose than in case of Glu+ strain.The growth of Glu+ strain in the presence of 1% D-sorbitol (9k medium) was maximum after 144 hours of incubation. Where as in the case of strain Thio+ on the same medium the growth was at peak 96 hours of incubation. It was concluded that Glu+ utilized D-sorbitol as a good energy source as compared to strain Thio+ .The results indicated that on (mineral salt medium) galactose 1% the growth of strain Glu+ after the incubation of 216 hours was maximum. While in case of strain Thio+ the growth on same medium shoot up on 96 hours of incubation and immediately dropped. This indicated that the Glu+ strain utilized best galactose as a carbon source as compared to that in case of Thio+ strain. In the presence of lactose 1% (mineral salt medium) the strain Glu+ started growth after the incubation of 120 hours and was maximum after 192 hours. Where as on the same medium strain Thio+ growth was maximum after 24 hours incubation. The conclusion was that Thio+ strain was found to be better utilizer of Lactose as a carbon source than strain Glu+.In the presence of 1% maltose in 9k medium the strain Glu+ indicated clear results, that maltose was utilized as carbon source and growth of the isolate was maximum at 144 hours of incubation. Where as the strain Thio+ on the same medium indicated maximum growth after 120 hours of incubation. From this it was concluded that Glu+ strain was found to be best utilizer of maltose as an energy source as compared to that in case of Thio+ strain.Result indicated that the strain Glu+ was grown in the presence of 1% rhammanose (9k medium) after the incubation of 48 hours. Where as the result of Thio+ was different in which after the incubation of 144 hours, the growth was at peak. This indicated that on rhammanose the Glu+ strain growth was found to be better than Thio+ strain. The growth pattern of Glu+ in the presence of 1% mannose (mineral salt medium) indicated that the growth after the incubation of 216 hours was maximum. Where as the Thio+ strain on the same medium showed growth after 96 hours of incubation. The conclusion was that strain Glu+ was found to be best utilizer of mannose as compared to that in case of Thio+ strain.
References
[1] | Tomasz Grobelski, Jadwiga Farbiszewska-Kiczma, Teresa Farbiszewska.. Bioleaching of Polish Black Shale. Physicochemical Problems of Mineral Processing, 41 (2007), 259-264 |
[2] | T. Rohwerder, T. Gehrke, K. Kinzler, W. Sand. Progress in bioleaching: fundamentals and mechanisms of bacterial metal sulphide oxidation. Appl Microbiol Biotechnology. 2003. 63: 239-248. |
[3] | Shahroz Khan, Faizul Haq, Fariha Hasan, Kausar Saeed, Rahatullah. Isolation and Characterization of Acidophilic Sulphur and Iron Oxidizing Acidithiobacillus ferrooxidans from Black Shale. International Journal of Biosciences. Vol. 2, No. 2, p. 85-94, 2012 |
[4] | D. E. Rawlings, D. Dew, C. Plessis. Biomineralization of metal containing ores and concentrates. Trends in Biotechnology. 2003. 21:38–44. |
[5] | D. E. Rawlings, B. D. Johnson. The microbiology of biominig development and optimization of mineral oxiding-microbial consortia. Microbiology. 2007 Feb;153 (Pt 2):315-24. |
[6] | Mark Dopson, Craig Baker-Austin, P. Ram Koppineedi and Philip L. Bond. Growth in sulfidic mineral environments, metal resistance mechanisms in acidophilic micro-organisms. Microbiolo; 2003. 149: 1959-1970. |
[7] | D.P. Kelly, A.P. Wood. Reclassification of some species of Thiobacillus to the newly designated genera Acidithiobacillus gen. Nov., Halothiobacillus gen. nov. and Thermithiobacillus gen. nov., Internacional Journal of Systematic and Evolutionary Microbiology. 2000. 50: 511-516. |
[8] | Jadwiga Farbiszewska-Kiczma, Teresa Farbiszewska , M. Bąk. Bioleaching of metals from polish black shale in neutral medium, Physicochemical Problems of Mineral Processing, 2004, 38, p. 273-280. |
[9] | Jadwiga Farbiszewska-Kiczma, Teresa Farbiszewska. Isolation of bacteria that degrade organometallic compounds from metallic wastes, Physicochemical Problems of Mineral Processing. 2005. 39, 263-267. |
[10] | R. Nareshkumar, Nagendran Parvathi. Bioleaching of heavy metals from contaminated soil using Acidithiobacillus thiooxidans: effect of sulfur/soil ratio. World J Microbiol Biotechnology. 2007. 24: 1539–1546. |
[11] | Jorge Valdes, Francisco Ossandon, Raquel Quatrini, Mark Dopson, and David S. Holmes Draft Genome Sequence of the Extremely Acidophilic Biomining Bacterium Acidithiobacillus thiooxidans ATCC 19377 Provides Insights into the Evolution of the Acidithiobacillus Genus. Journal of Bacteriology. 2011, p. 7003–7004 |
[12] | Quatrini, R., et al. 2009. Extending the models for iron and sulfur oxidation in the extreme acidophile Acidithiobacillus ferrooxidans. BMC Genomics 10:394. |
[13] | J.T. Pronk, W.M. Meijer, W. Haseu, J.P. Dijken, P. Bos, J.G. Kuenen. Growth of Thiobacillus ferrooxidans on formic acid. Appl Environ Microbiol; 1991. 57: 2057-2062. |
[14] | Gonzalo A, Padilla Luis, Cisternas A, and Cueto Y. (2008). On the optimization of heap leaching. Minerals Engin; 21: 673–678. |
[15] | Song C, Zhou Q, Qing Q, and Yue L. (2008). Bioleaching of sphalerite by Acidithiobacillus ferrooxidans and Acidithiobacillus thiooxidans cultured in 9K medium modified with pyrrhotite. J. Cent. South Univ. Technol; 15: 503-507. |