Armin Berenjian, Gareth Whittleston
School of Engineering, University of Salford, Manchester, United Kingdom
Correspondence to: Armin Berenjian, School of Engineering, University of Salford, Manchester, United Kingdom.
Email: |  |
Copyright © 2017 Scientific & Academic Publishing. All Rights Reserved.
This work is licensed under the Creative Commons Attribution International License (CC BY).
http://creativecommons.org/licenses/by/4.0/

Abstract
Glass usage in structures has increased dramatically during the last decades due to its special properties, such as transparency, high theoretical strength, low density, durability and functionality. This paper reviews glass as a material in addition to different methods of glass manufacturing (float glass and cast glass) and how they have developed over the years. In addition, this paper reviews different glass types (annealed glass, heat strengthened glass, toughened glass, and laminated glass) and their properties.
Keywords:
Glass, Glass manufacturing, Glass as a material
Cite this paper: Armin Berenjian, Gareth Whittleston, History and Manufacturing of Glass, American Journal of Materials Science, Vol. 7 No. 1, 2017, pp. 18-24. doi: 10.5923/j.materials.20170701.03.
1. Introduction
The word glass comes from the Teutonic term “Glaza”, which means amber. Although the origin of glass manufacture is still uncertain, the Mesopotamians from the 5th century BC discovered an ash by chance when they fire to melt clay vessel to use for glazing ceramics or when copper was smelted. In Egypt, greenish glass beads were excavated in some of the Pharaohs’’ burial chambers dating from the early 4th century BC, and this has been referred to as intentional glass manufacture. From the second century BC, the production of rings and small figures by using core-wound techniques began to appear. The oldest blueprint for glass was made on clay tablets in 669-627 BC, which read: “Take 60 parts sand, 180 parts ash from marine plants, and 5 parts chalk”. This blueprint is now held in the great library of the Assyrian king, Ashurbanipal, in Nineveh. [1]The invention of the Syrian blowing iron around 200 BC by Syrian craftsmen enabled the production of thin-walled hollow vessels in a wide variety of shapes. Excavations have revealed that in the Roman era glass was used for the first time as part of the building envelope of public baths in Herculaneum and Pompeii. These panes could have been installed in a bronze or wood surround or without a frame. In the middle ages, this technique spread to the northern Alpine regions, and utensils like drinking horns, claw beakers, and mastos vessels started to be produced; in addition, the use of glass increased in the building of churches and monasteries. [2]Blown cylinder sheet glass and crown glass were invented in the 1st century AD and the 4th century AD respectively. In both, a blob of molten glass was drawn off with a blowing iron, performed into a round shape, and then blown into a balloon. Blown cylinder sheet glass and crown glass remained two of the most important production techniques for producing flat glass until the early 20th century. From the 17th century, glass usage was not only limited to churches and monasteries but it also started to be used for glazing palaces. High demand motivated glass-makers to develop new methods, and in 1687 the process of casting glass was invented by the Frenchman Bernard Perrot, in which the glass melt was poured onto a smooth preheated copper table and pressed onto a pane with a water-cooled metal roller. In this way, a glass pane of up to 1.20 x 2 m could be produced. Although this method made it possible to produce glass at a cheaper price, the use of glass windows was still expensive.Considerable improvement was made after industrialisation in the 19th century. In 1839, the Chance brothers succeeded in adapting the gridding, cutting and polishing of blown cylinder glass in order to reduce breakages and improve the surface finish. In the 1850’s, it became possible to produce a massive amount of glass panes required for the construction of a crystal palace. Machine-made glass panes were not produced until 1905, when Emile Fourcault succeeded in drawing these directly out of glass melt. In 1919, Max Bicheroux made a vital discovery in the production of glass by concentrating several stages of the procedure into a continuous rolling mill; the glass melt left the crucible in portions and passed through two cooled roller to form a glass ribbon. In this way, a glass pane with the dimensions of 3 x 6m could be produced. In the 1950s, the Englishman Alastair Pilkington developed the float glass method, wherein viscous glass melt was passed over a bath of molten tin floating on the surface. Tin was used because of the high temperature range of its liquid physical state (232 to 2270°C) and having a much higher density then glass. [2]
2. Current Glass Production
Figure (1) details some of the most common glass production processes, processing methods and products. As shown, the main production steps are almost identical, with a melting point of between 1600 and 1800°C, a formation of between 800 and 1600°C, and cooling between 100 and 800°C.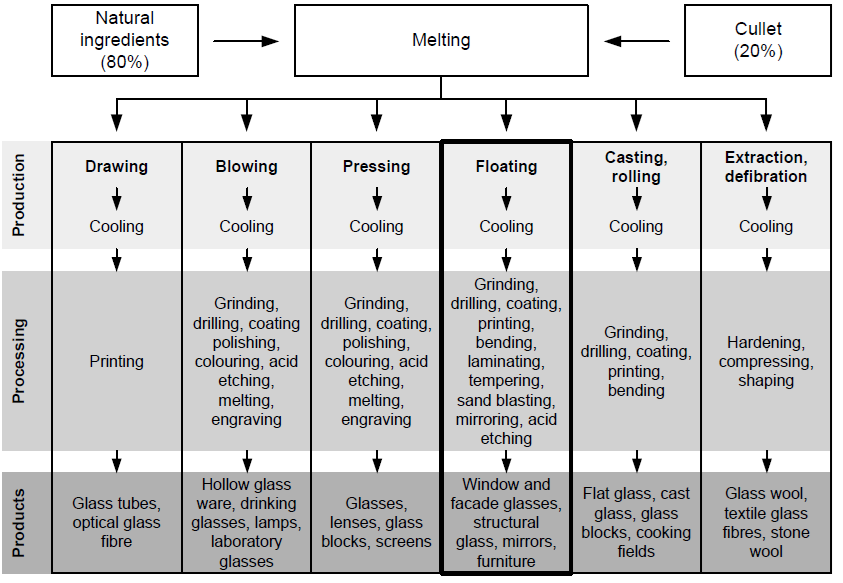 | Figure 1. Glass Production processes and products overview [3] |
Floating is currently the most popular process, representing over 90% of all flat glass production worldwide. Float glass is made in large manufacturing plants which operate 24 hours a day, 365 days a year. In this process, raw materials are melted at 1550°C, and the molten glass is poured continuously at 1000°C onto a shallow pool of tin. The glass float on the tin forms a smooth flat surface of almost equal thickness (depending on the speed of the rollers), which then starts to cool to 600°C; after this, it enters the annealing Lehr oven and slowly cools down to 100°C to prevent any residual stress. The typical size of glass panes are 6 x 3.20 m, and hard coating can be applied during the manufacture. [3]In this process, the two sides of the glass pane are slightly different. On the tin side, some diffusion of tin atoms onto the glass surface occurs, [5] causing a lower glass strength on this side due to the surface flaws occurring during production. [6] The tin side can be easily detected by ultraviolet radiation.Another process for the production of flat glass is the cast process. In this process, molten glass is poured continuously between metal rollers to produce glass with the required thickness. The rollers can be engraved to give the required surface design or texture and produce patterned glass. The glass can be given two smooth surfaces, one smooth and one textured, or two textured sides, depending on the design. In addition, a steel wired mesh can be sandwiched between two separate ribbons of glass to produce wired glass. Wired glass can keep most of glass pieces together after breakage, and it is therefore usually used as fire protection glass.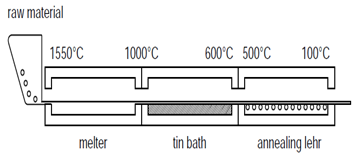 | Figure 2. Float glass production process [4] |
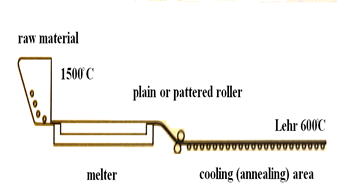 | Figure 3. Cast glass production |
This type of glass is not transparent, but rather translucent, so it cannot equal the transparency of float or drawn sheet glass. The physical properties of patterned and float glass are identical. The production of glass profiles is currently limited to U-shaped profiles and tubes (circular hollow sections).
3. Material Properties
Glass is an inorganic product of fusion, which has been cooled to a rigid condition without crystallization. Soda Lime Silica Glass (SLSG) is the most common type of glass in construction. Borosilicate Glass (BSG) is used for special applications in which extra resistance to temperature changes is needed, such as in heat resistant glazing or fire protection glazing. Glass does not consist of geometrically regular networks of crystals; instead, it is an irregular network of oxygen and silicon atoms with alkaline atoms (like Calcium or Sodium), as shown in Fig 4.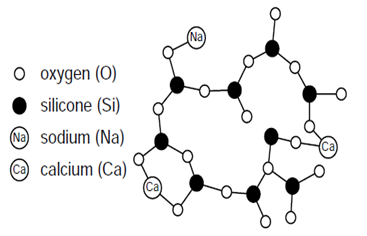 | Figure 4. Soda lime silica glass's irregular network [4] |
Table 1 shows the chemical composition of soda lime silica glass and borosilicate glass according to European standards. The chemical composition has an important influence on viscosity, thermal expansion and the melting temperature of glass. For example, by adding alkali materials to pure silica oxide, the melting temperature drops from 1700°C to 1300-1600°C.Table 1. Chemical compositions of two common types of glasses 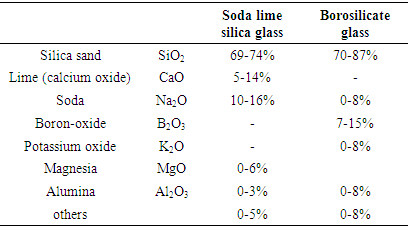 |
| |
|
Viscosity constantly increases during the cooling of liquid glass, until solidification occurs at about 1014 Pas. The temperature at solidification, called the glass transition temperature, is about 530°C for SLSG. The glass actually freezes, and no crystallization takes place. The extremely cooled liquid nature of glass means that, unlike most solids, the electrons cannot absorb energy to move to another energy level and are strictly confined to a particular energy level. Therefore, the molecules will not absorb enough energy to dissipate energy in ultra violet, infrared or visible bandwidths. However due to some impurities in SLSG, the glass could be greenish or brownish due to Fe2+ and Fe3+ respectively.Extra clear glass, called low iron glass, which has a reduced amount of iron oxides, is commercially available. The physical properties of glass mainly depend on the glass type. At room temperature, the dynamic viscosity of glass is about 1020 dPas, a very high amount bearing in mind that water is 1 dPas and honey is 105 dPas. With this high viscosity at room temperature, it could take more than an earth age for flow effects to be visible to the naked eye. Although some observation have shown that in old churches glass panes are thicker at the bottom than at the top, and have referred to this as flow, it is actually because of the glass manufacturing process at the time which was reliant on centripetal force relaxing (crown glass process), making the centre much thinner than the outer parts; in addition, when being installed, the thinner part was usually placed at the top for better visual sparkle and stability. [7]Some of important physical properties of glass are shown in table.Table 2. Physical Properties of Glass 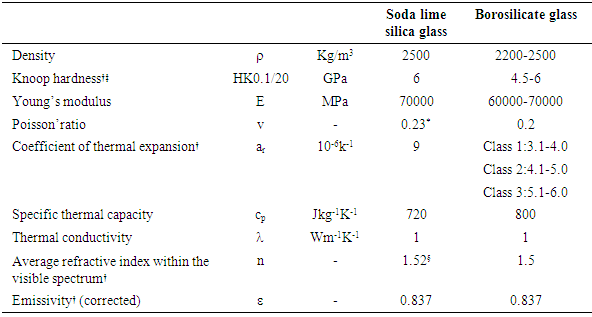 |
| |
|
4. Glass Types
Annealed glass is currently the most widely used type of glass. By using industrial processes (such as the float glass procedure), it is possible to produce massive quantities (600 tons of 4mm glass each day) of high quality clear glass with an (almost) flat surface. The thickness of glass could vary from 2mm to 25mm. By changing the raw materials (for example, lower Fe2O3 or FeO), it is possible to eliminate the natural green or brown tint of the glass, and this type of glass is called low iron glass or clear white glass.Annealed glass breaks into large dagger like shards with sharp edges, which could be a possible hazard if they fall.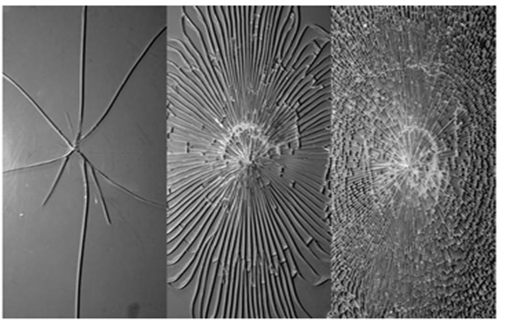 | Figure 5. Fractures of different type of glasses, from left: 1. Annealed glass, 2. HSG, 3. Thermally toughened glass |
Toughened glass is a kind of safety glass, which has a higher strength due to its residual stresses. It cannot be worked on any further (such as cutting or drilling) after the toughening process has been done [7]. Toughened glass is becoming more and more important as its range of applications grow. The main application of thermally toughened glass is glass for construction, automotive glass and some domestic glasses like Pyrex, while the main uses of chemically strengthened glass are as laboratory and aeronautical glass.Toughened glass (also known as Fully Tempered Glass - FTG) begins with annealed glass. It is heated to 620°C - 675°C (90-140°C above the transition temperature) and rapidly cooled with jets of cold air. This causes the outer surface of the glass to solidify before the inner part. As the interior cools, it tries to shrink, but the solidified outer surface resists this force and goes into compression (usually between 90 and 150 N/mm2) and the interior goes into tension. The temperature distribution is usually parabolic, with a colder surface and a hotter interior. To get the best results with maximum temper stress, the surface should be solidified exactly at the point when the highest temperature difference occurs and the initial tensile stress is released. In this type of glass, surface flaws do not propagate under compressive stress, and so toughened glass can sustain higher stresses than annealed glass. [9] Glass with low thermal expansion, such as BSG, is more difficult to be toughened. [10]EN 12150 parts 1 and 2, the fragmentation count and the maximum fragment size are specified as standard requirements, although American standards (ASTM C 1048-04) take 10000psi (~69 MPa) surface compression or 9710 psi (~67Mpa) as the minimum standard requirements. Different manufacturing methods can produce glass with widely different properties, and this could be due to the jet geometry, thermal expansion coefficient of the glass, air temperature, roller influence, glass thickness, air pressure, heat transfer coefficient between air and glass, etc. Toughening can have a great effect on the stress to the surface and interior of glass. Chemical toughening (tempering) is an alternative process to thermal toughening. Cutting and drilling is possible, but the cut or drilled parts will have the strength of annealed glass. The use of chemical tempering is very uncommon; it is used in conditions where the extreme angle or geometry causes thermal tempering to be not as effective as it should be. [12] The toughening process is based on ionic exchange (sodium ions in glass exchange with potassium); to do this, the glass is immersed in hot molten salt, which leads to compressive stress at the surface. However, the strengthened zone is shallow to about 20µm in 24 hrs [13]. The shortcoming of this type of glass is that if surface flaws are deeper than the compression zone, sub-critical crack growth can occur without an external load. This phenomenon, which can cause spontaneous failure, is called self-fatigue. [14] The fracture behaviour of this type of glass is like float glass.Heat strength glass (HSG) is produced using the same process for fully tempered glass, but with a lower cooling rate. In this way, the compressive stress that builds up at the surface is lower than that for safety glass, so its strength lies somewhere between annealed glass and thermally toughened glass. Heat strength glass is not classified as safety glass when used in a single pane, but it could be used as laminated safety glass (bearing in mind that the fracture pattern could be changed depending on its interlayer). It is therefore better to frame HSG in four edges due to its fracture pattern. Heat strength glass cannot be worked on any further, and only edge to edge fractures occur in this type of glass. Large fracture patterns result in a good post breakage performance by being able to resisting some loads. [15]Laminated glass consists of at least two panes bonded together by some transparent plastic (or polymer) interlayer. The glass pane may differ in thickness or even in heat treatment. Autoclaving is the most common lamination process; it is done at about 140°C and 14 bar pressure to ensure that the layers are stuck together without any void or air inclusion between them. [3]Laminated glass is of major interest in structural applications. Laminating the glass will result in significant improvements in its post breakage behaviour, and it is therefore a safety glass in that its fragments stick together after fracture. [7] After breakage, the glass fragments adhere to the film so that a certain remaining structural capacity is obtained as the glass fragments lock in place. This capacity increases with the increase in fragment size. [3]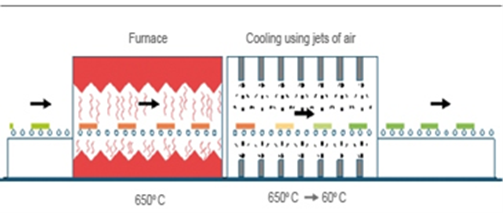 | Figure 6. Toughened process |
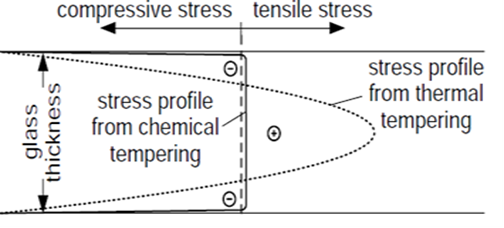 | Figure 7. Residual stresses in toughened glasses [14] |
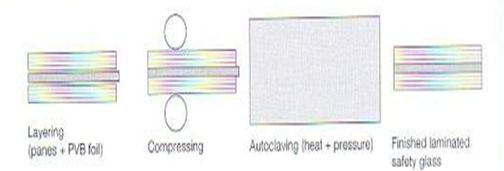 | Figure 8. Laminated glass production [15] |
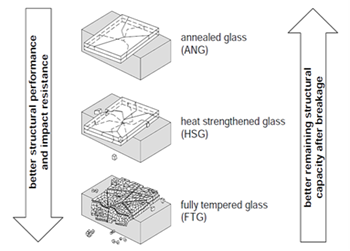 | Figure 9. Post breakage of Laminated Safety Glass with different types of glasses |
PVB (Polyvinyl Butyral) is the most common interlayer because it almost completely blocks UV radiation. A single PVB foil has (roughly) 0.38 mm thickness; in industry, usually 2 or 4 foils of PVB are used to form one PVB interlayer. PVB is a viscoelastic material, meaning that its physical properties vary with temperature and time duration. It is very soft, with 200% elongation at breakage at room temperature. At temperatures below freezing point (0°C) and for short loading times, PVB can usually transfer the full shear from one pane of glass to another. However, by increasing the temperature and long loading, the shear transfer reduces [16]. To produce a laminated PVB glass unit, the plastic sheet is placed between the panes and the whole unit is pressed together in an autoclave under the action of heat and pressure (Fig 10). As the plastic sheet is initially very dry, it can absorb humidity and hence weaken the bond at the edge, so extra care should be taken when installing to prevent any humidity. [2]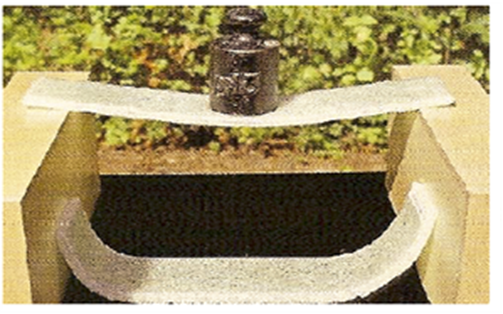 | Figure 10. DuPont’s Sentry Glass vs. PVB, right: Fire protection glass |
There are alternative interlayers with a higher stiffness, tensile strength, or temperature resistance - DuPont’s Sentry Glass Plus being an example - but they are not widely used due to the lamination process not being efficient enough up until now. [17] Special properties like bullet resistance, blast resistance, sound insulation, and fire protection glass can be achieved through different laminating processes. For instance, when fire protection glass is exposed to fire, the pane facing the flames fractures but remains in place, and the interlayers foam up to an opaque insulating shield that blocks any heat passing through. [14]An Insulating Glass Unit (IGU) consists of at least two separate panes kept apart by spacers fitted around the edge. The first seal is located between the spacers, and the panes of glass prevent moisture from entering the cavity between the panes. The second seal is located behind the spacers and between the panes, and this seal helps keep the panes and spacers together. Although the sealing prevents outside air (with probable moisture) from entering, but also if air could enter inside the panel due to damage to sealing, humidity will not rise in the cavity until the desiccants is saturated. When the air cools to below the dew point, the moisture in the air condenses and makes the pane fogged. [3]The most important function of insulating glass units is to reduce thermal loss; modern IGUs have achieved a heat transfer coefficient of 1.1 W/m2k for double and 0.7 W/m2k for triple glazed units. All types of glass can be used in IGUs.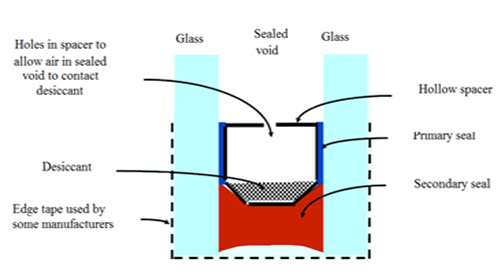 | Figure 11. Insulating glass [18] |
References
[1] | Rice, P. and Dutton, H. (1995). Structural Glass. 2nd Edition. UK: Taylor & Francis. |
[2] | Schttich, C., Staib, G., Balkov, D., Schuler, M. and Sobek, W. (2007). Glass Construction Manual. 2nd Edition. Munich: Institut fur internationale architektur-dokumentation GmbH. pp.8-10 & pp 15-20. |
[3] | Overend, M., Haldimann, M. and Luible, A. (2008). Structural Use of Glass. 1st Edition. Zurich: ETH Zurich. pp.1-4. |
[4] | Overend, M., Bridge, I,., S., Luible, A., and Haldimann, M. (2008). Structural Use of Glass. Zürich, Switzerland: International Association for Bridge and Structural Engineering. |
[5] | Haldimann, M. (2009). Fracture Strength of Structural Glass Elements. 1st Edition .Germany: Hochschulschriften. pp.6-7. |
[6] | Lotz, S. (1995). Untersuchung zur Festiket und Langzeitbestandigkeit adhasiver verbindungen zwischen fugepartnern aus floatglas. 1st Edition .Germany: University of Kaiserslautern. pp.1-4. |
[7] | Sadlacek, G., Blank, K., Laufs, W. and Gusgen, J. (1999). glas im konstruktiven ingenieurbau. Earnst & Sohn. |
[8] | Schttich, C., Staib, G., Balkov, D., Schuler, M. and Sobek, W. (2007). Glass Construction Manual. 2nd Edition. Munich: Institut fur internationale architektur-dokumentation GmbH. pp.30. |
[9] | Overend, M., Haldimann, M. and Luible, A. (2008). Structural Use of Glass. 1st Edition. Zurich: ETH Zurich. p.15. |
[10] | O’Regan (The Institution of Structural Engineers), C. (1999). Structural use of glass in buildings. first edition edn. London: IStructE Ltd. |
[11] | Overend, M., Haldimann, M. and Luible, A. (2008). Structural Use of Glass. 1st Edition .Zurich: ETH Zurich. p.11. |
[12] | ACG Glass. (no date) Available at: http://us.agc.com/sites/default/files/TemperingProcess.jpg (Accessed: 29 January 2017). |
[13] | Overend, M., Haldimann, M. and Luible, A. (2008). Structural Use of Glass. 1st Edition. Zurich: ETH Zurich. p.12. |
[14] | Worner, J.D, Shneider, j. and Fink, A. Glasbau: Grundlagen, berechnung, konstruktion. |
[15] | Haldimann, M. (2009). Fracture Strength of Structural Glass Elements. 1st Edition .Germany: Hochschulschriften. pp.6-7. |
[16] | Schttich, C., Staib, G., Balkov, D., Schuler, M. and Sobek, W. (2007). Glass Construction Manual. 2nd Edition. Munich: Institut fur internationale architektur-dokumentation GmbH. pp.68. |
[17] | Overend, M., Bridge, I., S., Luible, A., and Haldimann, M. (2008). Structural Use of Glass. Zürich, Switzerland: International Association for Bridge and Structural Engineering p15. |
[18] | DuPont (2003). SentryGlas Plus-The interlayer for Structural Laminated Glass. DuPont, 2003. |
[19] | Yang, J. (2012). Advanced Structures. Birmingham: University of Birmingham. |