Omar A. El Seoud 1, Paulo A. R. Pires 1, Edgar O. Moraes 2, Jairo J. Pedrotti 2, Haq Nawaz 1
1Institute of Chemistry, the University of São Paulo, São Paulo, SP
2Department of Chemistry, Mackenzie Presbyterian University, São Paulo, SP
Correspondence to: Omar A. El Seoud , Institute of Chemistry, the University of São Paulo, São Paulo, SP.
Email: |  |
Copyright © 2015 Scientific & Academic Publishing. All Rights Reserved.
Abstract
We present an interdisciplinary project (physical- and organic chemistry/spectroscopy) on the kinetics of the pH-independent hydrolysis of bis(2,4-dinitrophenyl) carbonate in mixtures of water and acetonitrile or dimethoxyethane. In order to increase the students’ interest in the project, we used the following constructivist teaching approach: we stated the problem (studying the kinetics of ester hydrolysis), and then challenged the students to define an experimental protocol. The students did the experiment and presented their data, namely the dependence of rate constants and activation parameters on water concentration and temperature. We then linked theory to experiment by discussing the mechanistic use of isosbestic points and activation parameters; the determination of the partial kinetic orders with respect to the reactants (ester and water), and emphasized the question of obtaining quality kinetics data. We suggest this project for science students in general, because the experiment is safe, low-cost, and can be used to teach theory.
Keywords:
Active learning, Constructivist teaching, Ester hydrolysis, Reaction mechanism, Quality kinetics
Cite this paper: Omar A. El Seoud , Paulo A. R. Pires , Edgar O. Moraes , Jairo J. Pedrotti , Haq Nawaz , An Undergraduate Project “For all Seasons”: The pH-Independent Hydrolysis of bis(2,4-dinitrophenyl) Carbonate in Aqueous Solvents, Journal of Laboratory Chemical Education, Vol. 3 No. 1, 2015, pp. 1-6. doi: 10.5923/j.jlce.20150301.01.
1. Introduction
Acyl-transfer reactions, in particular, ester hydrolysis and trans-esterification are of central importance in chemistry [1], biochemistry [2], and more recently in biofuel production [3]. Determination of the rate constants of base-catalyzed hydrolysis of carboxylic acid esters, in particular ethyl acetate, is a widely used experiment in the undergraduate physical chemistry laboratory [4]. This experiment is carried out with different degrees of sophistication, e.g., back titration of base employing an acid-base indicator, or by measuring solution conductivity [5]. Another variety is to study this reaction under acid-, general-base, or nucleophilic catalysis [6].By doing any of these experiments, the student gains what is expected from a “conventional” experiment: He (or she) learns practical skills (sample preparation; equipment manipulation, data acquisition) and gains experience in processing, and discussing his data; not enough! We used the constructivist approach and a versatile kinetic experiment to get the students more interested in the practical course, and to link theory to experiment [7]. We achieved this by introducing an interdisciplinary project (physical and organic chemistry/spectroscopy) on the kinetics of pH-independent hydrolysis of bis(2,4-dinitrophenyl) carbonate (DNPC) in aqueous solvents [8].Doing chemical kinetics can be deceptively simple because the student usually calculates a number (rate constant), whether the experiment was done properly or not. This is because most equipment employed for studying chemical kinetics (spectrophotometers, conductivity meters, etc.) are connected to computers for data acquisition; software is available for subsequent calculations. The problem, if it does occur, appears later when data from different groups are employed, e.g., in order to calculate the activation parameters. For example, plots of ln kobs, (observed rate constants) or ln k2 (second order rate constants) versus 1/T (temperature) may show obvious scatter. When this occurs, the students feel frustrated, justifiably so, because there may be no time left to find out what went wrong, or to repeat the experiment in order to get consistent data. This situation contrasts with the organic chemistry laboratory where the students assess the success of their synthesis by comparing the properties of the products obtained (m.p., IR or NMR spectra) with the corresponding literature data.With this background in mind, we have introduced the pH-independent hydrolysis of DNPC in binary mixtures of water and organic solvents, vide infra. We used the project for teaching both theory (reaction mechanism; use of isosbestic points and activation parameters; solvent effects) and practical kinetics (obtaining quality rate data). The students appreciated our use of the constructivist approach, as well as their participation in decision making regarding the experimental protocol.
2. Experimental
Solvents, reagents, and stock solution preparationWe purchased the chemicals from Alfa-Aeser and Merck. The staff purified acetonitrile, MeCN and dimethoxyethane, DME by distillation from CaH2. DNPC was available from a previous study [8]; it can be synthesized by nitration of diphenyl carbonate by HNO3/H2SO4 mixture [9]. The staff purified DNPC by sublimation under reduced pressure.Stock solution preparationThe staff prepared (0.005 mol L-1) DNPC stock solutions in dry MeCN or DME. The students prepared all aqueous solvent mixtures in a fume hood. These “solvents” contained 0.005 mol L-1 HCl, and were prepared by volume by using precision burettes, e.g., Kimble No. 17021H 25, or adjustable volume pipette, e.g., Fisher FBE-05000. Mixtures of water and the organic solvents employed are not ideal, i.e., the final volume is not the sum of volumes of the two components [10]. Therefore the students weighted 10 mL empty volumetric flasks (Schott No. 2167808); added 0.1 mL of 0.5 mol L-1 HCl solution; completed the rest of water to achieve the required volume; weighted the flasks again, and then completed the volume to the mark with the organic solvent at 25℃. Kinetic runsKinetic runs were performed with CPU-controlled single-beam Zeiss PM6KS- UV-Vis spectrophotometers, provided with cell holders whose temperature were controlled by water circulation from thermostats. The students initiated the runs by injecting 20 μL of the ester stock solution (in MeCN or DME) into 2 mL of the acidified binary solvent mixture. They used hand-held, battery-operated micro-stirrer (model 338.004, Hellma) to homogenize the reaction mixture, after which they followed the increase in solution absorbance (At) as a function of time (t) at wavelength, λ = 302 nm (due to liberation of 2,4-dinitrophenol) until the absorbance was practically constant (“infinity” reading, A∞). From the absorbance (At) and the corresponding time (t) the students first calculated (A∞) with a non-linear regression analysis program [11]; theoretically calculated and experimental (A∞) agreed within 3%. Next they calculated the value of kobs, in s-1, from the slope of ln(A∞ - At) versus (t) plot, and k2, in L mol-1 s-1, by dividing kobs by [H2O]. The activation parameters were calculated at T = 25℃, based on the values of k2, by using the following standard equations [12]: | (1) |
 | (2) |
 | (3) |
In Eqn. 2, T is in K; the time unit of k is s-1;
is in cal mol-1.Determination of the best conditions for carrying out the experiment, i.e., the experimental protocol was carried out as off-class activity with help of the instructor, using Shimadzu UV-2500 double-beam spectrophotometer.
3. Results and Discussion
Active learning makes the students interested and is a stimulating approach to learn theory from experimentThe pharmacy students that did this project (a total of 56; divided in 8 equal-number groups) had previously two semesters of organic chemistry and one semester each of general- and physical chemistry. The students did the kinetics of DNPC hydrolysis as an application of UV-V is spectroscopy. Rather than giving them a “recipe”, we used the constructivist approach to involve them in planning the experimental protocol, and learn aspects of theory from experiment (reaction mechanism; use of isosbestic points, kinetic orders, and activation parameters). We ran the project in steps, by employing the following sequence of activities:1- Read theoryTwo weeks before going to the laboratory we asked them to review what they studied before about themes related to the experiment: mechanisms of acyl transfers and chemical kinetics. 2- Plan and carry out the experimentOne week before doing the kinetics, we explained the general idea: use UV-Vis spectrophotometry to study the hydrolysis of DNPC in an aqueous solvent at different temperatures. We handed out a quiz, where we asked them: (i) Write down the steps of hydrolysis of DNPC; (ii) Choose a solvent from the following list, based on miscibility with water, b.p. and toxicity: acetone, MeCN, DME, methanol, ethanol, and 2-propanol. (iii) Elaborate a protocol for the experiment (λ to follow reaction progress; water concentration range). The students worked in eight groups (7 each) to solve these questions. With regard to (i), most were able to write the correct reaction mechanism shown in Scheme 1, but did not have information to indicate its rate-limiting step. As will be shown below in Figure 2, the experiment that they did answered this question.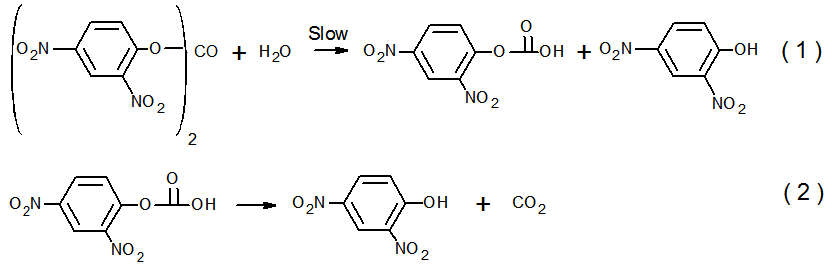 | Scheme 1. Mechanism of the hydrolysis of DNPC. The slow step involves water attack on the ester acyl group, followed by fast decomposition of the mono-ester of carbonic acid [8] |
The students avoided alcohols (point ii) because their use leads to two parallel reactions, hydrolysis and formation of dialkylcarbonate, respectively. Instead, they chose MeCN and DME. We explained that this reaction is independent of acid concentration in the range 0.005 to 0.05 mol L-1, corresponding to (nominal) pH 2.3 to 1.3, and that the kinetics will be carried out under acid condition (0.005 mol L-1 HCl) in order to suppress any catalysis by traces of base [8]. This part of the activities took two hours.Part (iii) required that they use a recording spectrophotometer and dedicate some time in order to find out the best conditions for the reaction. Therefore, they carried out this part in off-class hours, with help of the instructor.Figure 1 shows the spectra recorded for authentic DNPC in dry MeCN, and the corresponding reaction product under acid- (2,4-dintrophenol; 0.005 mol L1 HCl) and alkaline condition (sodium 2,4-dinitrophenolate; 0.001 mol L1 NaOH;). From this experiment, they decided to use λ = 302 nm, and follow the formation of 2,4-dinitrophenol. 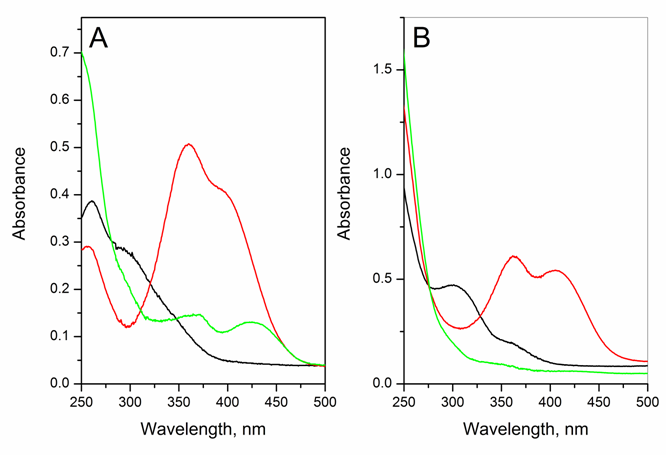 | Figure 1. UV-Vis spectra of 5x10-5 mol L-1 DNPC solution in pure organic solvent (green curves) and the corresponding hydrolysis product under acidic- (2,4-dinitrophenol, black curve), and basic condition (sodium 2,4-dinitrophenolate, red curve), in 30 mol L-1 water in MeCN, part (A) and DME, part (B); 1 cm path cell, 25℃ |
Figure 2 shows repetitive scans of the reaction as a function of time at [water] = 30 mol L-1. From this result, and from literature data on the reaction in MeCN [8], they considered this water concentration as an upper limit.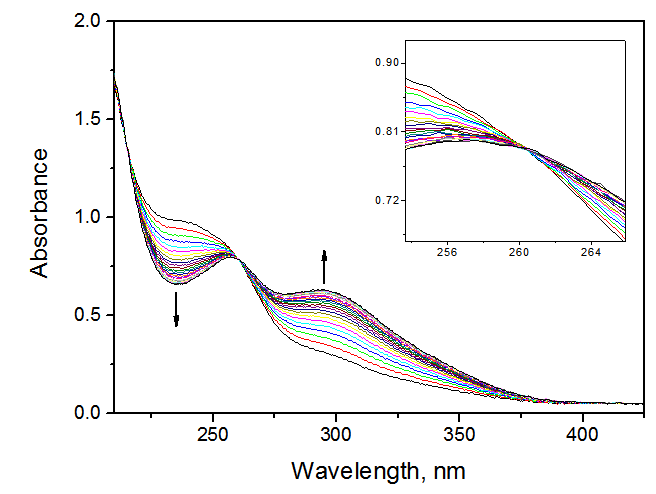 | Figure 2. Kinetics of the hydrolysis of DNPC in 30 mol L-1 water in MeCN, 0.005 mol L1 HCl. The insert shows the isosbestic point at 260.3 nm. The arrows show the direction of the absorbance variation. The time interval between successive scans is 60 s |
Having decided on the experimental protocol, in terms of λ and [water] they went to the laboratory and carried out the experiment. Four groups studied the effects on kobs of changing [water] at 25℃, whereas the other four groups studied the effect of changing T at fixed [water] = 10 mol L-1. The results obtained are shown in Tables 1 and 2, respectively. This part of activities took eight hours (two four-hour periods), including the time required for demonstration by the instructor (how the spectrophotometer works; the software commands for data acquisition). Table 1. Effect of [H2O] on kobs for the hydrolysis of DNPC in MeCN-W and DME-W, at 25 ℃.a 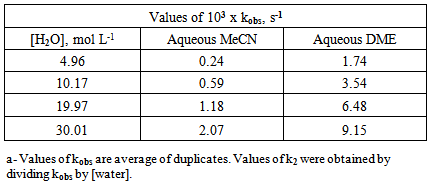 |
| |
|
Table 2. Effect of T on kobs and activation parameters for the hydrolysis of DNPC in 10 mol L-1 of water in MeCN and DME.a 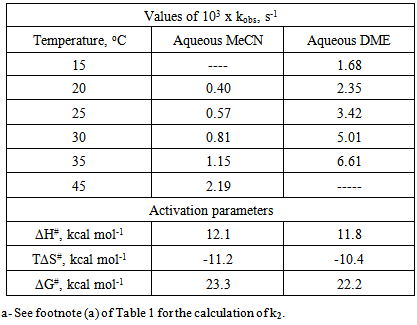 |
| |
|
Conclusion of the activities: Learning theory from experimentThe students groups exchanged their data, carried out the calculations, presented and discussed the following effects on rate constants: water concentration; temperature, and nature of the organic solvent. This was followed by discussion, oriented by us, of the data obtained so that they learn the following aspects of theory:- Mechanistic use of the isosbestic pointFigure 2 shows a clear isosbestic point at 260.3 nm. That is the reaction intermediate, the mono-ester of carbonic acid (Scheme 1), does not accumulate. Consequently, its formation is rate-limiting [8, 12, 13].- Obtaining quality kinetic data and determination of the partial kinetic orders with respect to the reactantsWe discussed several aspects of Figure 3, based on their results (parts I and II) as well as one experiment carried out by us under faulty conditions (III) in order to show a potential problem in chemical kinetics (no proper T control). Because they carried out the reaction under pseudo-first order conditions (water in large excess), then the linear dependence of ln(A∞-At) on (t) means that the reaction is first order in ester, as shown in part (II) of Figure 3. From Table 1, plots of log(kobs) versus log [water] gave straight lines, whose slopes correspond to the kinetic order with respect to water, (nWater); practically unity for both binary solvent mixtures. Unlike several “classic” physical-chemistry experiments, e.g. mutarotation of glucose and inversion of sucrose, where water is the solvent, the present experiment permits variation of [water], hence calculation of (nWater). We explained that the meaning of this order is more complex, because the properties of the binary solvent mixture change massively as a function of [water] [14], so that the value of (nWater) should be employed with reserve [8]. An important aspect of Figure 3 is the residues plot (Aexperimental – Acalculated). The quality of the experiment can be judged by the magnitude (small) and variation (randomness) of these plots. Part (III) of Figure 3 shows how a bad experiment looks like. We explained that this experiment was done, on purpose, by using a faulty procedure. Namely, we started the experiment without proper T control. As is clear, the ln(A∞-At) versus (t) plot is not linear; the residues plot is not random, and the limits of errors are large. 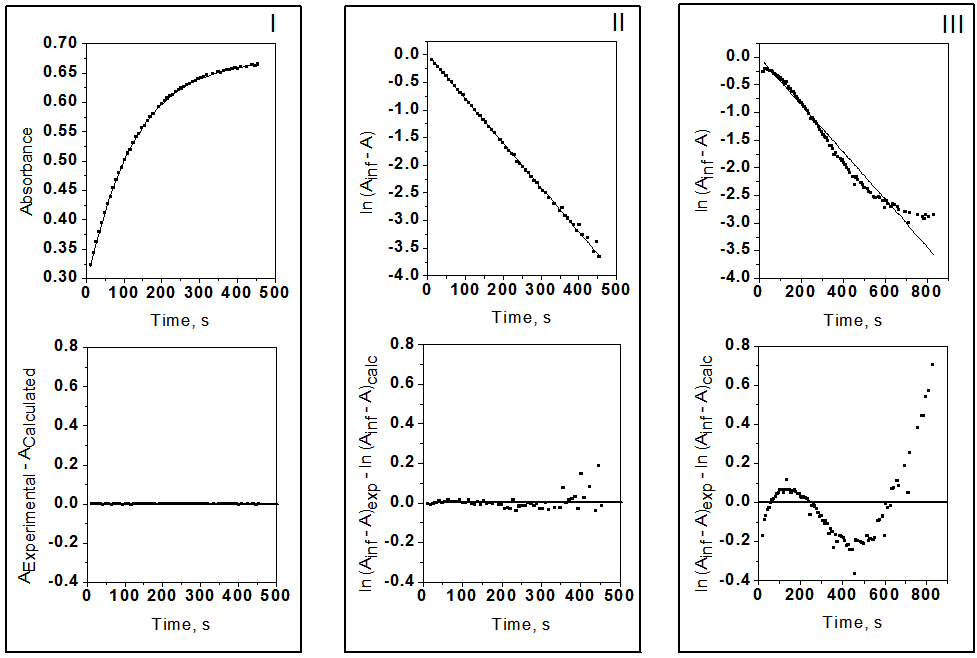 | Figure 3. Upper part: Absorbance versus t (part I), ln(A∞-At) versus t (part II), for the reaction in aqueous acetonitrile, and ln(A∞-At) versus t with no temperature control (part III). Conditions: λ = 302 nm; [water] = 10 mol L-1; T = 45℃. The corresponding residues plots are shown in the lower part |
Effect of the mediumThe faster reaction in water-DME needed an explanation. Their initial suggestion, acid catalysis by protonated DME, was rejected after they found out that the pKa of protonated aliphatic ethers is very low, ca. -3.5, [15] i.e., the solvent will not be protonated under the experimental conditions. Although MeCN is more polar than DME, the latter is much more basic, as indicated by solvatochromic dyes [14]. Based on this basicity, the propensity of DME to form hydrogen bonding with water is higher than that of MeCN [16]. We suggested that the rate enhancement is because DME is acting as a general-base for the water molecule that attacks the ester, as depicted in Figure 4.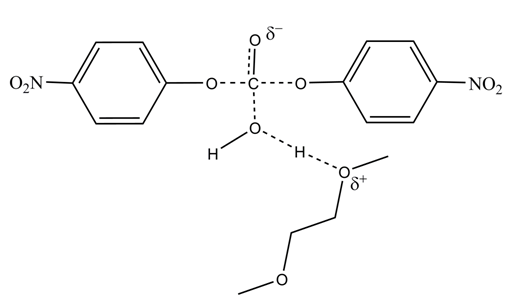 | Figure 4. Suggested transition-state structure for the reaction of hydrolysis of DNPC in the presence of DME [8, 16] |
Their kinetic results, Table 2, corroborate this suggestion. Thus the reaction in aqueous DME is faster than that in aqueous MeCN due to favorable
(0.3 kcal mol-1), resulting from activation of the attacking water molecule by hydrogen bonding, and favorable
(0.8 kcal mol-1) due to more structured reagent state. This last part of the project required two hours. At the end of this period, we handed out an evaluation sheet that they returned a week later.
4. Conclusions
The student-focused constructivist approach turns the laboratory interesting because the students are actively involved in all stages of the experiment. Rather than using standard teaching, we defined the problem, asked them for a solution and exploited the presentation of their results in order to teach theory. Although the project took 12 hours to be concluded, their evaluation sheets indicated that they enjoyed the constructivist approach and working in groups. They were satisfied with the agreement between their results and literature data (aqueous MeCN) [8], especially because the latter research was done in their own Institute. Finally, they appreciated the link between this experiment and the mechanism of organic reactions. Hydrolysis of DNPC is a convenient experiment because it requires little preparation; no buffers, and generates very little waste. Additionally, the value of the rate constant can be adjusted simply by changing [water], hence data acquisition can be done manually, if required. The time required for this project can be reduced without decreasing its didactic value, e.g., by studying only one experimental variable (T or [water]), in one binary solvent mixture. Therefore, we recommend it for any science undergraduates (chemistry, biology, engineering, etc.) instead of reliable, but otherwise uninspiring experiments, e.g., the hydrolysis of ethyl acetate and sugar reactions (mutarotation of glucose and inversion of sucrose) [4, 5, 17].
ACKNOWLEDGEMENTS
We thank the students of Chem-0343 for their participation and enthusiasm; the state of São Paulo (FAPESP) for financial support. H. Nawaz and O. A. El Seoud thank the National Council for Scientific and Technological Research (CNPq) for fellowship and research productivity fellowship, respectively.
References
[1] | Vollhardt, P.; Schore, N. Organic Chemistry, 6. ed. New York: W. H. Freeman, 2009. |
[2] | Nelson, D. L.; Cox, M. M. Lehninger Principles of Biochemistry, 3. ed. New York: W. H. Freeman, 2004. |
[3] | Hansen, A. C.; Zhang, Q.; Lyne, P. W. L. Ethanol–diesel fuel blends- a review. Bioresource Tech., 2005, 96, 277-285. |
[4] | Levitt, B.P. Ed. Findlay's Practical Physical Chemistry, 9th ed.; Longman: London, 1973, p 338-341; Sime, R.D. Physical Chemistry: Methods, Techniques and Experiments. Saunders: Philadelphia, 1990, 609-620. |
[5] | Das, K.; Sahoo, P.; Baba, M. S.; Murali, N.; Swaminathan. P. Kinetic studies on saponification of ethyl acetate using an innovative conductivity-monitoring instrument with a pulsating sensor. Intl. J. Chem. Kin. 2011, 43, 648-656. |
[6] | Lombardo, A. Kinetics of imidazole catalyzed ester hydrolysis: Use of buffer dilutions to determine spontaneous rate, catalyzed rate, and reaction order. J. Chem. Educ., 1982, 59, 887-889; Neuvonen. H. Kinetics and mechanisms of reactions of pyridines and imidazoles with phenyl Acetates and trifluoroacetates in aqueous acetonitrile with low content of water: Nucleophilic and general base catalysis in ester hydrolysis. J. Chem. Soc. Perkin Trans. II, 1987, 159-167. |
[7] | Boud, D. J.; Dunn, J.; Kennedy, T.; Thorley, R. The aims of science laboratory courses: A survey of students, graduates and practicing scientists. 1980, Int. J. Sci. Educ., 2, 415-428; Eilks, I.; Byers, B. The need for innovative methods of teaching and learning chemistry in higher education- reflections from a project of the European Chemistry Thematic Network. Chem. Educ. Res. Pract., 2010, 11, 233-240. |
[8] | El Seoud, O. A.; El Seoud, M. I.; Farah, J. P. S. Kinetics of the pH-independent hydrolysis of bis(2,4-dinitrophenyl) carbonate in acetonitrile-water mixtures: Effects of the structure of the solvent. J. Org. Chem. 1997, 62, 5928-5933. |
[9] | Hamilton, N. M. Bis(2,4-dinitrophenyl) carbonate .e-EROS Encyclopedia of Reagents for Organic Synthesis, 2001. Available on-line in:http://onlinelibrary.wiley.com/doi/10.1002/047084289X.rb152/abstract?deniedAccessCustomisedMessage=&userIsAuthenticated=false. Accessed in 22/12/2014. |
[10] | Zarei, H. A.; Lavasani, M. Z.; Iloukhani H. Densities and volumetric properties of binary and ternary liquid mixtures of water (1) + acetonitrile (2) + dimethyl sulfoxide (3) at temperatures from (293.15 to 333.15) K and at ambient pressure (81.5 kPa). J. Chem. Eng. Data 2008, 53, 578–585; Marchetti, L.P.; Tassi, L.; Ulrici, A.; Vaccari, G.; Zanardi, C. Density and volume properties of the 2-methoxyethanol + 1,2-dimethoxyethane + water ternary solvent system at various temperatures. 2001, Phys. Chem. Liq. Internt. J., 39, 151-168. |
[11] | Press, W.H.; Teukolsky, S. A.; Vetterling, W. T.; Flannergy, B. P. Numerical recipes: The art of scientific computing (3rd ed). Cambridge University Press, Cambridge. 2007. |
[12] | Anslyn, E. V.; Dougherty, D. A. Modern physical organic chemistry. University Science Books, Sausalito, 2006, 382. |
[13] | Isaacs, N. S. Reactive intermediate in organic chemistry. Wiley, New York, 1974, p 23. |
[14] | C. Reichardt, T. Welton, Solvents and Solvent Effects in Organic Chemistry, 4. ed. Weinheim, German. Wiley-VCH, 2011; El Seoud, O. A.; Siviero, F. Kinetics of the pH-independent hydrolyses of 4-nitrophenyl chloroformate and 4-nitrophenyl heptafluorobutyrate in water-acetonitrile mixtures: consequences of solvent composition and ester hydrophobicity. J. Phys. Org. Chem. 2006, 19, 793-802. |
[15] | Smith, M. B. March´s Advanced Organic Chemistry, Wiley-Interscience, New York, 2001, Chapter 8, p. 327. |
[16] | Vaidyanathan, G.; Garvey J. F. Mass spectrometric investigation of dimethoxyethane clusters: complete solvation of a protonated cation. 1994, J. Phys. Chem., 98, 2248-2253. |
[17] | Perles, C. E.; Volpe, P. L. O. A Simple laboratory experiment To determine the kinetics of mutarotation of D-glucose using a blood glucose meter, 2008, J. Chem. Educ., 85, 686-688; Leininger, P. M.; Kilpatrick, M. The Inversion of Sucrose, 1938, J. Am. Chem. Soc., 60, 2891–2899. |