Maged Mohammed1, 2, E. Chakir1, T. El Khoukhi3, H. Boukhal2, M. Azahra2, J. El Bakkali2, Mroan. A. A. Saed2, T. El Bardouni2
1LHESIR, Faculty of sciences, IbnTofail University, Kenitra, Morocco
2Radiations and Nuclear Systems Laboratory, University Abdelmalek Essaadi, Faculty of Sciences, Tetouan, Morocco
3USE, CEN-Maamora, CNESTEN, Rabat, Morocco
Correspondence to: Maged Mohammed, LHESIR, Faculty of sciences, IbnTofail University, Kenitra, Morocco.
Email: |  |
Copyright © 2015 Scientific & Academic Publishing. All Rights Reserved.
Abstract
This work aim to evaluate the impact of the field size on the dosimeric properties of 12MV photon beams generated by a Saturne43 linier accelerator, such as surface dose, mean energy, energy fluence, percent depth dose, quality index, beam profile . We used EGSnrc MC Code for modulated the head of accelerator and simulated the transports of 12MV photon beams. The (5×5,10×10,15×15,20×20,25×25,30×30) cm2 field sizes were examined. We found that the increase in the quality index from 0.608 to 0.673 and surface dose from 13.37 to 33.38 Gy, for 5×5, 30×30 cm2, respectively, increase of the percent depth dose, energy spectra with field size, and decrease in the mean energy with field size. We conclude that it is not recommended to use fields greater than 10×10cm2, particularly in deep tumors. In contrast using small fields allows the dose to be placed very precisely in the tumor volume and at the same time to spare healthy tissue which may be in close vicinity.
Keywords:
Field size, Dosimerric properties, Monte Carlo, Saturn43
Cite this paper: Maged Mohammed, E. Chakir, T. El Khoukhi, H. Boukhal, M. Azahra, J. El Bakkali, Mroan. A. A. Saed, T. El Bardouni, Dosimetric Properties of the Field Sizes of 12MV Photon Beams: A Monte Carlo Study, Journal of Nuclear and Particle Physics, Vol. 5 No. 3, 2015, pp. 52-57. doi: 10.5923/j.jnpp.20150503.02.
1. Introduction
External radiation therapy is efficient methods or more used in the present time to treat different types of cancer.The Monte Carlo method is a computer calculation methods used currently in vast and diverse areas. Its purpose is to provide a statistical solution to a problem that cannot be solved by a simple analytical approach.The fundamental principle of the Monte Carlo method is to estimate the average result of an amount interest generated by a series of stochastic events. In the physical transport of radiation, it describes the history of each particle, penetrating the middle by a single trajectory resulting from a series of interactions, whose probabilities are specific to the environment and the properties of the particle. In dosimetry, we are interested in led energy deposition by a source in a given geometry. The Monte Carlo method is widely used to simulate the trajectory of the particles from a source into a medium in order to obtain the mean energy absorbed by the medium. It is clear that the simulation must involve a large number of particles so that the expected result converges to this average value.It has become a powerful tool for investigating radiation dosimetric parameters and to simulate the transport of particles. It is a common objective of medical physics to achieve an accuracy of better than ±5% for the delivery of dose. But this can be realized only if the dose calculation accuracy is better than ±2%. Therefore, in the future, Monte Carlo algorithms will have a clear preference compared with all other methods of dose calculation. At present, different Monte Carlo codes are used widely for modeling medical linear accelerators [7, 8]. Many studies have been conducted using this method for analyzing linac head components and influencing factors on beam characteristics.In the treatment planning, the field size is one of the most important parameter. Dueit required to determination his size and shape which adequate with tumor volume [13, 15]. In radiotherapy four general groups of field shape are used: square, rectangular, circular and irregular. Square and rectangular fields are usually produced with collimators installed in radiotherapy machines, circular and irregular fields with special collimators attached to the treatment machine [15].In this work we used EGSnrc (MC) code for study an affected of variation a field size on the photon beams generated from a linier accelerator Saturne 43 linear accelerator. This work aims to examine the impact of the field size on the beam characteristics such as quality index, dose distribution, surface dose, and energy spectra.The variation in the field sizes in an external radiotherapy treatment is achieved through the collimator which is a set of two pairs of jaws moving perpendicular symmetrically with respect to the central axis, not diverging, of the photon beam. Modern accelerators linear medical are provided with an asymmetry option of one or two pairs of jaws allowing movement of a jaw independently of the other.
2. Materials and Methods
The BEAMnrc [1] Monte Carlo code has been used to simulate the transport of particles through the head of a Saturne43 accelerator. All the materials and the geometrical data of the accelerator have been provided by the group EURADOS [2].The backage BEAMnrc based on the EGSnrc code is used to perform the build or modeling the head of accelerator and to simulate the transport of particles. The components of the head are the target, the collimator, the flattening filter and the jaws. The phase space files (PS) were generated for each field size from 5×5 to 30×30 cm2, it used such a source in DOSXYZnrc for dose calculation in the water phantom .its includes all the information of the particles, which exit from the linac head, such as energy, position, incident angle and charge. The (PS) was set to 90cm at the target .The parameters were used in this simulation, (ECUT) cut-off energy for electrons =700KeV and (PCUT) energy for photons = 100KeV, (DBS) Directional Bremstrahlung Splitting with (R=20, SSD=60cm and NB=100), ESAVE =1MeV (Energy below which electron will be discard in range rejection) and the forcing photons was used. All simulations were performed using 106 histories. In Figure(1), we show our generated 2D view of the modeled linac head associated to a water phantom with dimension of 40 × 40 × 40 cm3 that was placed at a source to surface distance (SSD) of 90 cm.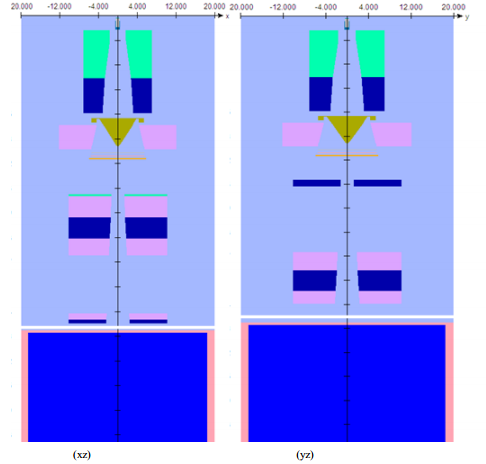 | Figure 1. The schematic representation the simulated geometry of the 12 MV photon beam Saturne43 Linac with phantomusing BEAMnrc |
The DOSXYZnrc [3] based on the EGSnrc code is used to perform the simulation. For calculate the dose distributions in a water phantom with dimension of 40×40×40cm3 was simulated with a source-surface distance (SSD) of 90 cm. A Non-uniform voxel size of 0.25*0.25*0.1cm3 was used in our work. In this parte we used the (PS) files generated by BEAMnrc as sources.In order to analyze the photon energy spectrum or photon beam in the surface of the phantom we used BEADP [4]. (BEAM Data Processor) is a program developed for the OMEGA project, used to analyze the BEAM phase-space data and to derive the spectral and planarfluence distributions for use by beam characterization models. It is very effective in the beam data analysis.
3. Results and Discussion
The statistical uncertainty in our calculations was 0.6%. The mean energy and energy spectral were calculated by BEAMDP from (PS) at z=90cm. The PDDs were measured on the central axis for open square field size from 5 ×5 to 30 ×30 cm2 in a water phantom to SSD=90cm normalized to the dmax and scored on the central axis. The lateral dose profile curves were scored at depth of 10cm in the water phantom.
3.1. Mean Energy and Quality Index
Fig. 2shows the mean energy for different field sizes, we note that the mean energy of spectra decreases with the field size. The increase in field size, allows a larger number and weak photons absorbed by the block to participate in the spectrum of the beam, this leads to decrease of mean energy. Mesbahi (2011) found that the mean energy increase with decrease the field seizes from 1.43 to1.76 MeV for 30 ×30 to 10×10 cm2 field sizes, respectively, for 6MV photon beams [5]. 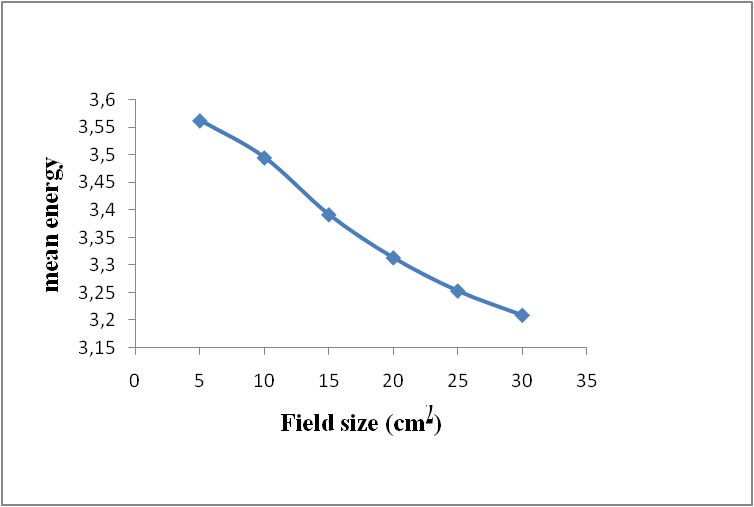 | Figure 2. Mean energy of photon energy spectra with field size |
Also the quality index a widely accepted measure of beam quality and ensure that energy radiation has not changed largely, it is defined as the ratioTPR20, 10 of doses at two depths in water (20cm and 10cm). It increase with field size from 0.608 to 0.673 for 5×5, 30×30 cm2 respectively. Due the attenuation of photon beams increase in small field size and it is dependent on the electrons contaminations [5, 9]. The quality index is dependent on beam energy and it increases linearly with photon beam energy. The mean energy of energy spectra for different field sizes are seen in table 1.Table 1. Mean energy of photon energy spectra for (5×5, 10×10, 15×15, 20×20, 25×25, 30×30) cm2 field sizes 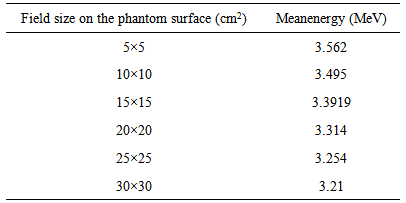 |
| |
|
3.2. Percentage Depth Dose at 10 cm
The value of percentage depth dose at 10 cm (%DD10) considered as a beam quality indicator and is supported to measure the absolute dose in AAPM TG 51[9, 10]. It includes the impacts of contamination of electrons on the photon beam [10]. In this study. We found that its value increase laniary with field size from 73 to 76.4 Gy for 5×5 to 30×30 cm2 respectively. This is a small increase and indicate that the contamination of electrons does not change significantly with increasing the field.
3.3. PDDs at the Central Axis and Surface Dose
The curves for (5×5, 10×10,15×15, 20×20,25×25 and 30×30 cm2) field sizes are shown in Fig.3.(a) Percent depth dose, it’s normalized to dmax; (b) surface dose, it normalized at depth 10 cm. In (3, a) we notice that the dmax equal for all the curves, it consistent with the measured (2.5cm). Due that not change the amount of particles in the central axis, after the dmax we found that the curves rise or increase the dose rate on the beam axis with increase the field size. Due a contribution of Compton photons scattered by the remainder of the irradiated volume. It increase as a function of depth and consequently the effective attenuation will be less. The contribution no change with small field size.Many parameters effect on the surface dose one of these is a field size. The surface dose was obtained within the first 1mm inside the water phantom on the central axis. The results obtained in this work show (3, b) the surface dose increase with the fieldsize. We found that it increase from 13.37 to33.38 Gy for 5 ×5 to 30×30 cm2 field size respectively, and increasing in the build up region. Reduce the field size contributes to the arrest or the absorption of a large amount of diffuse radiation, leading to reduced energy deposited in the surface. Wang (2012); Klein (2002) found that the surface dose increased linearly with field size [11, 12]. Due to increased electrons contaminated with field size. The large field size is useful for treat the large size of skin cancer.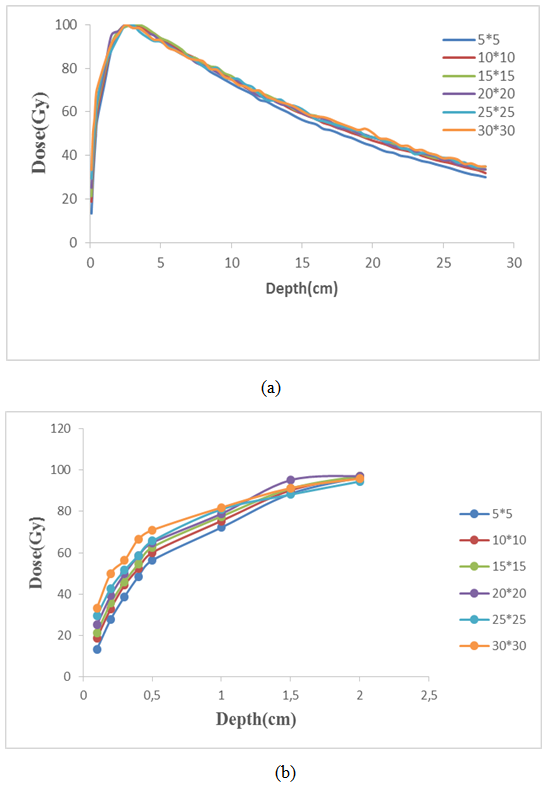 | Figure 3. The comparison between the relative central-axis (a) depth-dose curves (b) surface doses for (5×5, 10×10, 15×15, 20×20, 25×25 and 30×30 cm2) field sizes in water phantom |
3.4. Beam Profile
Lateral dose profiles were measured at a depth of 10cm in the water phantom. Fig. 4 shows the Profiles obtained at different field sizes (5×5, 10×10, 20×20, 25×25 and 30×30 cm2).We noted that the beam profile increase with field size, this is normal, because it increases the width of the photon beam. The ratio between the maximum and minimum dose was measured within 80% is 1.1 for all field sizes. Although this value is equal, but the large size has negative effects on healthy tissue adjacent.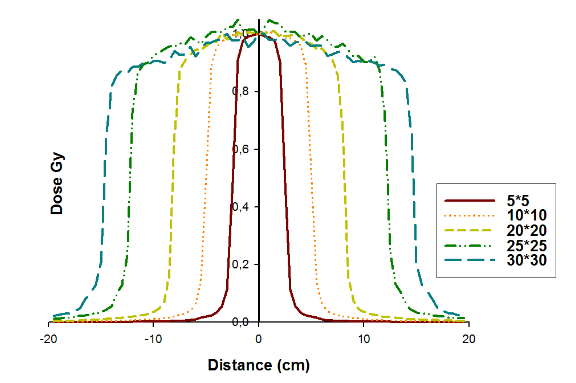 | Figure 4. Variation of beam profiles with field sizes of 12MV photon beams |
3.5. Total Scatter Factor (Sc,p)
It’s defined as the ratio of the dose at a reference depth in a phantom for a given field size r to the dose at the same point and depth in a phantom for the reference field size (10 ×10 cm2) [10]. Sc,p increases with field size from 0.947 to 1.14 for 5 ×5 to 30×30 cm2 field size respectively its reported in table (2) and presented in figure (5). Vassiliev (2006) found that Sc,p increases with field size of 6 MV and 18 MV photon beams from a Varian Clinac 21EX accelerator[14].Table 2. The total scatter factor Scp of 12MV photon for (5×5, 10×10, 15×15, 20×20, 25×25 and 30×30 cm2) field sizes. The Sc,p was measured at SSD = 100 cm, and at the depth of maximum dose of a 10 × 10 cm2 field 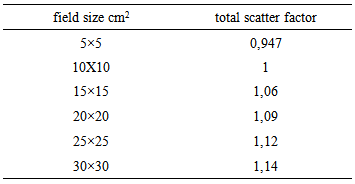 |
| |
|
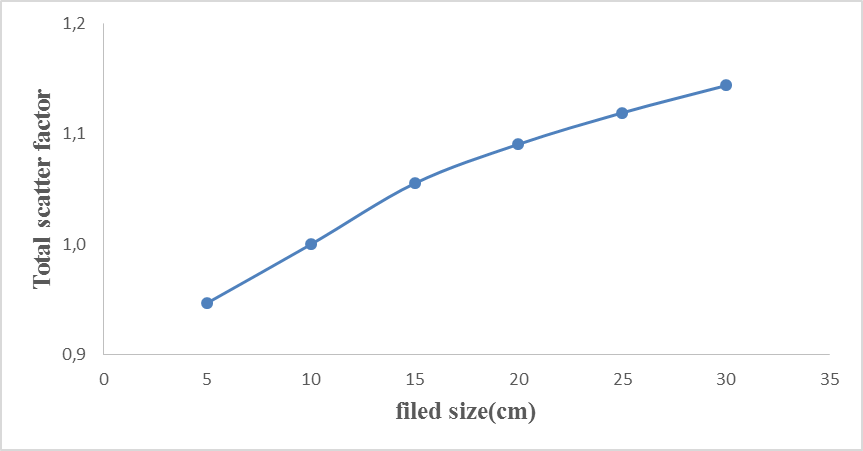 | Figure 5. Variation of total scatter factor with field size |
3.6. Photon Energy Spectra, Spectral Distribution
In fig.6 shows (a) the energy spectra and (b) the Energy fluence distribution for 5×5, 10×10,15×15,20×20, 25×25, 30×30 cm2 field sizes. The Photon energy spectra, Spectral Distribution were measured at a z=90cm that phase space file was placed. We conclude thatitincreases with field size, this is due the more attenuation in the small field per collimator secondary (jaws) or more photons penetrate in large field size [7]. 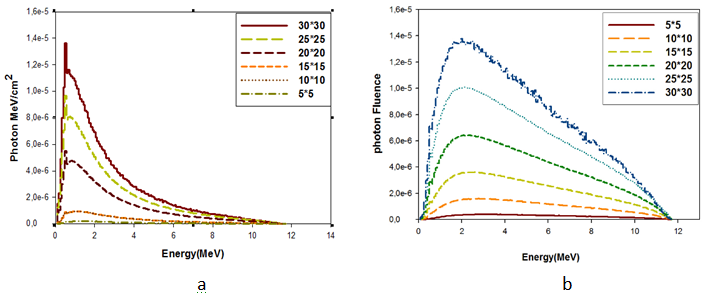 | Figure 6. The comparison between (a) Photon energy spectra, (b) Energy fluence distribution for (5×5, 10×10, 15×15, 20×20, 25×25 and30×30) cm2 field sizes |
4. Conclusions
The Monte Carlo method is very accurate for dose calculation in radiotherapy and studied beam characteristics. The impacts of modification of field size on the photon beams characteristics was studied in this work. We conclude that the increase of field size lead to decrease the mean energy and increase quality index, energy spectra, beam profile and Percent depth dose. This due to increase the contamination of the electrons emitted by collimator or air. We found that the quality index increased from 0.608 to 0.673 and surface dose from 13.37 to 33.38 Gy, for 5×5, 30×30 cm2, respectively. The small field size is useful and very accurate for treat the tumors. At the same time maintain a parts and surrounding tissues. We suggest conducting the same study with use MLC and without flattening filter.
References
[1] | D. W. O. Rogers, B. Walters, and I. Kawrakow, “BEAMnrc User’s Manual.” NRC Report PIRS 509(A) revL (2013). |
[2] | WORKING GROUP6: COMPUTATIONAL DOSIMETRY, Standard Monte Carlo Modeling of a Medical Linear Accelerator, 2010. |
[3] | B. Walters, I. Kawrakow and D.W.O. Rogers, “DOSXYZnrc User’s Manual,” NRCC Report PIRS-794revB, 2013. |
[4] | C.-M. Ma and D.W.O. Rogers, “BEAMDP User’s Manual”, NRCC Report PIRS-0509(C) revA, 2013. |
[5] | A. Mesbahi, 2007, “Dosimetric characteristics of unflattened 6 MV photon beams of a clinical linear accelerator: A Monte Carlo study,” Applied Radiation and Isotopes 65, 1029–1036. |
[6] | K. Abdul Haneefa, T. Siji Cyriac, M. M. Musthafa, R. Ganapathi Raman, V. T. Hridya, A. Siddhartha, and K. K. Shakir, FLUKA Monte Carlo for Basic Dosimetric Studies of Dual Energy Medical Linear Accelerator, Journal of Radiotherapy, 2014 , Article ID 343979. |
[7] | WHO, PAHO and ESTRO, Absorbed Dose Determination in External Beam Radiotherapy An International Code of Practice for Dosimetry Based on Standards of Absorbed Dose to Water, INTERNATIONAL ATOMIC ENERGY AGENCY, VIENNA, 2000, STI/DOC/010/398. |
[8] | Aldo Barreras Caballero, Rodolfo Alfonso Laguardia, DEVELOPMENT AND COMMISSIONING OF A MONTE CARLO PHOTON BEAM MODEL FOR ELEKTA SL LINEAR ACCELERATOR ,VIII Congreso Regional de Seguridad Radiológica y Nuclear, Octubre de 2010, Medellín Colombia. |
[9] | A. Pichandi, Kadirampatti Mani Ganesh, Amalraj Jerin, Karunakaran Balaji, Gurunath Kilara, Analysis of physical parameters and determination of inflection point for Flattening Filter Free beams in medical linear accelerator.reports of practical oncology and radiotherapy 1 9 ( 2 0 1 4 ) 322–331. |
[10] | Peter R. Almond, Peter J. Biggs, B. M. Coursey, W. F. Hanson, M. Saiful Huq, Ravinder Nath, D. W. O. Rogers, AAPM’s TG-51 protocol for clinical reference dosimetry of high-energy photon and electron beams, Med. Phys. 26 9.September 1999. |
[11] | Klein EE, Esthappan J, Li Z. Surface and buildup dose characteristics for 6, 10, and 18 MV photons from an Elekta Precise linear accelerator. J Appl Clin Med Phys 2003;4:1-7. |
[12] | Wang, Y., Khan, M.K., Ting, J.Y., Easterling, S.B., 2012. Surface dose investigation of the flattening filter-free photon beams. Int. J. Radiat. Oncol. Biol. Phys.83 (no.2), 281–285. |
[13] | Faiz. M. Khan, the of radiation therapy, 2nd ed. University of Minnesota medical School, 1992. |
[14] | Vassiliev, Uwe Titt, Falk Ponisch, Stephen F Kry, Radhe Mohan and Michael T Gillin, Dosimetric properties of photon beams from aflattening filter free clinical accelerator, Phys. Med. Biol. 51 (2006)1907–1917doi:10.1088/0031-9155/51/7/019. |
[15] | E.B. Podgorsak, Radiation Oncology Physcs: A handbook for teachers and students, international atomic energy agency (IAEA), Vienna,2005. |