Todd Wimpfheimer
Department of Chemistry and Physics, Salem State University, Salem MA, USA
Correspondence to: Todd Wimpfheimer, Department of Chemistry and Physics, Salem State University, Salem MA, USA.
Email: |  |
Copyright © 2015 Scientific & Academic Publishing. All Rights Reserved.
Abstract
Particle in a one dimensional box laboratory experiments have traditionally used chemicals like polyenes or cyanine dyes as model systems. This paper describes an experiment in which beta-carotene and lutein, compounds that are present in carrots and spinach respectively, are used to model the particle in a one dimensional box system. This approach provides a useful, safe, and inexpensive physical chemistry experiment that exposes students to the chemistry of real world substances.
Keywords:
Physical chemistry, Quantum chemistry, Upper division undergraduate, Laboratory experiment
Cite this paper: Todd Wimpfheimer, A Particle in a Box Laboratory Experiment Using Everyday Compounds, Journal of Laboratory Chemical Education, Vol. 3 No. 2, 2015, pp. 19-21. doi: 10.5923/j.jlce.20150302.01.
1. Introduction
Laboratory experiments concerning the particle in a one dimensional box have long been a staple in the undergraduate physical chemistry laboratory. Traditionally, chemicals like cyanine dyes [1, 2] have been used to model the one dimensional box system. More recently, polyenes [3, 4], and polyynes [5, 6] have been used. All of these systems contain long conjugated systems that can be approximated at some level to being linear and therefore can be modelled using the quantum mechanical particle in a one dimensional box. Theoretically, other systems that have long, conjugated, nearly linear chains could also be used. Also of interest would be to use chemicals with which students may be more familiar and that they see in their daily life. The chemical systems proposed in this work are beta-carotene and lutein, compounds found in common vegetables like carrots and spinach.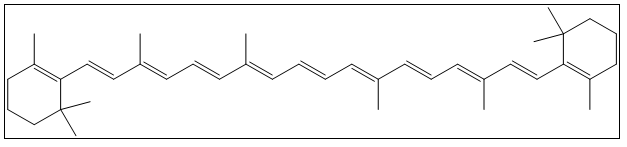 | Figure 1. Structure of beta-carotene |
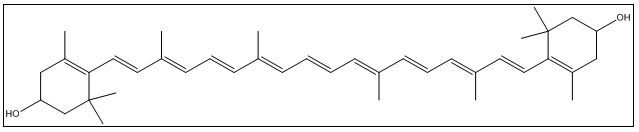 | Figure 2. Structure of lutein |
When used in particle in a box experiments, polyenes and cyanine dyes are often dissolved in solvents such as cyclohexane. Some of these compounds take a while to sufficiently dissolve in the solvent. The solutes proposed for this work are soluble enough in just a few minutes to make this experiment quick, safe, and even possible to do without a fume hood.
2. Experimental
To begin, a speck of beta-carotene is dissolved in approximately 4 mL of ethanol. After a light color develops, the absorption spectrum is obtained from 400 nm – 700 nm. In our lab we use Spec-200 spectrophotometers as we have enough instruments for pairs of students to work with their own. The students record the wavelength that corresponds to the lowest energy transition in the conjugated system (i.e. the peak having the highest wavelength). If their absorbance is too high, dilution is necessary. A few small pieces are shaved off the exterior of a carrot and likewise dissolved in ethanol until a light color develops. Light grinding with a stirring rod will aid dissolution. Using the spectrum of the pure beta-carotene as a guide, the appropriate wavelength of the beta-carotene is then determined. Next, a pure lutein gel capsule is punctured with a pin and a drop is dissolved in approximately 4 mL of ethanol until a light color develops. The wavelength that corresponds to the lowest energy transition is then recorded. A couple of small pieces of fresh spinach are then dissolved in ethanol until a light color develops. Using the spectrum of pure lutein as a guide, the appropriate wavelength of the lutein in spinach is determined. The instructor can, if desired, discuss the spectral peak for the chlorophyll in spinach which occurs above 600 nm. Example experimental spectra are given in Figures 3 and 4.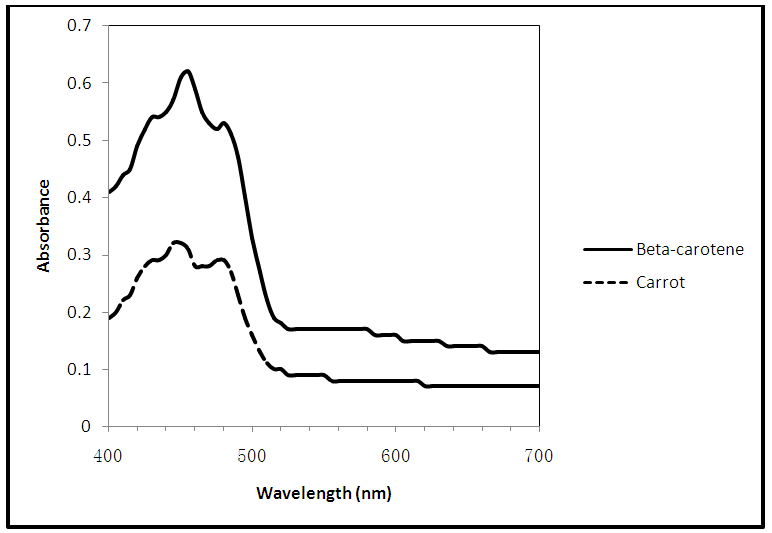 | Figure 3. Visible absorption spectra of beta-carotene and carrot |
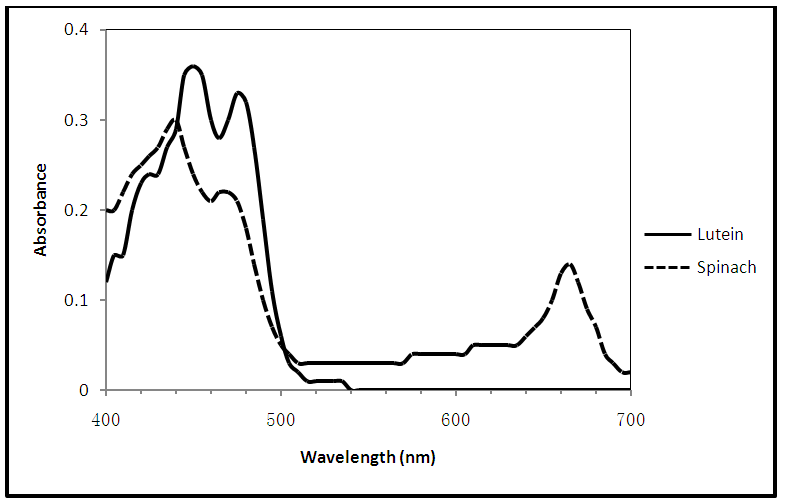 | Figure 4. Visible absorption spectra of lutein and spinach |
Students then use the wavelength (λ) obtained from each spectra to calculate the length of the one dimensional box. The formula for the energy of a particle in a one dimensional box is given by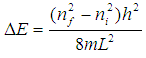 | (1) |
where L is the length of the box, m is the mass of the electron, h is Planck’s constant, and the n’s are the initial and final quantum levels for the transition. The lowest energy transition is between the highest occupied molecular orbital (HOMO) and the lowest unoccupied molecular orbital (LUMO). Each molecular orbital holds two electrons. If N is the number of electrons in the conjugated π system, then the number of occupied molecular orbitals is N/2. The energy difference is then: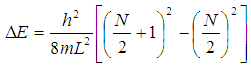 | (2) |
If the wavelength associated with the lowest energy transition is measured, the length of the box can be calculated as follows: | (3) |
Setting equations (2) and (3) equal and solving for L2 yields: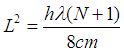 | (4) |
3. Hazards
Ethanol is flammable. Beta-carotene, lutein, spinach, and carrot pose no significant health hazard; in fact, they are part of a healthy diet.
4. Discussion
This experiment is simple for the students to run and uses substances that are less hazardous than those typically employed in a particle in a box experiment. By experimenting with food like carrots and spinach, students also may see a connection to chemistry in everyday life. On a tight budget, the instructor could omit the pure beta-carotene and lutein and run the experiment with just carrots and spinach. The lengths of the one dimensional boxes obtained using the experimentally determined wavelengths are given in Table 1. These lengths may be compared to a box length constructed using typical carbon-carbon single and double bond lengths of 1.53 Å and 1.34 Å respectively. Those box lengths are also given in Table 1 for comparison. Table 1. Comparison of Box Lengths Obtained From Experimentally Determined Wavelength Versus Those Determined Using Typical Bond Lengths |
| |
|
However, in conjugated chains such as the ones in this experiment, the single bond is usually shorter than the typical length. Students are asked to perform a molecular modeling calculation using Spartan or a similar program. An optimization of the beta-carotene structure using Spartan yields a box length of 23.1 Å. The students are then asked to discuss why there is a difference between the experimentally determined value of the box length and the value obtained from modeling. Furthermore, the instructor can discuss modeling a linear box length assuming an all-trans zigzag carbon chain with C-C-C bond angles of 120° and employing the Law of Cosines. The instructor can also discuss whether the box ends at the six-membered rings or whether it is appropriate to allow for the box to extend into the rings. The experimental results show qualitative agreement with theory. This is acceptable considering the rudimentary approximation of modeling a complex, three dimensional system as a one dimensional box. This experiment is appropriate for the instructor who is seeking a simple experiment to expose students to a quantum mechanical system using accessible compounds. More accurate results may be obtained by using cyanine dyes or utilizing first-order perturbation theory [7]. The experiment described here is easily run during a typical laboratory period. Our typical lab size is 12-14 students working in pairs.
References
[1] | D. P. Shoemaker, C. W. Garland, and J. W. Nibbler, Experiments in Physical Chemistry, 8th ed., New York; McGraw-Hill, 2009, pp 393-397. |
[2] | R. J. Sime, Physical Chemistry Methods, Techniques, and Experiments, Philadelphia: Saunders College, 1990, pp 687-694. |
[3] | Soltzberg, L. J., 2001, Electronic spectra of conjugated systems: a modern update for a classic experiment, J. Chem Educ., (78), 1432. |
[4] | Anderson, B. D., 1997, Alternative compounds for the particle in a box experiment, J. Chem. Educ., (74), 985. |
[5] | Henry, M, Roth H., and Anderson, B. D., 2012, The particle in a box redux: improved experimental conditions for the laser synthesis of polyynes, J. Chem. Educ., (89), 960-961. |
[6] | Anderson, B. D. and Gordon, C. M., 2008, The laser synthesis of linear polyynes, J. Chem. Educ., (85), 1279-1281. |
[7] | Autschbach, J., 2007, Why the particle in a box model works well for cyanine dyes but not for conjugated polyenes, J. Chem. Educ., (84) 1840-1845. |