Efe Ikponmwosa1, Funso Falade1, Christopher Fapohunda2, James Aransiola1
1Department of Civil and Environmental Engineering, University of Lagos, Nigeria
2Department of Building, Caleb University, Imota, Lagos, Nigeria
Correspondence to: Efe Ikponmwosa, Department of Civil and Environmental Engineering, University of Lagos, Nigeria.
Email: |  |
Copyright © 2014 Scientific & Academic Publishing. All Rights Reserved.
Abstract
This study reports the results of investigation conducted to evaluate the performance of foamed aerated concrete beams with bamboo as the tensile reinforcement. The flexural parameters assessed were, load-deflection behaviour, failure mode, and flexural moments. Its density and compressive strength were also investigated. Foamed aerated concrete made from cement-sand ratio of 1:3 with foaming agent to water ratio of 1:33 was prepared. Twelve 150x150x150mm cube specimens were tested for compressive strength at different curing ages of 7, 21, 28 and 45days. Three sets of 12 beams of 225 x 225 x 2350 mm containing bamboo as reinforcement were tested for flexural strength. The first, second and third set respectively had 2, 4, and 6 numbers of 10 mm x 10 mm bamboo as reinforcement. For the cube specimens tested, the compressive strength increased with curing age. The flexural moments of beams increased with increase in the percentage of bamboo strip reinforcement and curing age. All the beams exhibit flexurfal failure. The deflection decreased with increase in the area of bamboo splints as tensile reinforcement, but failure load decreased with increase in area of bamboo in the tension zone. It can be concluded that inclusion of bamboo splints as reinforcement in tension zone improved the flexural performance of foamed aerated concrete.
Keywords:
Aerated concrete, Bamboo, Beams, Flexural strength, Ultimate moment
Cite this paper: Efe Ikponmwosa, Funso Falade, Christopher Fapohunda, James Aransiola, Flexural Performance of Bamboo-Reinforced Foamed Aerated Concrete Beams, American Journal of Materials Science, Vol. 4 No. 2, 2014, pp. 56-63. doi: 10.5923/j.materials.20140402.02.
1. Introduction
Bamboo as reinforcing material to concrete is a recent innovation in structural engineering, though building with bamboo dates back to an ancient time and tradition in the regions in which the plant grows in abundance, such as in South America, Africa and, in particular, South-East Asia. Bamboo attains its greatest strength after three years, when it assumes a brownish colour. The bending strength of bamboo depends significantly on the location of nodes, which stiffens stem at intervals sufficient enough to preventing it from buckling or collapse. The tensile strength of bamboo is relatively high. Yu et al. (2008) reported that values of tensile strength for bamboo vary from between 115 and 309 N/mm2. Agarwal and Maity (2011) observed a tensile strength of 370N/mm2 in their own investigations. Thus it is obvious that bamboo’s tensile strength can be in the range of mild steel with tensile strength in excess of 250N/mm2. This suggests that bamboo can be used to replace mild steel for low cost structural construction. Research efforts have also been directed towards the development of methodology for bamboo application in space structures and as reinforcement in concrete (Ghavami, 2004). Investigation conducted by Sakaray et al. (2012) revealed that bamboo can be used as substitute for steel reinforcement. AdomAsamoah and Afrifa (2011) in their studies did a comparative study of bamboo-reinforced beams with different stirrup material, with a view to determining the most economic for low cost construction. The stirrup materials used in the study were cane, bamboo, and steel. Through the beam performance index in terms of energy absorbed per unit cost of beam, they found out steel stirrups to be the most economical. Falade and Ikponmwosa (2006) investigated the scope of bamboo reinforcement in concrete beams for low-cost housing. Findings showed that the computed deflection for each loading of bamboo-reinforced beams increased with increase in value of applied moment and span but reduced with increase in the quantity of bamboo reinforcement in the beams. The results also show that the optimum span for bamboo reinforcement in reinforced concrete beams based on cross-sectional dimensions of 225x450mm is 4000mm at optimum load of 60% Mu (ultimate moment of resistance). Within this limit, the requirements of ultimate and serviceability limit states were achieved. Presently, most research efforts are towards the use of bamboo as reinforcement in conventional concrete. Research efforts to widen its used in foamed aerated concrete are limited. Foamed aerated concrete is a lightweight concrete that is increasingly becoming popular as a weight-reducing material and for low cost construction. Its use has also spread to the area of geo-technics and foundation engineering where it is being used as void filling and ground improvement material (Falade and Ikponmwosa, 2008). However, the need to enhance the use of foamed aerated concrete as structural material for low cost construction requires that possible substitute to steel (which is expensive) as reinforcing material be found through research efforts.The objective of this work is to investigate to what extent the use of bamboo as tensile reinforcement can improve the structural performance of foamed aerated concrete. The parameters investigated were: density, compressive strength, and flexural performance measured in terms of crack pattern and deflection. The definitions of some nomenclature in the paper are:fbT = ultimate stress of bamboo in tension = 95N/mm2fbC = ultimate stress of bamboo in compression = 20N/mm2s = depth of compression zone in the aerated concrete section of the simplified stress blockfcua = compressive stress of aerated concrete TAb = area of tensile bamboo reinforcementAb’ = area of compressive bamboo reinforcementB = with of the beam sectiond = depth of tensile reinforcementd’ = depth of bamboo compressive reinforcementMu = ultimate moment of resistance
2. Experiment Details
2.1. Materials
The cement used was Ordinary Portland Cement (OPC) which conforms with British Standard B.S 12 of 1971. The fine aggregate was River sand from Sango Otta of Ogun State Nigeria, having grains with a lower size limit of 70 or 60 µm and not larger than 4mm, with quartz being the major mineral constituent, the particle size distribution curves of the sand was determined. The coefficient of uniformity (Cu) and the coefficient of curvature (Cc) used to standardize gradation criteria for the sand were obtained from the relationships
and
, where C60 = diameter in millimeters of the 60% passing size, D30 = diameter in millimeters of the 30% passing size and D10 = the diameter in millimeters of the 10% passing size (Brajas, 2008). The foaming agent was protein-based lithofoam, sourced from Germany. The water used was potable water. In line with the findings of researchers (Salau et al, 2012), the bamboos with the following characteristics were selected:i) Only bamboos showing a pronounced brown colour were used in order to ensure that the plant is matured and at least three (3) years old.ii) Selection of the longest large diameter culms available was made while as far as possible, straight long bamboos without any deformations and cracks were selected. Bamboos used are free of any decay, fungus growth or holes due to white ants.iii) The bamboos were not cut under wet condition because the culms are generally weaker due to increased fiber moisture content.iv) All main reinforcements consist of bamboo splints with the nodes exposed upwards to increase bond; thus bamboo species with the greatest number of nodes were selected.The selected bamboos were air dried for over 30 days (seasoning in air), and then sawn into strips size of 10 x 10 x 24000mm. In order to reduce water absorption and increase the bond with lightweight aerated concrete matrix, the bamboo strips were coated with bitumen and wound round with 1mm diameter coir rope at a pitch of about 25mm along the strip from end. The coir rope was also coated in hot bitumen after being wound round the bamboo strip. This gave a surface similar to a ribbed steel surface. The ribbed surface was expected to improve the bond considerably and the structural behaviour of the bamboo reinforced aerated concrete. In addition, fine sands were sprinkled over the coats of bitumen with the aim of inducing roughness on its surface. Bamboo reinforcing bars completely coated and sand blasted are shown in Figure (1).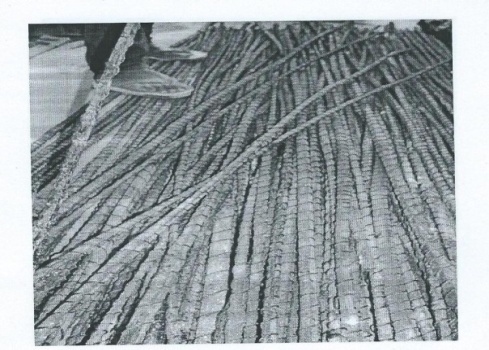 | Figure 1. Treated Bamboo Reinforcing bars |
2.2. Experimental Procedures
The mix proportion for the production of foamed aerated concrete used for this work is cement/sand ratio 1: 3, foaming agent/water ratio of 1: 33, and water/cement ratio of 0.7. The bamboo reinforcing bars were arranged are as shown Figure (2) with the 8mm diameter links spaced at 150mm centers.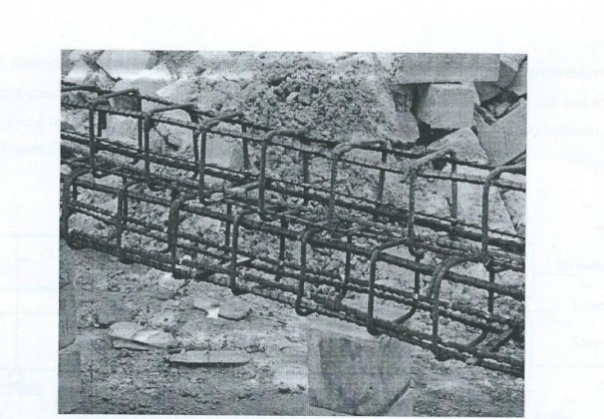 | Figure 2. The arrangement of bamboo in the foamed aerated concrete beam |
The bamboo strips were 10mm x 10mm in section. The average tensile strength of the bamboo strip as determine from the Avery universal tensile machine, was 95N/mm2. Twelve foamed aerated concrete cubes of 150 x 150 x 150 mm were prepared for the determination of the compressive strength at different curing age of 7, 21, 28 and 45 days. Thirty six foamed aerated concrete beams of 225 x 225mm cross-sectional area and 2350mm long reinforced with different percentages of the treated bamboo strips as tensile reinforcement were cast for the determination of the flexural strength, deflection and crack pattern. The thirty six beams were divided into three groups I, II, and III as shown in Figure (3). Group I consists of eight (12) beams with 2Nos of bamboo reinforcement bars at the tension face. Group II consists of eight (12) beams with 4Nos of bamboo reinforcement, while Group III has eight (12) beams reinforced with 6 Nos. of bamboo reinforcement at the tension zone. Three beams in each of the group were tested tin flexure under third point loading at the curing ages of 7, 21, 28 and 45 days. The loading arrangement is shown in Figure (4).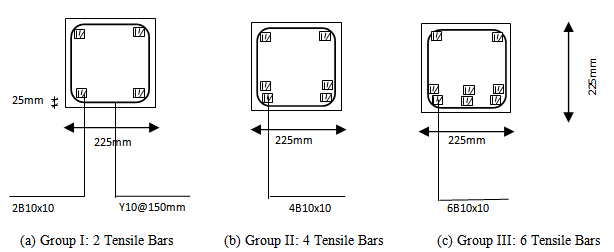 | Figure 3. The cross sections of the three Groups of beams |
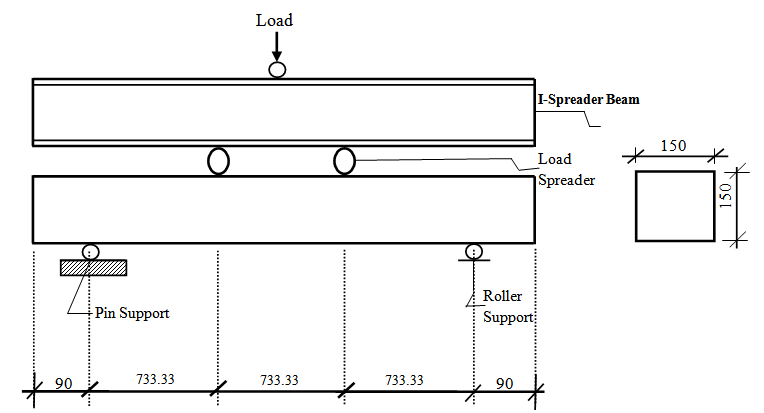 | Figure 4. Typical Beam and Loading Arrangement |
Theoretical Computation of Flexural StrengthThe theoretical moment was calculated for each of the beam specimens using the simplified stress block at the ultimate limit state in accordance with the provision of BS 8110 (1997) for the analysis of reinforced concrete section subjected to pure flexural force. The symbols have been however modified to suit the present work as shown in Figure 5.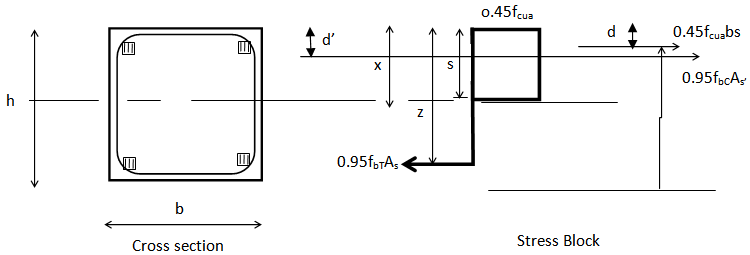 | Figure 5. Simplified Stress block for Foamed aerated concrete beams section under Flexural Forces |
The factor of safety for aerated concrete and bamboo are assumed to be of concrete and steel as defined by BS 8110-1:1997 which are 1.5 and 1.05 respectively.From the simplified stress block, the ultimate moment of resistance can be determined by taking moment at the centre of tensile bamboo reinforcement. | (1) |
The value of s in the equation can be determined from the stress block by considering equilibrium of forces in the section. The governing equation will be | (2) |
Therefore | (3) |
 | (4) |
Utilizing the equation 1 and 4 derived above, the calculations of the simplified depth of compression zone, s, as well as the theoretical ultimate bending moment of resistance for the beams are presented as follows:Group I Beams (with two bamboo splints as tensile bars)Taking the centre of tensile force to be the mid-depth of the double layers of the bamboo reinforcement, d = h – c – diameter of link – (diameter of bar)/2d = 225 – 25 - 10/2 = 185mm.From Equation (4).s = 0.95(95 * 200 – 20 * 200)/ (0.45 * 9.333 * 225)s = 15.08mmSubstituting in Equation (1),Mu = 0.45 * 9.333 * 225 * 15.08 (185 – 15.82/2) + 0.95 * 20 * 200 (185 x 30) x 10 -6Mu = 3.62KNmSimilarly, forGroup II Beams (with four bamboo splints as tensile bars), Mu = 5.65KNmGroup III Beams (with six bamboo splints as tensile bars),Mu = 8.02KNm
3. Results and Discussions
3.1. Preliminary Results
The results of sieve analysis of the sand used are shown in Table (1). Table 1. Some properties of the Sand used 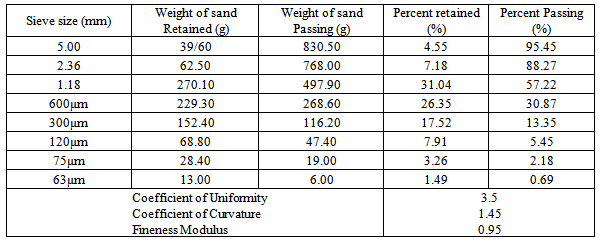 |
| |
|
From the results of the of sieve analysis conducted for the sand, the computed coefficient of uniformity (Cu) and coefficient of curvature (Cc) of the sand were respectively 3.5 and 1.45. Also the fineness modulus was 0.95. As these values fall within the range considered for good quality concrete production (Mindess et al., 2003), it can be concluded that the sand was fine enough, well graded and suitable for foamed aerated concrete production. The densities of the aerated concrete determined from cubes at 7, 21, 28, and 45 days curing were 1674.20kg/m3, 1738.20kg/m3, 1709.10kg/m3, and 1697.5kg/m3 respectively. The seeming decrease in density after 21 days may due to cessation of hydration due to lack of water to cause substantial hydration which would have densified the matrix. However with the average density of 1704.75kg/m3, the foamed aerated concrete used for this investigation fell within the limits defined for lightweight structural concrete (ACI, 2003), which is what this investigation is concerned with. The average compressive strength of aerated concrete is 9.333N/m2. The strength at 45 days of air curing produced the same value as that of 28 days, this really support the fact that concrete achieves appreciable strength at 28 days. The reason the gain in strength of aerated cubes seems to have ceased after 28 days in this experiment might be attributed to non availability of water within the cubes mass for further hydration of cement since it is expected that any water molecule must have been lost to evaporation, even at room temperature, at that curing age. The average tensile strength of bamboo splints was 95N/mm2 which is about 38% of the mild steel. Also bamboo splints showed negligible elongation from the results obtained from all the specimens tested.
3.2. Failure Modes
None of the beams exhibited shear failure. All beams with 2 and 4 number of bamboo reinforcement in tension zone exhibited flexural tension failure of the bamboo reinforcement. This types of failure is identified by many tiny vertical cracks originating from concrete in the tension zone of the beam specimens, and propagating almost vertically upwards in the section towards the compression zone. At failure, one or more visible vertically longer cracks developed at the tension zone. The specimens with four bamboo splints at the tension zone developed more than one visible crack at failure. The cracks were usually longer.Beams with 6 numbers of bamboo reinforcements in tension zones exhibited flexural compression failure by crushing of concrete in compression. As the load is increased, the portion of concrete section above the bamboo splint reinforcement bars in the compression zone became crushed in addition to the development of large vertical cracks in the tension zone, and shear plane developed through which a small upper portion in compressed zone separated from the remaining part of the beam section. The separation of concrete in compression zone might have resulted from one or both of these causes: (i) different compressive capability of the aerated concrete and bamboo reinforcement bars in the compression zone of the beam, (ii) bound failure between aerated concrete and bamboo reinforcement in the compression zone.
3.3. Deflection Characteristics of Beam Specimens
The deflection characteristics of foamed aerated concrete beam specimens reinforced with bamboo splint, with 2, 4, and 6 bars at the tension zone are respectively shown in Figures (6) to (8) and Table (2).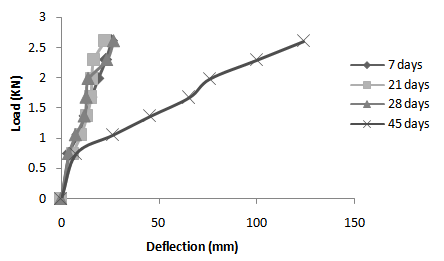 | Figure 6. Load and deflection Characteristic at Different curing Age for Two (2) umbers of Bamboo Reinforcement Bars |
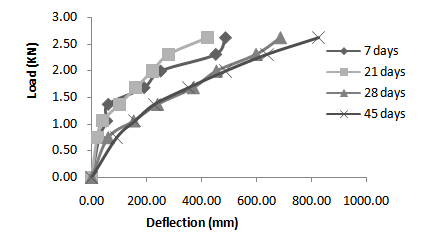 | Figure 7. Load and deflection Characteristics at different curing Age for 4 Numbers of Bamboo Reinforcement Bars |
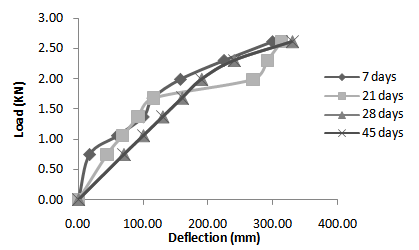 | Figure 8. Load and deflection Characteristic at Different curing Age for 6 Numbers of Bamboo Reinforcement Bars |
Table 2. Deflection of Beam Specimens at Failure at different curing age  |
| |
|
From these Figures and Table, the followings can be observed:Deflection – Before Failurei) The average mid span deflection decreased with increase in the numbers of bamboo in the tensile zone.ii) The deflection increased as the curing age increased irrespective of the numbers of bamboo used as tensile reinforcement in the tensile zone. iii) It can be seen that the shape of load-deflection curve is non-linear before initial crack. This behaviour is expected, because bamboo-reinforced aerated concrete is a composite material of bamboo and foamed aerated concrete, both of which have been found not to exhibit linear stress-strain relationship.Deflection – At Failurei) Maximum deflection increased with curing ages for all the specimens for all the beam specimens. For example, for specimens with 2 bars, the deflections were 5.32 mm, 5.36 mm, 5.44 mm, and 9.12 mm respectively for 7-, 21-, 28-, and 45-day curing. ii) The deflection decrease as the number of bamboo splints used in the tensile zone is increased at all the curing ages. A typical pattern for the specimens at 7-day curing is shown in Figure (9).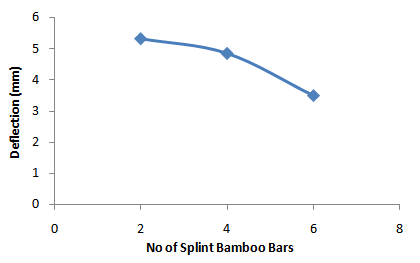 | Figure 9. Variation of Deflection with the number of Bamboo splints At 7 days of curing |
Failure Loadsi) The failure loads decreased as the number of bamboo in the tension zone increased for all specimens, with the exception of specimens cured for 45days. This suggests that no advantage may be derived from increased number of bamboo bars in the tension zone.ii) For the specimens with 2 numbers of bamboo splints at the tension zone, the failure loads are constant at 3.23KN up to 28 days of curing. But this value drop by 20% to 2.61KN after 45 days of curing. The same trend is observed for specimens with 4 numbers of bamboo splints in the tension zone. But for specimens with six numbers, the failure load is constant at 2.92 for all the curing ages. This confirms the deduction made above that no relative structural advantage may be derived by increasing the number of bamboo in the tension zone.
3.4. Flexural Moments
The results of variation in ultimate moments are presented in Table (3). It can be seen that moments observed experimentally increased with curing ages irrespective of the numbers of bamboo splints in the tension zone.Table 3. Experimental Moments for the Beam Specimens at different curing age  |
| |
|
Also, moments increased as the number of bamboo splints in the tension zone increased. This is an indication that increasing the number of bamboo splints in the tension zone improved the bending response, hence the flexural performance of the beam specimens. The experimental and theoretical ultimate moments of resistance for the beams at 28 days of curing are shown in Table (4).Table 4. Experiment and Theoretical Ultimate Moment of Resistance at 28 days  |
| |
|
From Table (4), it can be seen that both the experimental moments and the theoretical moments increased with the number of bamboo splints in the tensile zone. However, the theoretical moments are larger than the experimental moments. Generally, the moments are low. The low theoretical moments can be due to low compressive strengths of foamed aerated cubes. Low compressive strength is characteristics of foamed aerated concrete owing to the presence of voids in the matrix. Voids in the concrete have been found to greatly reduce its compressive strength (Neville, 1996). Low experimental moments can be due to the effect of air curing as oppose to water curing. According to Innovations (2012) and Cadman (2012), testing beams specimens in a dry state results in lower strength. This is because cracks, which contribute to low flexural strength of test specimens, are easily generated and propagated in a dry condition (Neville, 1996). The relationship between the theoretical moments and experimental moments is graphically represented by a scatter plot in Figure (10). With a statistical correlation coefficient of 0.912, it can be seen that there is a positive linear relationship between experimental ultimate moments and theoretical ultimate moments.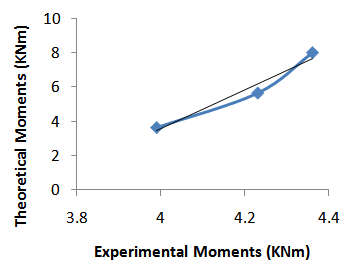 | Figure 10. 28-day Relationship between the Experimental and Theoretical Moment of Bamboo Reinforced Aerated Concrete |
Through regression analysis, this relationship can be expressed mathematically by equation (5) as: | (5) |
where:y = theoretical moment, and x = experimental moment
4. Conclusions
The following conclusions can be drawn from the above experimental work:• The ultimate moment of resistance (experimental and theoretical) of bamboo splints foamed aerated concrete beams increased with increase in the number of bamboo splints in the tensile zone.• The failure loads of beams increased with increase in the number of bamboo splints in the tensile zone.• The deflection of the beam specimens reduced as the number of bamboo bars in the tension zone increased.• The use of bamboo splints as tensile reinforcement in pairs and bundles, as tensile will improve the flexural performance of foamed aerated concrete beams
ACKNOWLEDGEMENTS
We wish to appreciate the Management of the University of Lagos, Nigeria, for providing substantial part of the funds for this research, as well as creating the enabling environment for the execution of the work at the structures and concrete laboratories, Civil and Environmental Engineering Department of the institution.
References
[1] | ACI Committee 213 (2003) “Guide for Structural Lightweight Aggregate Concrete”. American Concrete Institute, Farmington Hills, MI. |
[2] | AdomAsamoah, M. 1, and Afrifa, O. R. (2011) “A Comparative Study of Bamboo-reinforced Concrete Beams Using Different Stirrup Materials for Rural Construction”. International Journal Of Civil And Structural Engineering, Volume 2, No1, pp. 407 – 423. |
[3] | Brajas, M. D. (2008) “Fundamentals of Geotechnical Engineering”. Thompson Learning International Edition, Toronto, pp. 622. |
[4] | British Standard BS 8110. (1997) “Code of Practice for Design and Construction”. British Standards Institution, London. |
[5] | Cadman (2102) “Flexural Strength of Concrete”. http://www.cadman.com (assessed 12/1/2013). |
[6] | Falade, F. & Ikponmwosa, E. (2006). Scope of Bamboo Reinforcement in Concrete Beams for low-Cost Housing. Journal of Construction and Materials Technology, 3(1-2), 39-45. |
[7] | Falade, F. & Ikponmwosa, E. (2008). “An Overview of Foamed Aerated Concrete – A Building and Civil Engineering Construction Material”. Journal of Engineering Science and Technology, Vol. 3, No. 3, pp. 1 – 9. |
[8] | Ghavami, K. (2004). “Bamboo As Reinforcement In Structural Concrete Elements”. Cement and Concrete Composites, Vol. 27, pp. 637 – 649. |
[9] | Innovations (2012) “How is Flexural Strength Calculated”. http://www.innovations.net/print (assessed 14/1/2013). |
[10] | Mindess, S., Young, J. and Darwin, D. (2003) “Concrete”. Pearson International Education, 2nd Edition. |
[11] | Neville, A. M. (1996) “Properties of Concrete”. Wiley, New York. |
[12] | Sakaray, H., Togati, N. V, and Ramana Reddy, I. V. (2012) “Investigation On Properties Of Bamboo As Reinforcing Material In Concrete”. International Journal of Engineering Research and Applications, Vol. 2, Issue 1, pp. 77 – 83. |
[13] | Salau, M.A., Adegbite. I., and Ikponmwosa. E. (2012). “Characteristics Strength of Concrete Column Reinforced with Bamboo Strips”. Journal of Sustainable Development, Vol. 5, No. 2, pp. 133 - 143. |
[14] | Yu, H. Q., Jiang, Z. H., Hse, C. Y. & Shupe, T. F. (2008). “Selected Physical and Mechanical Properties of Moso Bamboo (Phyllostachys pubescens)”. Journal of Tropical Forest Science, 20 (4), 258-263. |