Jacob Carter1, 2, Rebecca Steiger3
1Department of Ecology and Evolutionary Biology, University of Kansas, Lawrence, KS, 66044, USA
2Work undertaken by Jacob Carter was completed while at Kansas State University, Manhattan, KS, 66506, USA
3Science Department, Junction City High School, Junction City, KS, 66441, USA
Correspondence to: Jacob Carter, Department of Ecology and Evolutionary Biology, University of Kansas, Lawrence, KS, 66044, USA.
Email: |  |
Copyright © 2014 Scientific & Academic Publishing. All Rights Reserved.
Abstract
Crime scene investigation scenarios have become a popular tool used to teach scientific concepts across a breadth of disciplines. Here we describe a lesson for high school students that uses a crime scene scenario toconvey the complex concept of isotopic fractionation of Carbon-13 across two photosynthetic pathways (C3 vs. C4), and how isotopic data are used by scientists today to study real world problems. This interdisciplinary activity links concepts in chemistry and biology. In addition, this activity demonstrates the importance of programs that foster collaborations between teachers and scientists.
Keywords:
Inquiry, Interdisciplinary, Isotopes, Mass spectrometry, Photosynthesis
Cite this paper: Jacob Carter, Rebecca Steiger, Crime Scene Inquiry Activity: Using a Modern Research Technique to Teach about Photosynthesis and Isotopes, Journal of Laboratory Chemical Education, Vol. 2 No. 2, 2014, pp. 18-24. doi: 10.5923/j.jlce.20140202.03.
1. Introduction
1.1. Overview & Goals
Here we present an inquiry-based criminal scene investigation lab activity for advanced high school classes in chemistry or biology. We had three goals for student learning outcomes: 1) to advance student knowledge and understanding of how isotopic data are used in plant science through inquiry learning, 2) to enhance student learning of how current scientific techniques are used, and 3) to have students critically think about the applicability of using isotopic techniques to address real world problems. This activity addresses many disciplinary core ideas of the Next Generation Teaching Standards and the National Science Education Standards as well as standards for inquiry learning(explicitly described in section 3.2) [1-2]. Students should understand the following scientific concepts as prerequisites before the lab is conducted: isotope chemistry, enzymes, photosynthesis, cellular respiration, and differences between C3 and C4 photosynthetic pathways. The following materials are needed for the activity: 2.5” X 4.25” coin envelopes (how many depends on how much data your classes collect), plastic sandwich bags, canned black beans, different types of lettuces, corn leaves, canned salsa, food coloring (optional), and forceps. Unless using hypothetical data, teachers will need to send samples to a stable isotope mass spectrometry laboratory. We describe partnering with university scientists or working with mass spectrometry labs at universities below. The activity will take approximately 2 hours. We include both teacher and student procedures below as well as conceptual information. Furthermore, for teaching a full module on the use of stable isotopes in plant science, we have included two original supplementary lessons that we used before instruction of the activity presented here.
1.2. Partnering with University Scientists
Opportunities to expose students to current methods in scientific research and their applications can be exciting to students and can increase both interest and efficacy, so that students can better picture themselves “doing” science [3-5]. Some programs have involved scientists in partnerships with classroom teachers to develop educational materials. One program of the U.S. National Science Foundation (NSF), the Graduate STEM Fellows in K-12 Education (GK-12) graduate training program [6], has explicitly used partnerships between universities and public schools to bring topics in scientific research to secondary school students. Through GK-12, graduate students in science, technology, engineering and mathematics (STEM) fields improve their science communication skills by presenting aspects of their research content, in addition to modeling the process of scientific inquiry. Many other training programs as well as individual investigator grants may have outreach projects in public schools as part of the “Broader Impacts” required by NSF. Where such funded partnerships are not available, scientists and teachers can interact by building upon resources created through such projects; and teachers can even interact with university personnel at a distance. The activity presented here resulted from a year-long partnership as part of a GK-12 program at Kansas State University. As a graduate student studying the physiology and ecology of invasive plant species using isotopic evidence [7-8], JC was partnered with high school chemistry and biology teacher RS, and interacted with her classrooms throughout the academic year. The inquiry activity presented here builds directly upon JC’s graduate research, and is placed within the context of learning about photosynthesis and stable isotopic analysis. This activity can be utilized as part of a chemistry or biology course, and can be presented in collaboration with a university scientist (as here); by the teacher, with samples sent to a university lab; or by the teacher alone with hypothetical data.
1.3. The Concept of Photosynthesis (C3 and C4)
Prerequisites for this activity include a basic knowledge about photosynthesis and the major differences between C3 and C4 photosynthetic pathways; the photosynthetic pathways are named for the molecules in which carbon-dioxide is incorporated, either a 3-carbon or 4-carbon molecule. In addition to students understanding the light reactions of photosynthesis, students should have a detailed understanding of how carbon dioxide (CO2) enters a leaf, and how CO2 is broken down and synthesized into sugars in the Calvin cycle (also referred to as the Calvin-Benson cycle or light independent reactions). The current high school science standards do not support student learning at this level of detail in plant science; however, we believe this presents an opportunity for students to learn and apply advanced concepts.The Calvin cycle results in the breakdown and fixation of carbon-dioxide (CO2) into useable sugars using energy harvested from the light reactions. Carbon dioxide enters a leaf through tiny pores on the leaf surface called stomata (Figure 1). Although CO2 enters through these pores, it is not able to enter as quickly as water evaporates from these pores; therefore, there is a trade-off between water loss and carbon gain. This trade-off is controlled by guard cells that can close or open around stomata (Figure 1).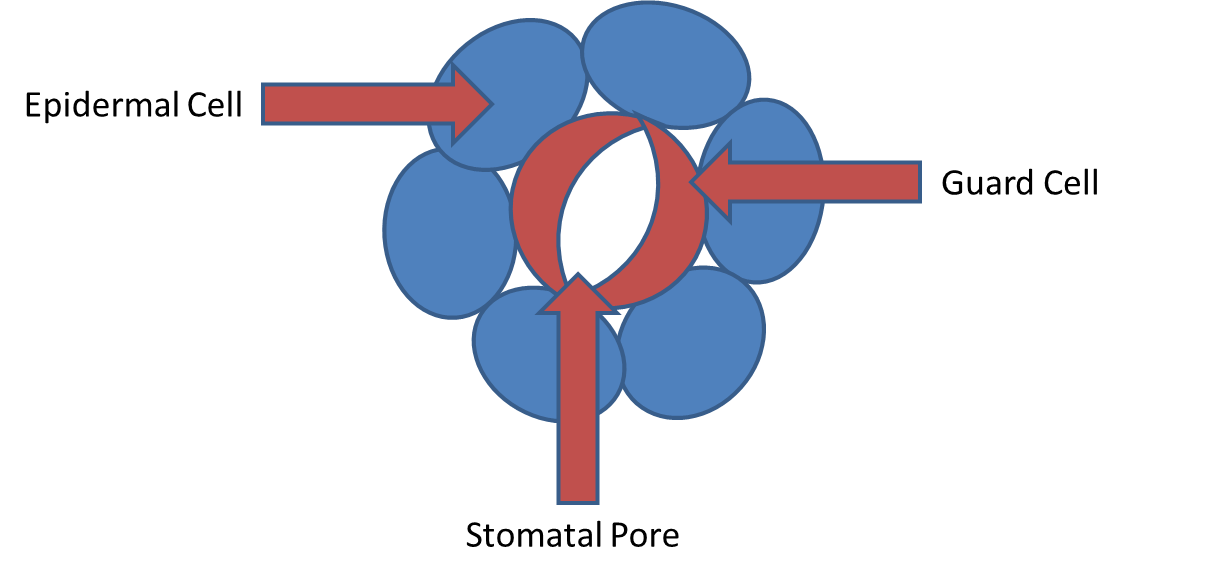 | Figure 1. A cartoon image depicting a stomate with guard cells |
Carbon dioxide is broken down in the Calvin cycle by the enzyme Ribulose-1,5-bisphosphate carboxylase oxygenase (RuBisCO) and subsequently fixed into sugars. This process happens in chloroplasts within mesophyll cells of the leaf in C3 plants, an area with a high concentration of molecular oxygen (O2). RuBisCO is able to catalyze a reaction between both CO2 and O2; however, when RuBisCO catalyzes a reaction with O2, the photosynthetic process is energetically wasteful requiring more adenosine triphosphate (ATP) than what is made during respiration. This wasteful process is known as photorespiration. C4 plants have evolved physiological and morphological adaptationsto lower rates of photorespiration. Plants with the C4 photosynthetic pathway have different leaf anatomy (Kranz anatomy; Figure 2) and use a precursor enzyme to RuBisCO (phosphoenolpyruvate carboxylase; PEP carboxylase), which increases the efficiency of carbon fixation during the Calvin cycle. The Kranz anatomy refers to a “wreath” like cell-structure in which bundle sheath cells that are highly concentrated with chlorophyll are surrounded by mesophyll cells (Figure 2). PEP carboxylase has a high affinity to bind to CO2, and a very low affinity to bind to O2. When PEP carboxylase binds to CO2, it catalyzes a reaction that incorporates CO2 into a 4-carbon acid. It should be noted that PEP carboxylase does not break down CO2 as RuBisCO does, and RuBisCO is still needed to complete carbon fixation for C4 plants. The 4-carbon acid is transported to bundle-sheath cells where CO2 is highly concentrated compared to mesophyll cells, and where the concentration of O2 is very low reducing photorespiration.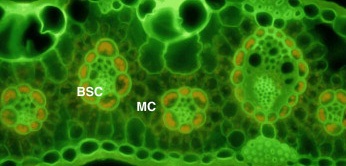 | Figure 2. Fluorescence image of a transverse section of a leaf from a C4 species (Pennisetumvillosum) with labelled bundle sheath cells (BSC) and mesophyll cells (MC) [9] |
The 4-carbon acid is decarboxylated giving off CO2. RuBisCO is then able to break down and fixCO2 into useable sugars as is done in C3 plants. The C4 photosynthetic pathway requires more energy than the C3 pathway; however, because RuBisCO’s affinity for O2 increases with temperature, C4 photosynthesis is a favorable process in warmer environments.
1.4. Isotopic Fractionation of Carbon-13
Isotopic elements have additional neutrons making up the atomic nucleus and can be either stable or radioactive. Radioactive isotopes decay over time, some faster than others. Carbon-14 (14C) is a radioactive isotope that is often used to date ancient materials due to its slow decay and known half-life. Stable isotopes, such as Carbon-12 (12C) and Carbon-13 (13C), do not undergo radioactive decay. Approximately 99% of carbon in CO2 in Earth’s atmosphere is 12C, and approximately 1% is 13C [10]. 14C accounts for only about 1 in 1 trillion carbon atoms [10]. Although many scientists study isotopes of other elements (e.g., oxygen, nitrogen, sulfur), here we focus on carbon due to its importance in the process of photosynthesis. When carbon rich sugars produced during photosynthesis are used to form structural tissues (e.g., leaves, wood), the isotopic signature (13C and 12C) of the CO2used to make those sugars is fixed in those tissues until they are decomposed. The δ13C of a tissue is first determined by measuring the ratio of all stable carbon isotopes in a given substance. For carbon, it is the ratio, 13C:12C.The13C:12C of the tissue being analyzed is then compared to a standard with a known 13C:12C of zero. For carbon, the standard is pee dee belemnite. The comparison with a standard is necessary to determine the extent that 13C is depleted in the tissue. The δ13C of most organismal tissue is negative because the tissue is depleted in 13C as compared to the standard. This depletion is seen because 13C is fixed in tissues at extremely low amounts as compared to the high amount of 13C fixed in the standard. In other words, the sample is depleted as compared to the standard. δ13C can vary in leaf tissue for several reasons. Processes that cause these variations in the δ13C are referred to as fractionations. 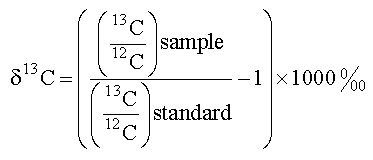 | Figure 3. 13C isotopic signature formula |
There are two major processes of fractionation in plants. The enzyme RuBisCO discriminates greatly against 13C and will preferentially fix 12C when available. It is enzymatically unfavorable for RuBisCO to fix 13C due to the excess energy needed, this is because 13C is more massive than 12C having an additional neutron in its atomic nucleus. In C3 plants, this fractionation process accounts majorly for the depletion of 13C in leaf tissue. In C4 plants, the precursor enzyme PEP carboxylase does not discriminate as strongly against 13C as RuBisCO. Instead, the 4-carbon acids of C4 plants “deliver” both 13CO2 and 12CO2 to RuBisCO in the bundle sheath cells where the enzyme has to fix whatever isotopic form of CO2 it receives. Consequently, there is little room for RuBisCO to discriminate against 13CO2 in C4 plant species and more 13C is fixed in C4 plants.For both C3 and C4 plants, CO2 containing 13C diffuses more slowly through the stomata which also affects the δ13C of leaf tissue. The rate at which CO2 diffuses into the leaf through stomata is dependent on the concentration of CO2 in the intercellular air spaces inside the leaf. Since diffusion happens from an area of higher concentration to lower concentration, the diffusion of CO2 from the atmosphere through stomata will happen fastest when the concentration of CO2 is low inside the leaf. Low concentrations of CO2 inside the leaf are generally due to drought stress as guard cells close around stomata to prevent water loss. If guard cells close to prevent water loss, RuBisCO is “forced” to fix 13CO2, and this will result in a less depleted (more positive) δ13C. Because of the difference in the photosynthetic pathways, specifically the use of different enzymes, leaf tissue of C3 and C4 plant species will differ greatly in their δ13C. Leaf δ13C of C3 plants will be more negative (generally in the range of -30 to -25‰) than leaf δ13C of C4 plants (generally in the range of -14 to -12‰).
1.5. Mass Spectrometry
Mass spectrometry is the method of choice for determining the relative abundances of stable isotopes in a given sample. The first machine used in the method of mass spectrometry was called a “mass spectrograph” [11]. The mass spectrograph was useful in detecting isotopes of many different elements in samples, but it could not determine the abundances of these isotopes. The mass spectrograph evolved into the “mass spectrometer” which is still used today and can determine the relative abundances of different isotopes within a given sample. In the case of leaves, samples are first dried at 60°C in a drying over for 48 hours. The dried leaves are then ground into a fine powder using a mortar and pestle and liquid nitrogen. A small amount of this powder (2-3 mg) is loaded into a tiny tin foil cup.The tin foil cups are placed into the mass spectrometer where the sample is first heated and vaporized. The sample is then ionized by a beam of electrons. After ionization, the sample is accelerated by an electric field with high-voltage difference, and is focused into a narrow beam of ions by the use of collimating slits and electronic lenses. The ion beam then passes through magnetic and/or electric fields that resolve the single ion beam into several distinct ion beams. Each of these beams is composed of ions that have similar characteristics, such as mass. Each of these individual beams hits a detector plate that electronically quantifies and subsequently translates the relative abundances of each type of ion in the sample. Isotopic elemental atoms with additional neutrons in their nucleus are more massive, and so their ions will also be more massive. Thus, the differentiated beams created by the mass spectrometer are representative of different isotopes (e.g., 12C vs. 13C). The relative abundances given by the mass spectrometer can then be used to calculate δ13C.
2. The Crime Solving Activity
2.1. Course Context and Concept Introduction
We used this activity in a junior/senior level advanced Chemistry course, and therefore needed to bring in the necessary content on photosynthesis before students conducted the exercise (explained generally in sections 1.2 and 1.3). Students in a Biology class could be given this activity as a part of a unit on photosynthesis. Students must learn why 13C isotope fractionation is different between the C3 and C4 photosynthetic pathways. We explained that the enzyme RuBisCO in C3 plants discriminates against 13C, but the enzyme PEP-Carboxylase used in C4 plants does not discriminate against 13C to as great a degree. This leads to a greater amount of 13C being fixed into sugars in C4 photosynthesis.
2.2. Teacher Procedures
Divide the class into groups (we used four groups) and provide a story about a modern day celebrity. We presented the victim as a famous rapper known to students (any particular celebrity could be posed as the character). We created a story wherein the celebrity dies from anaphylactic shock from an edible C4 plant (Box 1). They have at their disposal a scientist, Agent “Y” (Agent Carter in our case), who can run samples on a mass spectrometer. Students play the role of crime scene coroners.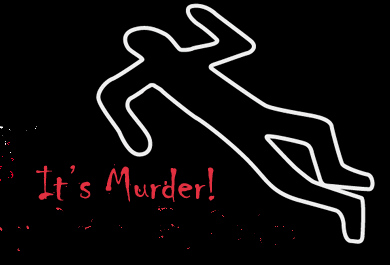 | Figure 4. The hand-out given to students that explained the crime, and put them on the case to solve the murder of Victim “X” |
What Killed Victim “X?” “Victim X” was at his favorite restaurant, “The Crème de la Crème,” on Friday night. As the waitress approached “Victim X” and his party, she announced that tonight the restaurant had a superb southwestern salad that included many different types of lettuce, some tomato salsa, black beans, and a southwest dressing that was garnished with corn leaves. “Victim X” thought the salad would be extremely delicious, so he ordered it. However, he asked the waitress to make sure that the corn leaf garnish would not be included because he is severely allergic to corn. “Victim X” and his crew enjoyed their salads and the rest of their meals until dessert, when “Victim X” suddenly appeared ill, and then collapsed at the dinner table dead. You are pronounced as the crime scene coroner on this case. It is your job to figure out how “Victim X” died. We have sent the stomach remains from the crime scene to you immediately. The crime scene investigators need to know if the corn leaf garnish was in the stomach contents or not. You have Agent “Y” at your disposal; he can run any plant materials through a mass spectrometer and give you the results. Using your knowledge of the isotopic signature of C4 plants, you should be able to tell us if we have a murder on our hands or not. Good luck Coroner, you’ll need it!Mix various types of leaf lettuce, as well as black beans and tomato salsa (all C3 species). Add to the mixture pieces of corn leaves (a C4 species). Water with red and blue food coloring can be added to the mixture. Separate the mixture into plastic sandwich bags and the bags will serve as the “stomach contents” (we added a can of black beans and its juices to give the contents a distinct smell to increase the “gross factor” as well).Instruct the students to sort the “stomach contents” with forceps and to put their samples into coin envelopes to be sent to the stable isotope mass spectrometry lab. This can be done in collaboration with a researcher at a nearby university, or by locating a mass spectrometry lab (nearby or not) and sending samples. Samples can be run fairly cheaply — Carbon-13, or δ13C, analysis will cost ~$6.00 per sample. We allowed students to analyze 5 samples across four groups and then pooled the class data to make these analyses less costly (approximately $120 total). Alternatively, hypothetical data can be used (those presented here, or other). To find an appropriate facility nearby search for “stable isotope facility [your state’s name inserted here]”. We used the stable isotope facility at Kansas State University (http://www.k-state.edu/simsl/SIMSL_Home.html). It is a good idea to contact the facility several months before sending samples to ensure they are prepared to run the samples immediately.
2.3. Additional Caveats and Resulting Data
We did not give the students any instruction as to how to sort or label the contents as we wanted to be able to discuss the importance of sampling and labeling as well as the idea that different scientists use different methods in the post-discussion activity. The process of sorting, sampling and labeling led students to think about what their research objective was and how their sampling technique should be appropriate for their specific research question. Students asked questions such as “should we collect everything or just the leaves?” or “not all the lettuce leaves look the same, should we collect them all?” After discussion within their group, most students decided that only leaves needed to be collected and processed their samples by sorting different types of lettuce. Some groups processed all the stomach contents including all the vegetables and fruits. Neither processing method is wrong, but the story says that “Victim X” was allergic to corn leaves. Therefore, only the leaves are necessary to answer the question “was a corn leaf in “Victim X’s” salad?”You could vary this (e.g., if corn kernels were included, they would also show a C4 signature).The scenario posed is clearly fictitious, and some students may point out that it’s not entirely realistic. For example, stomach contents would be obtained during an autopsy, not from the crime scene itself (we liked using stomach contents because students enjoyed the “gross” scenario). The anaphylactic shock scenario is somewhat unrealistic (and it’s likely that an individual with a severe corn allergy would avoid restaurants all together). This sort of situation wouldn’t necessarily be ruled a crime, or “murder.” It’s important to clarify to the students that the activity was developed to demonstrate how a particular technology can provide information about plants present, and link to real uses of mass spectrometry by scientists (discussed below).Figure 5 provides sample data that one would receive from a stable isotope lab. From data like this, our students were able to infer that a corn leaf was indeed ingested by “Victim X” (we included the corn leaf). An alternate course in this lesson would be to leave the corn leaf out, which would suggest the death of “Victim X” was due to something else. We also suggest including a control for the corn leaf and a control for a non-corn leaf (any C3 leaf lettuce will work) so that students can see the expected δ13C for both, and to demonstrate the importance of experimental controls in scientific studies. 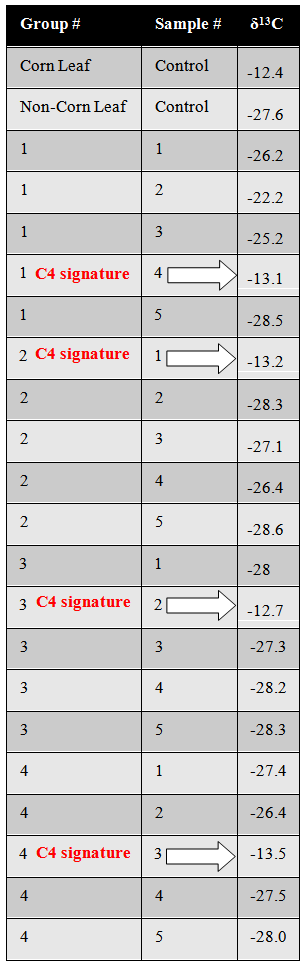 | Figure 5. Sample data that would be received from an isotope facility for 13C analysis of leaf tissue. The C4 signatures from corn leaves are indicated by white arrows within each group |
2.4. Student Procedures
Use stable isotopic evidence to determine if “Victim X” died from ingesting a corn leaf (see Figure 1). To do this, sort and label any important evidence from the stomach contents. When the data from the mass spectrometer analysis of your samples are returned, determine whether corn leaf material was present in “Victim X’s” salad and could be responsible for his death.
2.5. Assessment
This activity itself can be used as a performance assessment for a unit on isotopes in a chemistry course or a unit on photosynthesis in a biology course. Students correctly interpreting the mass spectrometer data to indicate the presence or lack of a corn leaf provided evidence that they understand that corn leaves are C4 plants, and that they were able to identify the presence of a C4 plant based on the isotopic signature in their samples.We wanted to know if the students could apply this knowledge to a real research situation. We gave students the prompt: “Explain how a plant makes sugar out of inert carbon dioxide. Also, if we analyzed a leaf for its stable isotopic composition and discovered it was -13%, does this plant use a C3 or C4 photosynthetic pathway? Answer these questions and explain using the following terms: Light reactions, Calvin cycle, RuBisCO, Pep-Carboxylase, 12C and 13C, stomata, ATP, NADPH, CO2 and Ribulose-1, 5-BisPhosphate.” Instead of a prompt, teachers could also ask students to complete a concept map using these terms for assessment. Although individual differences existed among the essay answers, 12 out of the 14 students answered the questions correctly. Examples of student answers are presented below: Student #1“In the process of photosynthesis, plants take in CO2 and convert this into usable energy called sugar or glucose. This process of photosynthesis first starts out with the Calvin’s [sic] Cycle in which a leaf takes in light and carbon dioxide through the stomata and then goes through the first step of the Calvin Cycle. The cycle uses a catalyst in the form of an enzyme, RuBisCO. Then the proceeding steps use ATP and NADPH to form sugar.Some plants can use 12C and others can use 12C and 13C. The difference is between C3 and C4 plants, respectively. Another difference is that C4 plants have an enzyme called, PEP-Carboxylase, that allows them to use both forms of carbon isotopes. A way to tell the difference between the two types of plants are that C4 plants stable isotopic fractionation factors are much closer to zero than C3 plants. This is due to the different weights of the isotopes.”Student #2“In light reactions, CO2 enters through the leaf’s stomata and combines with ribulose-1,5-bisphosphate. CO2 gas enters the second step in the Calvin’s Cycle in which the molecule breaks down into two 3-carbon molecules. Next, ATP adds phosphate groups to both ends of the molecule. Following that, NADPH takes one phosphate group from the molecule. This molecule becomes G3P and two G3P molecules combine to make one molecule of glucose. If the plant is a C4 plant, then a precursor step is added in which PEP-Carboxylase combines with CO2 for more efficient use of CO2.If a plant was examined and had a fractionation factor of -13‰ that would indicate that it had a 13C isotopic signature since it is a heavier isotope than 12C. Discovery of a 13C isotopic signature indicates that the plant is a C4 type since 12C isotopic signature is most common in C3 plants.”
3. Conclusions
3.1. Follow-up Discussion
Explicitly involving students in hands-on science by using current research techniques and involving scientific researchers can get students excited about science, increase their breadth of knowledge, and give them the tools to critically assess the world around them. To end this lesson, we had a discussion with students about the applicability of stable isotopes to real-word problems. Although stable isotopes are used to address various scientific issues, we discussed their application in global climate change research since this is a popular topic often appearing in mass media. Stable isotopic analyses are a valuable tool to climate-change scientists, and are often reported as evidence of climate change in scientific journals and in popular media. For example, stable oxygen isotopic analysis has provided information regarding the climate of Earth thousands of years in the past, allowing us to better understand how Earth’s climate system has functioned in the past, and how it will likely function in the future. 13C analysis has allowed us to better understand how plants will respond to future increases in temperature and drought expected due to climate change, which will be especially important to the future of farming and our food supply. Along this line of crop management, we asked our students during our discussion to think about how δ13C would be different for C3 plants in future warmer and drier environments, and how that information may influence the management of plant germplasm in the future. 13C analysis has also provided evidence that humans are increasing the concentration of CO2 in Earth’s atmosphere since it is old fossilized plants (fossil fuels) that are being burned for energy (the released CO2 carries the 13C signature of the fossilized plants, which has changed the carbon signature on average of CO2 in Earth’s atmosphere) [12]. Teachers could also discuss how stable isotopes can be used to infer what the diets of ancient peoples and animals were using fossilized teeth. In this activity, students demonstrated that they could comprehend a very complex biochemical topic (stable isotope analysis) and use this knowledge in a research situation. This activity used a popular topic, crime-scene investigation, to engage students in demonstrating how concepts they learn in their course (photosynthetic pathways and isotopes) are used in real, every day, scientific research. This and similar lessons from the KSU GK-12 program are available on the website www.ksu.edu/gk12.
3.2. Standards
3.2.1. Next Generation Science Standards
Science & Engineering Practices
• Planning and carrying out investigations
• Constructing explanations and designing solutions
• Scientific investigations use a variety of methods Disciplinary Core Ideas
• Structure and properties of matter (HS-PS1-1)
• Organization for matter and energy flow in organisms (HS-LS1-5; HS-LS1-6; HS-LS1-7)
3.2.2. National Science Education Standards
Content Standard A- Scientific Inquiry
• Abilities necessary to do scientific inquiry
• Identify questions
• Design and conduct scientific investigations
• Use technology and mathematics to improve investigations and communication
• Formulate and revise scientific explanations and models using logic and evidence
• Communicated and defend a scientific argument
• Understandings about scientific inquiryContent Standard B- Physical Science
• Structure of atoms
• Structure and properties of matter
• Chemical reactionsContent Standard C- Life Science
• The cell
• Matter, energy, and organization in living systems
ACKNOWLEDGEMENTS
We would like to thank Teresa Woods, Laci Gerhart, and Katie Becklinfor their feedback that greatly improved an earlier version of this manuscript. We especially thank Dr. Carolyn Ferguson who directed the GK-12 EIDRoP program, and also provided feedback on this manuscript. We also would like to acknowledge the NSF GK-12 EIDRoP program at Kansas State University (#DGE-0841414). Finally, we would to thank our students who participated in our lesson and were open to learning a challenging topic.
References
[1] | NGSS Lead States, Next generation teaching standards: For states, by states, Washington, D.C.: The National Academies Press, 2013. |
[2] | National Committee on Science Education Standards and Assessment, National Science Education Standards, Washington, D.C.: The National Academies Press, 1996. |
[3] | Briggs, B., Mitton, T., Smith, R., Magnuson, T., “Teaching cellular respiration and alternate energy sources with a laboratory exercise developed by a scientist-teacher partnership,” The American Biology Teacher, 2009, 71, 164-167. |
[4] | Shell, D.F., Snow, G.R., Claes, D.R., “The Cosmic Ray Observatory Project: Results of a summer high-school student, teacher, university scientist partnership using a capstone research experience,” Journal of Science Education and Technology, 2011, 20, 161-177. |
[5] | Dolan, E., Tax, F., “Partnership for research &education in plants (PREP): Involving high school students in authentic research in collaboration with scientists,” The American Biology Teacher, 2011, 73, 137-142. |
[6] | Mumba, F., Mejia, W.F., Chabalengula, V.M., Mbewe, S., “Resident scientists’ instructional practice and their perceived benefits and difficulties of inquiry in schools,” Journal of Baltic Science Education, 2010, 9, 187-195. |
[7] | Carter, J.M., Nippert, J.B., “Physiological responses of Tamarixramossima to extreme NaClconcentrations,” American Journal of Plant Sciences, 2011, 2, 808-815. |
[8] | Carter, J.M., Nippert, J.B., “Leaf-level physiological responses of Tamarixramossisimato increasing salinity,” Journal of Arid Environments, 2012, 77, 17-24. |
[9] | Christin, P., Freckleton, R.P., Osborne, C.P., “Can phylogenetics identify C4 origins and reversals,” Trends in Ecology and Evolution, 2010, 25, 403-409. |
[10] | Farquhar, G.D., Ehleringer, J.R., Hubick, K.T., “Carbon isotope discrimination and photosynthesis,” Annu. Rev. Plant Physiol. Plant Mol. Biol., 1989, 40, 503-537. |
[11] | Criss, R.E., Principles of Stable Isotope Distribution, Oxford University Press: New York, NY, 1999. |
[12] | Peterson, B.J., Fry, B., “Stable isotopes in ecosystem studies,” Ann. Rev. Ecol. Syst., 1987, 18, 293-320. |