M. Mehade Hasan1, M. I. Ali2, D. Paul2, M. A. Haydar2, S. M. A. Islam1
1Department of Physics, Jahangirnagar University, Savar, Dhaka-1342, Bangladesh
2Health Physics and Radioactive Waste Management Unit (HPRWMU), Institute of Nuclear Science and Technology (INST), Atomic Energy Research Establishment (AERE), Bangladesh Atomic Energy Commission (BAEC), Savar, Dhaka, Bangladesh
Correspondence to: M. Mehade Hasan, Department of Physics, Jahangirnagar University, Savar, Dhaka-1342, Bangladesh.
Email: |  |
Copyright © 2012 Scientific & Academic Publishing. All Rights Reserved.
Abstract
The activity concentrations of naturally occurring radionuclides 226Ra, 232Th and 40K in soil and water samples collected from Barapukuria 2×125 MW Coal Fired Thermal Power Plant area situated in Dinajpur district of Bangladesh were measured using gamma ray spectrometry technique. Two types of soil samples namely, active and passive soil samples and both surface and underground water samples were analysed under the current study. The average activity concentrations of 226Ra, 232Th and 40K in active soil samples were found to be 27.89±5.15, 41.52±6.19 and 632.45±133.06 Bqkg-1, respectively and the values were 32.82±6.48, 56.01±8.50 and777.41±168.39 Bqkg-1, respectively in the passive soil samples. These results are slightly higher than the worldwide average values of 35, 30 and 400 Bqkg-1, respectively for corresponding radionuclides except the 226Ra for both active and passive soils. The activity contents of the water samples did not show any evidence of enhancement due to the power plant operation and the mining activities near the sample collection area. The average absorbed dose rate (D) and outdoor annual effective dose (E) were observed to be 64.52 nGyh-1 and 0.078 mSvyr-1, respectively in the active and 81.65 nGyh-1 and 0.10 mSvyr-1, respectively in the passive soil samples. The average absorbed dose rates were higher than worldwide average values for both active and passive soil samples. Whereas, the radium equivalent activity and external hazard indices were less than the world average values.
Keywords:
Natural radioactivity, Activity concentration, High-purity germanium (HPGe) detector, Gamma-ray spectrometry, Active soil, Passive soil, Radium equivalent activity, Gamma absorbed dose rate, Annual effective dose rate, External hazard index
Cite this paper: M. Mehade Hasan, M. I. Ali, D. Paul, M. A. Haydar, S. M. A. Islam, Natural Radioactivity and Assessment of Associated Radiation Hazards in Soil and Water Samples Collected from in and around of the Barapukuria 2×125 MW Coal Fired Thermal Power Plant, Dinajpur, Bangladesh, Journal of Nuclear and Particle Physics, Vol. 4 No. 1, 2014, pp. 17-24. doi: 10.5923/j.jnpp.20140401.03.
1. Introduction
There are many sources of radiation in the environment. Gamma radiation emitted from naturally occurring radionuclides, also called terrestrial background radiation, represents the main external source of exposure to human body. Human beings are exposed to radiation mainly from cosmic rays and from the gamma ray emitters in soils, building materials, water, food, and air. Investigation concerning the level of radionuclide distribution in the environment provides essential radiological information[1]. Radionuclides have been essentially present in the environment since the creation of the earth. Only nuclides with half-lives comparable to the age of the earth or their corresponding decay products such as 40K, and the radionuclides from the 238U and 232Th series[2] can still be found on earth. Human beings are primarily exposed to ionizing radiations emitted from naturally occurring radioactive materials found in earth’s crust as well as from man-made sources[3].The radioactivity concentrations in soil give information on both natural and man-made sources which is important in radiological monitoring and assessment of radiation dose for public[4]. Measurement of natural radioactivity in soil is very important to determine the amount of change of the natural background activity with time as a result of radioactivity release. Studies of natural radioactivity are necessary not only for their radiological impact but also for their ability to act as excellent biochemical and geochemical traces in the environment. Though natural radioactivity is found in rocks and soils throughout the earth, the accession in specific areas varies relatively within narrow limits[5]. Natural sources contribute almost 80% of the collective radiation exposure of the world’s population[6]. Therefore, great interest has been expressed worldwide for the study of naturally occurring radiation sources as well as environmental radioactivity has led to the interest in extensive surveys in many countries. Bangladesh has a good amount of coal deposit in its territorial area. Especially the northern part of the country is rich in coal deposit. It has already discovered five coal mines in its territory. These are namely; Barapukuria, Phulbari, Khalashpir, Jamalganj and Dighipara and all these mines are located in the northern region of the country. The coals extracted from these mines are used in various purposes including generation of electric power, burning of bricks and so on[7]. Among these coal mines only the Barapukuria coal mine is currently under production. A 2×125 MW coal-fired thermal power plant has been established adjacent to the Barapukuria coal mine site in order to use the coal extracted from this mine as the feed coal for its power generation purpose. During the operation, a coal-fired power plant produces two types of coal ashes namely; bottom ash and fly ash which being mixed with the surrounding soil and water may produce hazardous effect on these environmental elements. Moreover, contamination may occur in the surrounding environmental components such as soil and water due to the leaching of radionuclides caused by power generation activities. A very little data of radiological impacts on the people and environment around the coal-fired power plants in Bangladesh is available. Therefore, this study was designed to conduct a thorough study on the radiological implications of the power plant on its workers, public and surrounding environment.
2. Methods and Materials
2.1. Study Area
Dinajpur is an administrative district under the Rangpur Division and is situated in the Northern part of Bangladesh. It is bounded by Thakurgaon and Panchagarh districts in the north, Gaibandha and Joypurhat districts in the south, Nilphamari and Rangpur districts in the east and the state of West Bengal, India in the west. Figure 1 shows the location map of the study area. The Barapukuria power Plant site is located in flat land of the Northwestern "corner" of Bangladesh at about 45 km east of the district headquarters of Dinajpur and 20 km east from the border of India. The north-south gauge railway passes through the east of the site. The site is located at about 1 km north of the coal mine mouth under construction. The only large industrial area nearby is this coal mine including its residential complexes as seen in Figure 2. The nearest town is Phulbari, a Thana (Upazila) headquarter and primary administrative centre. Phulbari is located about 6 km south of the site. 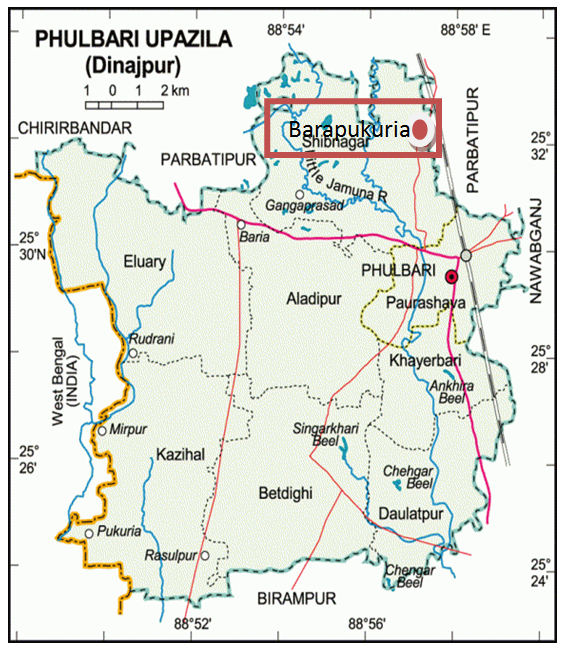 | Figure 1. Location map of the Barapukuria 2×125 MW coal fired thermal power plant in Bangladesh |
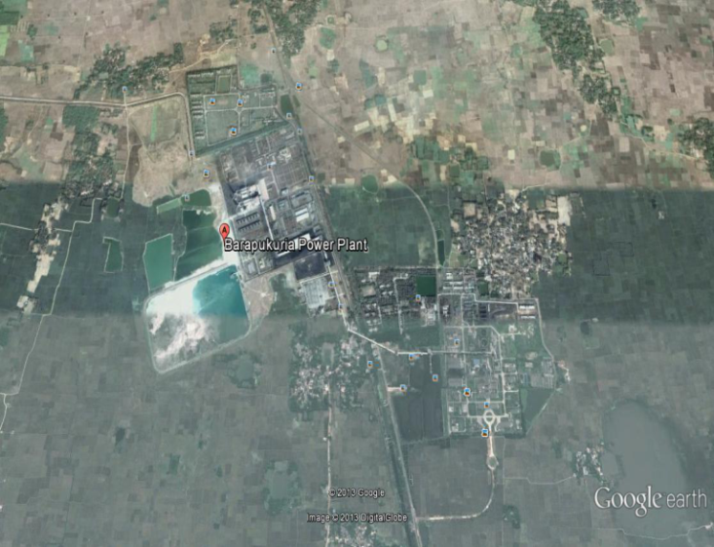 | Figure 2. Location map of the Barapukuria 2×125 MW coal fired thermal power plant in Bangladesh including its residential complexes |
2.2. Sampling Locations
A total of 11 samples namely; 8 soil and 3 water samples; were collected from in and around of the power plant area. Eight soil samples were divided into two groups namely; active and passive soil samples. Moreover, among three water samples, one was fresh pond water, another one was chemical and fly ash mixed pond water and the rest one was underground (tap) water used for drinking purpose. These samples were collected during the whole day on 9 July 2013. Location coordinates of the Barapukuria 2×125 MW coal fired thermal power plant are latitude 25º33´N and longitude 88º 57´E.
2.3. Sample Collection and Preparation
It was stated in the earlier section that 8 soil samples and 3 water samples were collected from in and around the power plant area. Active soil samples were collected from the four sides of the bottom ash storage pond with an approximate distance of 10 meter from the pond and were marked as AS-1, AS-2, AS-3 and AS-4. Passive soil samples were collected from approximately 1000 meter distance from the bottom ash storage pond and were marked as PS-1, PS-2, PS-3 and PS-4. Water samples were collected from the ponds and deep tube-well surrounding the power plant area and were marked as PW-1 (fresh pond water), PW-2 (chemical and fly ash mixed pond water) and TW-3 (tap water).
2.3.1. Processing of Solid Samples
After collection, packaging and marking all the samples were transported to and preserved at the sample processing laboratory of the Health Physics and Radioactive Waste Management Unit, Bangladesh Atomic Energy Commission (BAEC), Savar, Dhaka. Each sample was then cleaned and dried in the sun separately and crushed into fine powder by using a grinder. The samples were then mixed homogeneously and passed through a sieve of 400 µm mesh size. The homogenized soil samples were then dried in an oven at 110˚C for about 24 hours. The samples were then transferred to sealable cylindrical plastic containers of 7 cm height and 5.5 cm in diameter and the weights of the samples were recorded using an electrical balance. The sample-filled containers were marked individually with identification parameters e.g., name and location of the sample, date of preparation and net weight. The containers were sealed tightly with insulating tape around their opening for impeding the possibility of moisture contamination. In order to maintain radioactive secular equilibrium between 226Ra and its daughter products, the sealed containers were stored for a period of 4 weeks.
2.3.2. Processing of Liquid Samples
Marinelli type beakers (2 litre in capacity) were used to process and measure the water samples. At the start of the sample processing steps, the beakers were made contamination-free by cleaning those well using light hydrochloric acid solution and de-ionized water. The beakers were then dried using a temperature-controlled oven and filled with appropriate amount of water sample. Finally, the sample-filled beakers were closed by caps, wrapped with thick vinyl tape around their necks and kept for 4 weeks for achieving the secular equilibrium between gaseous and non-gaseous decay products of naturally occurring radionuclide series.
2.4. Measurement Set-up
The detection and measurement of radionuclides in the samples were carried out by gamma spectrometry system using a vertical coaxial cylindrical high purity germanium (HPGe) detector of 172 cm3 active volume and with 40% relative efficiency. The p-type HPGe detector supplied by CANBERRA (Model- GC4020) had a resolution of 2 keV at 1332 keV of Cobalt-60 gamma-ray line. The detector was coupled to a 16 k-channel analyser. The spectra of all samples were perfectly analysed using Genie-2000 spectra analysis software (which matched various gamma energy peaks to a library of all possible radionuclides) to calculate the concentrations of 226Ra, 232Th and 40K. The detector was enclosed in a cylindrical shielding container made of lead and iron with 11.3 cm thickness, 51 cm height and 28 cm internal diameter and having a fixed bottom and moving cover to reduce the external gamma-ray background. All the samples were counted for 10 ks. Prior to the measurement of the samples, the environmental gamma background at the laboratory site was determined with an identical empty Marinelli beaker and plastic container used in the sample measurement. The energy regions selected for the corresponding radionuclides were 295 keV and 352 keV of 214Pb and 609 keV, 1120 keV and 1764 keV for 214Bi for 226Ra, 583 keV and 2614 keV of 208Tl, 911 keV and 969 keV of 228Ac for 232Th, and 1460 keV for 40K[8].
2.4.1. Calibration of the Detector
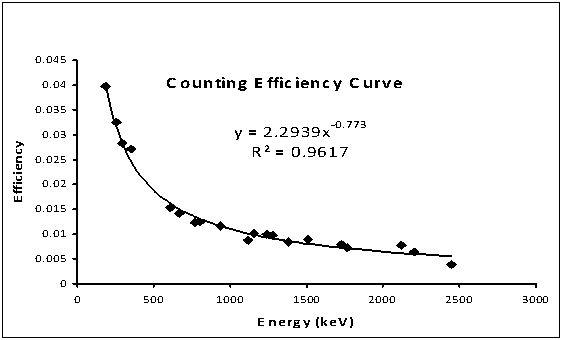 | Figure 3(a). Efficiency curve of the HPGe detector for liquid matrix |
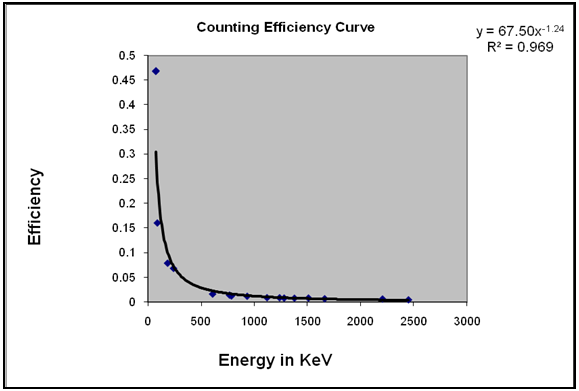 | Figure 3(b). Efficiency curve of the HPGe detector for solid matrix |
The efficiency calibration of the detector was performed by standard sources of solid and liquid matrices prepared using 226Ra standard using identical containers used for the measurement of the samples, e.g., 2L Marinelli beaker for liquid and 180 ml plastic container for solid samples. The preparation process of standard sources had been reported elsewhere[9]. The detector efficiency calibration curves as a function of energy for both liquid and solid matrices are shown in Figure 3(a) and Figure 3(b). The energy calibration of the detector was performed by 137Cs and 60Co point sources.
2.5. Activity Concentrations of Soil and Water Samples
The radionuclide contents and their activity levels in the samples were measured using a calibrated HPGe detector. The activity concentration (A) of each radionuclide in the samples was determined by using the net count (cps) (found by subtracting the background counts from the gross counts with same counting time under the selected photo peaks), weight of the sample, the photo-peak efficiency and the gamma intensity at a specific energy as[10]: | (1) |
Where, A = Activity concentrations of the sample in Bqkg-1 or BqL-1.cps = The net counts per second= cps for the sample- cps for the background valueE = The counting efficiency of the gamma energyI = Absolute intensity of the gamma ray and W = Net weight of the sample (in kilogram, kg or litre, L).The errors in the measurement were expressed in terms of standard deviation (±2σ), where σ is expressed as[11]: 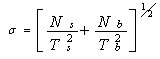 | (2) |
Where, Ns is the sample counts measured in time Ts, and Nb is the background counts measured in time Tb. The standard deviation ±2σ in cps was converted into activity in Bqkg-1 according to equation (1).
2.6. Absorbed Dose Rates
The external outdoor absorbed gamma dose rates due to terrestrial gamma rays from the nuclides 226Ra, 232Th and 40K at 1m above the ground level were calculated as[11]: | (3) |
Where, ARa, ATh and AK are the specific activities of 226Ra, 232Th and 40K, respectively in Bqkg-1.
2.7. Outdoor Annual Effective Dose
The absorbed dose rate was converted into annual effective dose equivalent by using a conversion factor of 0.7 SvGy-1 recommended by the UNSCEAR 2000 and 0.2 for the outdoor occupancy factor by considering that the people on the average, spent 20% of their time in outdoors[12]. The effective dose due to natural activity in the soil samples was calculated by: | (4) |
2.8. Radium Equivalent Activity
The radionuclides 226Ra, 232Th and 40K are not homogeneously distributed in soil. The inhomogeneous distribution of naturally occurring radionuclides is due to disequilibrium between 226Ra and its decay products. For uniformity in exposure estimates, the radionuclide concentrations are defined in terms of ‘Radium equivalent activity’ (Raeq) in Bqkg-1. This allows comparison of the specific activity of materials containing different amounts of 226Ra, 232Th and 40K according to Beretka and Mathew[13] as follows: | (5) |
Where, ARa, ATh and AK are the specific activities of 226Ra, 232Th and 40K, respectively in Bqkg-1.
2.9. External Hazard Index
Local soil of the area is used for the construction of houses and also for agricultural purposes. These soils may contribute to the external gamma dose rates to the public. The external hazard index (Hex) is the indoor radiation dose rate due to the external exposure to gamma radiation in construction materials of dwelling which is calculated by[14]: | (6) |
Where, ARa, ATh and AK have the same meanings as in equation (3), and (5). The value of Hex must be lower than unity in order to keep the radiation hazard insignificant.
3. Results and Discussions
The results of the present study on the two types of samples are summarized in following sections.
3.1. Activity Concentration in Active Soil Samples
Activity concentrations for 226Ra, 232Th and 40K nuclides in active soil samples were determined by equation (1) and the results for the same are shown in Table 1 with the uncertainty level of ±2σ. The results for the radionuclides (226Ra, 232Th and 40K) are also shown independently in Figure 4, Figure 5 and Figure 6, respectively.Table 1. Activity Concentration in (Bqkg-1) in Different Active Soil Samples 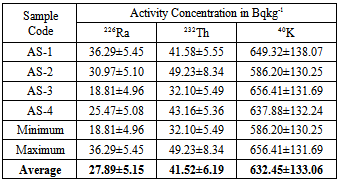 |
| |
|
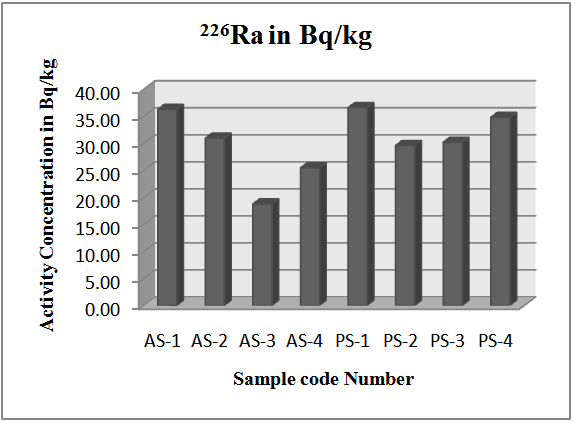 | Figure 4. Distribution of 226Ra in all soil samples |
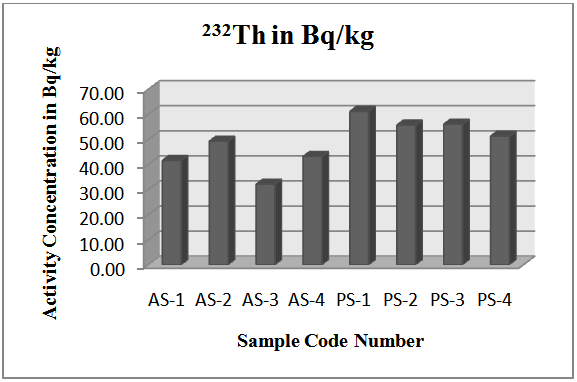 | Figure 5. Distribution of 232Th in all soil samples |
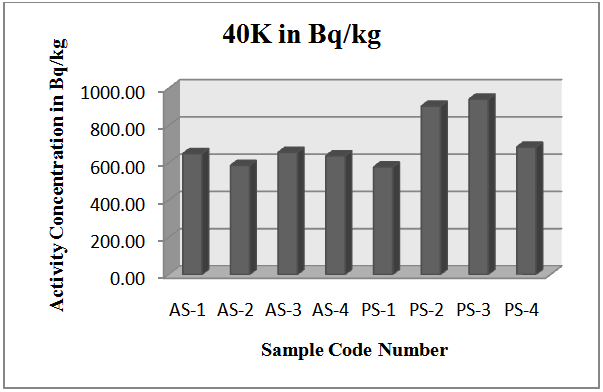 | Figure 6. Distribution of 40 K in all soil samples |
3.1.1. Activity Concentration of 226Ra
In active soil samples, the activity concentrations of 226Ra were found in the range of 18.81±4.96 to 36.29±5.45 Bqkg-1, with an average value of 27.89±5.15 Bqkg-1. This value was less than the worldwide average value of 35 Bqkg-1[11].
3.1.2. Activity Concentration of 232Th
The activity concentration of 232Th was found in the range of 32.10±5.49 to 49.23±8.34 Bqkg-1, with an average value of 41.52±6.19 Bqkg-1. This value was higher than that of world average value of 30 Bqkg-1[11].
3.1.3. Activity Concentration of 40K
The activity concentrations of 40K were in the range of 586.20±130.25 to 656.41±131.69 Bqkg-1, with an average value of 632.45±133.06 Bqkg-1. This value was significantly higher than that of the worldwide average value of 400 Bqkg-1[11].
3.2. Activity Concentration in Passive Soil Sample
Activity concentrations for radionuclides 226Ra, 232Th and 40K in passive soil samples were determined by equation (1) and the results for the same are shown in Table 2 with the uncertainty level of ±2σ. The results for the radionuclides (226Ra, 232Th and 40K) are also shown in Figure 4, Figure 5 and Figure 6, respectively.Table 2. Activity Concentration (in Bqkg-1) in Different Passive Soil Samples 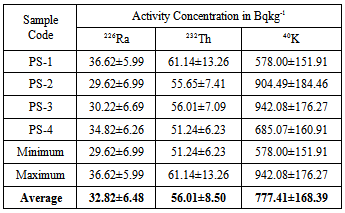 |
| |
|
3.2.1. Activity Concentration of 226Ra
In passive soil samples, the activity concentrations of 226Ra were found in the range of 29.62±6.99 to 36.62±5.99 Bqkg-1, with an average value of 32.82±6.48 Bqkg-1 which was comparable to the worldwide average value of 35 Bqkg-1[11].
3.2.2. Activity Concentration of 232Th
The activity concentration of 232Th was found in the range of 51.24±6.23 to 61.14±13.26 Bqkg-1, with an average value of 56.01±8.50 Bqkg-1 which was also significantly higher than the world average of 30 Bqkg-1[11].
3.2.3. Activity Concentration of 40K
The activity concentrations of 40K were found in the range of 578.00±151.91 to 942.08±176.27Bqkg-1, with an average value of 777.41±168.39Bqkg-1 which was again significantly higher than the worldwide average value of 400 Bqkg-1[11].
3.3. Activity Concentration in Water Samples
The radionuclides in almost all the water samples were below the detection limit. Therefore, the activity contents of the water samples collected from the surrounding areas of the power plant did not show any evidence of enhancement of radioactivity due to the operational activities of the power plant.
3.4. Radiological Indices
In order to assess the health effects, the quantities such as absorbed dose rate (D), outdoor annual effective dose (E), radium equivalent activity (Raeq) and external hazard index (Hex) have been calculated from the activity concentrations of 226Ra, 232Th and 40K using equations (3), (4), (5) and (6), respectively and the values are shown in Table 3.The results shown in Table 3 depict that the absorbed dose rates due to the terrestrial gamma rays at 1m above the ground are in the range of 55.65 to 69.15 nGyh-1 with an average of 64.52 nGyh-1 for active soil samples and are in the range of 75.81 to 87.36 nGyh-1 with an average value of 81.65 nGyh-1 for those of passive soil samples. Both of these values are higher than the world average value of 55 nGyh-1 [11].Table 3. Absorbed dose rate (D), outdoor annual effective dose (E), radium equivalent activity (Req) and external hazard index (Hex) of all active and passive soil samples 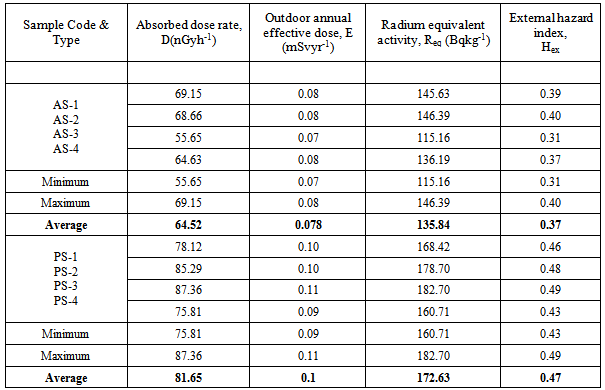 |
| |
|
The outdoor annual effective doses are in the range of 0.07 to 0.08 mSvyr-1 with an average of 0.078 mSvyr-1 for active soil samples and from 0.09 to 0.11 mSvyr-1 with an average value of 0.1 mSvyr-1 for passive soil samples; which are significantly lower than the world average value of 0.460 mSvyr-1[11].The radium equivalent activity varied from 115.16 to 146.39 Bqkg-1 with an average value of 135.84 Bqkg-1 for active soil samples, and from 160.71 to 182.70 Bqkg-1 with an average value of 172.63 Bqkg-1 for passive soil samples. However, all the values for radium equivalent activity obtained here fall far below the limit of 370 Bqkg-1[15]. It is apparent that the radium equivalent activity originating from different regions shows some variations, which are likely to be related to the position of collected soil samples, transport process, etc. This is important in selecting suitable soils not only for construction but also for agriculture purposes in order to keep the radiation hazard minimum.The external radiation hazard index ranged from 0.31 to 0.40 with an average value of 0.37 for active soil samples and from 0.43 to 0.49 with an average value of 0.47 for passive soil samples which are far less than the unity indicating the non-hazardous category of the samples. The values of hazard indices confirm that it is safe to carry out the activities in the area for the power plant workers and general public and moreover, no significant radiological impact of power plant operation is seen on the surrounding environment.
4. Conclusions
The radionuclide contents, activity concentrations and radiological impact of the soil and water samples collected from the Barapukuria coal fired thermal power plant in Dinajpur district of Bangladesh and its adjacent areas were investigated in the present study. The results indicated that only the natural radionuclides were present in the samples. The natural radioactivity concentrations of 226Ra, 232Th and 40K were relatively higher than the world average values except in 226Ra for active and passive soil samples. The values of mean absorbed dose rate, outdoor annual effective dose and the radium equivalent activity were lower than the global average values; whereas the external hazard indices were found less than unity which indicated that there was no probability of immediate health effect on workers and public due to natural radioactivity present in the samples. Moreover, adjacent environmental components fell far below the hazard level as no trace of significant enhancement of radioactivity concentration were observed due to the activities of power plant operation.
ACKNOWLEDGEMENTS
The authors are thankful to Barapukuria 2×125 MW coal fired thermal power plant authority for giving the permission to collect the samples, specially Mr. Dinesh Chandra Mistry, Executive Engineer (coal & ash) for his kind help to collect the samples. The authors also like to thank the technical support staffs of HPRWMU for their help in conducting the study.
References
[1] | A. S. ALAAMER, Assessment of Human Exposures to Natural Sources of Radiation in Soil of Riyadh, Saudi Arabia, Turkish J. Eng. Env. Sci., 32 (2008), 229 – 234. |
[2] | O. Abu Haija, Determination of Natural Radionuclides Concentrations in Surface Soil in Tafila/Jordan, Modern Applied Science, Vol. 6, No. 3, March 2012. |
[3] | Tabassum Nasirm, Huda Al-Sulaiti and Patrick Henry Regan, Assessment of Radioactivity in Some Soil Samples of Quatar by Gamma-Ray Spectroscopy and the Derived Dose Rates, Pak. J. sci. ind.res.Ser.A:phys.sci.2012 55(3)128-134. |
[4] | EPA, 2007. United States. Ionising Radiation, Fact Book. Environmental Protection Agency, March 2007. EPA-402- F-06-061. |
[5] | Surinder Singh, Baldev Singh, AjayKumar, Natural radioactivity measurements in soil samples from Hamirpur district, Himachal Pradesh, India, Radiation Measurements, 36 (2003) 547 – 549. |
[6] | Kaleel Mohammed Thabayneh, Mohanad Mohammed Jazzar, Natural Radioactivity Levels and Estimation of Radiation Exposure in Environmental Soil Samples from Tulkarem Province-Palestine, Open Journal of Soil Science, 2012, 2, 7-16. |
[7] | M. Mehade Hasan, M. I. Ali, D. Paul, M. A. Haydar, S. M. A. Islam, Measurement of Natural Radioactivity in Coal, Soil and Water Samples Collected from Barapukuria Coal Mine in Dinajpur District of Bangladesh, Journal of Nuclear and Particle Physics, USA, vol.3, No. 4, pp. 63-71(2013). |
[8] | C. E. Roessier, Z. A. Smith, W.E. Bloch and R.J Prince, Uranium and radium in Floride phosphate materials, Health Phys. vol. 37, 1970, pp. 269-277. |
[9] | M. A. Usif and A. E. Taher, Radiological assessment of Abu-Tartur phosphate, western desert Egypt, Radiation Protection Dosimetry , vol.130, 2008, pp. 228-235. |
[10] | G. F. Knoll, Radiation Detection and Measurement, Third edn.1998, New York. |
[11] | UNSCEAR, Sources and effects of ionizing radiation, Report of the United Nations Scientific Committee on the Effects of Atomic Radiation to the General Assembly, United Nations, New York, USA, (2000). Annex. |
[12] | K. Debertin and R.G. Helmer.Gammand X-ray spectrometry detectors, North Holland, 1988. |
[13] | Beretka, J. and Mathew, P. J. , Natural radioactivity of Australian buildings, materials, industrial wastes and by products, Health Physic, 48, 87-95 (1985). |
[14] | Lu, X. And Xiolan, Z. (2006): Measurement of natural radioactivity in sand samples collected from the Booje Weithe sand park, China, Environ. Geol. 50, 977-988. |
[15] | J. Beretka and P. J. Mathew, Natural Radioactivity of Australian Building Material, Industrial Waste and By-products, Health Physcs, Vol.48, No.1, 1985, pp.87-95. doi:10.1097/00004032-198501000-00007. |