Frazier Nyasulu, Rebecca Barlag, Lauren McMills, Phyllis Arthasery, Whalmany Ounkham
Department of Chemistry and Biochemistry, Ohio University, Athens, OH, USA
Correspondence to: Frazier Nyasulu, Department of Chemistry and Biochemistry, Ohio University, Athens, OH, USA.
Email: |  |
Copyright © 2018 Scientific & Academic Publishing. All Rights Reserved.
This work is licensed under the Creative Commons Attribution International License (CC BY).
http://creativecommons.org/licenses/by/4.0/

Abstract
In this exercise, we replace traditional water displacement schemes with a simpler, safer, and easier setup based on a combination of a standard and a unitary wash bottle. The goal is to identify two unknown metals whose ions can have charges of +1, +2 or +3. The volume of the H2(g) produced by reaction of the metal with HCl(aq) is determined using the new water displacement setup. The atomic mass is calculated by considering the mass of the metal used, moles of H2(g) calculated from the ideal gas law and the stoichiometry of the reaction. For each metal ion charge, class data are pooled (N >10) and the 95% confidence interval of the atomic mass is calculated. In the event that a number of metals fit, known physical and chemical properties of the metals are used to make a final identification. The percent composition of a mixture of magnesium and manganese is determined from a calibration plot of moles H2(g) per gram of metal versus percent Mg. This determination is possible because magnesium and manganese produce widely differing moles of H2(g) per gram of metal. Students’ results were within ±0.3% of the actual value.
Keywords:
First-Year/General Undergraduate, Stoichiometry, Ideal Gas Law, Water Displacement
Cite this paper: Frazier Nyasulu, Rebecca Barlag, Lauren McMills, Phyllis Arthasery, Whalmany Ounkham, A Simple, Safe, and Easy Water Displacement Exercise for the Identification of Two Metals and the Composition of a Mixture, Journal of Laboratory Chemical Education, Vol. 6 No. 2, 2018, pp. 21-23. doi: 10.5923/j.jlce.20180602.01.
1. Introduction
Water displacement schemes are widely used in general chemistry labs to determine the volume of gas produced by chemical reactions [1-10]. The popularity is due in part to the simplicity of the setup with items ordinarily available in teaching laboratories. When the temperature and pressure are known, the amount of gas can be calculated using the volume of gas generated and the ideal gas law. Water displacement schemes can also be used to determine kinetics of reactions if the volume is measured as a function of time [6, 10].The identification of an unknown substance by profiling its physical and chemical properties is an important part of chemistry. A commonly practiced general chemistry laboratory exercise is identifying an unknown metal by an oxidation reaction with an acid and measuring the volume of the H2(g) produced. In order to identify the metal, the charge on the ion must be provided so that the correct stoichiometry of the reaction can be written. An alternate procedure based on measuring the pressure in a fixed volume system has also been reported [11]. If two metals produce widely differing moles of H2(g) per gram, the difference can be used to determine the percent composition of a mixture of the two metals. Zinc produces 0.0153 moles of H2(g) per gram and aluminum produces 0.0553 moles of H2(g) per gram. This difference has been used to determine the percent composition of a zinc-aluminum alloy [7, 8].We present an improved water displacement laboratory exercise in which:i. wash bottles are used to provide a simpler and safer water displacement scheme.ii. two metals (M1 and M2) with possible ion charges of +1, +2, or +3 are identified from pooled class data and the calculated 95% confidence interval of the atomic mass. Physical and chemical properties of the metals are used to make a final identification.iii. the percent composition of an unknown mixture of M1 and M2 is determined from a calibration curve of moles H2(g) per gram versus % composition of M2.Targeted Learning OutcomesFor each metal, assuming a charge of M+, M2+, and M3+, students will be able to:i. Write overall and net ionic equations for the possible chemical reactions.ii. Measure the volume, pressure, and temperature of the collected H2(g) and use the ideal gas law to calculate moles. iii. Use stoichiometric relationships to calculate moles of M.iv. Calculate the atomic mass of M.v. Calculate the 95% confidence interval of the atomic mass using pooled student data and determine if the interval includes more than one element. vi. Use known properties to identify the metal in cases of ambiguity.vii. Use moles of H2(g) per gram of M1 and moles H2(g) per gram of M2 to plot a calibration curve using class data. viii. Determine the percent composition of a mixture of M1 and M2.
2. Experimental
2.1. Materials, Equipment and Setup
Hazards: 3 M HCl(aq) is corrosive; avoid physical contact.500-mL unitary wash bottles, 250-mL standard wash bottles, 0.9 mL gelatin capsules (Parr Instrument Co.), magnesium powder, manganese powder, 3 M HCl(aq), milligram mass balance, temperature measurement device, pressure measurement device, stir bars and stirrers. A picture of the setup is shown in Figure 1.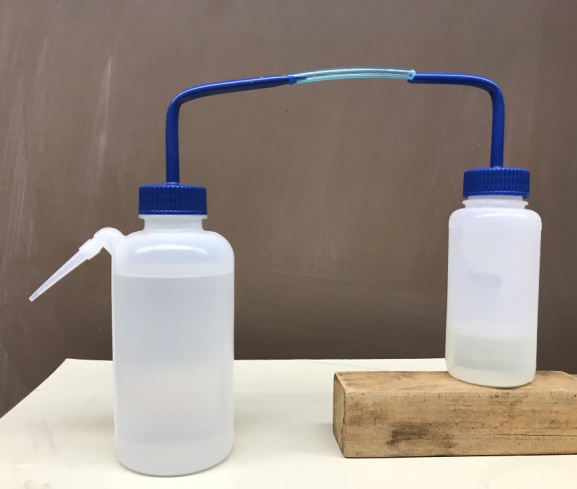 | Figure 1. Water displacement scheme |
2.2. Procedure
Two wash bottles are used and connected to each other as indicated in Figure 1. The 500-mL bottle (unitary wash bottle) is filled with tap water to the mark. The 250-mL standard bottle is the reaction chamber. An empty gelatin capsule is weighed, loaded with metal sample and reweighed. A stir bar, 20 mL of 3 M HCl(aq), and the gelatin capsule are added to the reaction wash bottle and the lid secured. It takes about 3 minutes for the gelatin capsule to dissolve and expose the metal to the acid, which is enough time to secure the wash bottle lid. A beaker is placed to collect the water displaced from the unitary wash bottle. When the water displacement initially stops, the reaction bottle is swirled for one minute to react unreacted metal. This procedure is repeated two times to ensure complete reaction of all the metal. The volume of the water displaced from the wash bottle is measured using a graduated cylinder. The atmospheric pressure is measured; the gas pressure inside the flask is assumed to be equal to the atmospheric pressure. The temperature in the room is measured; it is assumed that the room temperature is equal to the temperature of the H2(g) in the wash bottle.
3. Results and Discussion
3.1. Selecting the Metals to be Analyzed
The metals tested were magnesium, aluminum, zinc, manganese and cadmium. The criteria used in selecting the two metals to be analyzed were: (i) A large difference in the moles of H2(g) produced per gram of metal. The large difference is necessary for the determination of the percent composition of a mixture of the two metals. (ii) Ease with which H2(g) is generated by the metal. (iii) Toxicity of the metal. Magnesium produces 0.0412 moles H2(g) per gram, Al produces 0.0556 moles H2(g) per gram, Zn produces 0.0153 moles H2(g) per gram, Mn produces 0.0182 moles H2(g) per gram, and Cd produces 0.000890 moles H2(g) per gram. Although Al produces the largest number of H2(g) moles per gram, its reaction is slow. Cadmium was eliminated due to toxicity. The metals selected were Mg and Mn.
3.2. Identification of Metals
3.2.1. M1
Sample data associated with the identification of M1 are shown in Table 1. Assuming M1 has a charge of +1, the calculated molar mass is 12.2 ± 0.2 g/mol (N = 11) indicating carbon. Since carbon is not a metal, this does not apply. Assuming M1 has a charge of +2, the molar mass is 24.2 ± 0.2 g/mol (N = 11) indicating magnesium. Since the 95% confidence interval does not include any other element, magnesium is indicated. Assuming M1 has a charge of +3, the molar mass is 36.2 ± 0.2 g/mol (N = 11) suggesting chlorine, which is a nonmetal. Therefore, it can be concluded that M1 is magnesium.Table 1. Sample data, calculations, and results for M1 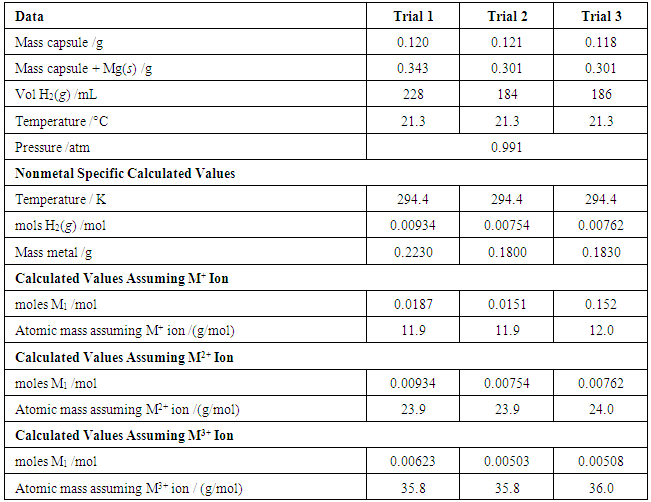 |
| |
|
3.2.2. M2
Similar data and the related calculations performed for M2 afforded results summarized in Table 2. Based on the results, M2 is either Al or Mn. Because Al has a charge of +3 and not +1, Al is eliminated. A second method is to measure the density of M2 and compare it to the known densities of Al (2.70 g/cm3) and Mn (7.20 g/cm3).Table 2. Summary of Results for Identification of M2  |
| |
|
3.2.3. Mixtures
Students use the following equation to determine the two-point calibration plot:
The equation is: % M2 = 0.000239x + 0.0182For a mixture known to consist of 68.3% Mg, students’ results showed this to be 68.1 ± 0.3% Mg (N = 11).
4. Conclusions
The use of two wash bottles, one wash bottle for the reaction and the other for water displacement greatly simplifies data collection compared to other water displacement schemes. The H2(g) is safely contained within the wash bottle prior to release in the fume hood. In all the identification of metal water displacement exercises previously reported, the metal ion charge is provided to the students. We ask students to consider three possible charges, +1, +2 and +3. Based on atomic mass, each one of these may lead to an element that may or may not be a metal meaning that other chemical or physical properties must be considered to make a final determination. When student results are pooled, a sizeable sample (N > 10) is achieved allowing for meaningful statistical analysis to be performed. This lab uses an application of the 95% confidence interval that is easily understood by students.
References
[1] | Roberts, J. L., Hollenberg, J. L., Postma, J. M., General Chemistry in the Laboratory, Freeman, 1984, pp 93-102. |
[2] | Szafran, Z., Pike, R. N., Foster J. C., Microscale General Chemistry Laboratory with Selected Macroscale Experiments, John Wiley, New York, 1993, pp 143-154. |
[3] | Nelson, J. H., Kemp, K. C., Laboratory Experiments, Chemistry the Central Science, Prentice Hall, 8th ed., Upper Saddle River, NJ, pp 149-159. |
[4] | Munov, S. L., Experiments in General Chemistry, 4th ed. Thomson Brooks Cole, 2004, pp 209-224. |
[5] | Weiss, G.S., Greco, T.G., Rickard, L.H., Experiments in General Chemistry, Principles and Modern Applications, 7th ed., Prentice Hall, 1997, pp 91-96. |
[6] | Teggins, J., Mahaffy, Kinetics studies in a wash bottle, C. J. Chem. Educ., 1997, 74, 566. |
[7] | Masterton, W. L., Analysis of an aluminum-zinc alloy: A general chemistry laboratory, J. Chem. Educ., 1961, 38, 558. |
[8] | Slowinski, E. J., Chemical Principles in the Laboratory, Brooks/Cole, 9th ed., pp 61-68. |
[9] | Nyasulu, F., Paris, S., Barlag, R., Wash bottle laboratory exercises: Mass of NaHCO3 in an Alka-Seltzer tablet, molar mass of CO2 and the ideal gas constant, J. Chem. Educ., 2009, 86, 842. |
[10] | Barlag, R., Nyasulu, F., Wash bottle laboratory exercises: iodide-catalyzed H2O2 decomposition reaction kinetics using the initial rate approach, J. Chem. Educ., 2010, 87, 78-80. |
[11] | Gordon, J., Boyce, A., Maule, T., The microscale determination of the molar mass of metals using a gas-pressure sensor, The Chemical Educator, 2004, 9, 364-365. |