Joseph C. Sloop, Leonard Anagho, Patrick Coppock, Greta Giles, Sang Park, Richard Pennington, David Pursell, Gillian Rudd, Mai Yin Tsoi
School of Science and Technology, Georgia Gwinnett College, Lawrenceville, GA, 30043, USA
Correspondence to: Joseph C. Sloop, School of Science and Technology, Georgia Gwinnett College, Lawrenceville, GA, 30043, USA.
Email: |  |
Copyright © 2012 Scientific & Academic Publishing. All Rights Reserved.
Abstract
Cyclohexane derivative stability is investigated using a combination of molecular modeling kits, conformational analysis, computational chemistry and polarimetry. Students build selected mono- and disubstituted cyclohexanes using model kits, predict the most stable conformation and calculate conformational equilibria. Students also construct cis-1,2-dimethylcyclohexane and trans-1,2-dimethylcyclohexane using the Avogadro computational chemistry program, optimize the structures and confirm the lowest energy conformations. Students utilize a polarimeter to measure the optical activity of several unknown cyclohexane derivatives and identify them from a list of possible (±) menthol and (±) carvone stereoisomers.
Keywords:
Cyclohexane, Polarimetry, Conformational Analysis
Cite this paper: Joseph C. Sloop, Leonard Anagho, Patrick Coppock, Greta Giles, Sang Park, Richard Pennington, David Pursell, Gillian Rudd, Mai Yin Tsoi, Conformational Analysis, Modeling, Stereochemistry and Optical Activity of Cyclohexane Derivatives, Journal of Laboratory Chemical Education, Vol. 1 No. 3, 2013, pp. 39-44. doi: 10.5923/j.jlce.20130103.01.
1. Introduction
Chemistry faculty in the School of Science andTechnology at Georgia Gwinnett College recently revised the two - semester organic chemistry course sequence laboratory program as part of the Science, Technology, Engineering and Math (STEM) II initiative[1]. Our overarching goals were to expose students to a four - year undergraduateresearch - like experience focused on “green” organic chemistry principles, development of technique at the microscale and miniscale levels, incorporation of computational chemistry and instrumentation use[2, 3].One of the key explorations students undertake in the first semester of the organic chemistry course sequence is an experiment where they are introduced to the interplay of molecular modeling, computational chemistry,stereochemistry and optical activity displayed by the cyclohexane family of organic molecules[4].The student activities selected for this laboratory experience reflect the linkage with topics covered in the classroom and their practical application. These include: (a) Pre - lab Activities – use of the model kit to buildcyclohexane, reading the Avogadro molecular modeling program instructional guide and viewing the polarimeter use video and (b) In-lab Activities – using model kits to understand conformations of substituted cyclohexanes, determination of Keq and ΔG for cyclohexane ring flips, using model kits to understand cis-trans stereoisomerism in disubstituted cylohexanes, using the Avogadro molecular modeling program to compute substituted cyclohexane energies and finally, using polarimetry to identify optically active cyclohexane derivatives from a list ofunknowns[4,5,6,7,8]. The in-lab experimental activities requireapproximately two hours to complete.
2. Materials and Instrumentation
The materials and instrumentation used for this experiment include:1. Molecular model kit2. Experimental Instructions (contained in the on-line Laboratory Text – Chemistry 2211K/2212K)3. Polarimeter Use Video[9]4. Avogadro Program Instructions (contained in the on-line Laboratory Text – Chemistry 2211K/2212K)5. Laptop computers (12) with Avogadro program Installed[6,7]6. Cyclohexane derivatives: (+) menthol (0.14 g/mL ethanol), (-) menthol (0.20 g/mL ethanol), (+) carvone (0.50 g/mL ethanol), (-) carvone (neat)[5] 7. Azzota Polarimeter with four polarimeter sample tubes (2 X 1dm, 2 X 2dm)[8]
3. Experimental Procedure
To prepare for the experiment, students are expected to read the portion of the laboratory text that corresponds to this experiment, perform the Pre-lab Activities and prepare their lab notebook in accordance with the course laboratory manual. In the lab, students are given a quiz at the outset of the lab that tests their preparedness. In-lab Activities are then conducted by the students working either in small groups or individually at the discretion of the instructor. The complete experimental writeup is available from the corresponding author.
3.1. Pre-lab Activities
3.1.1. Activity 1
Using a molecular model kit, build a model of cyclohexane, using a colored model sphere for one of the shaded atoms (see Figure 1). Then “flip” the model into the other chair conformation. Describe what happens to the colored sphere in your lab notebook, before and after the “flip”. Then, describe what happens to the other atoms within the cyclohexane molecule and the substituents around the ring.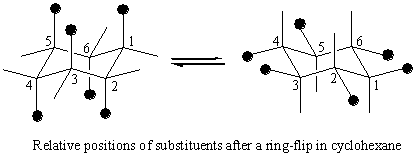 | Figure 1. Ring-Flip of Cyclohexane |
3.1.2. Activity 2
Read the instructional guide to using the Avogadro molecular modeling program found at Activity D.
3.1.3. Activity 3
View the video for using the Azzota Disk Polarimeter[9].
3.2. In-lab Activities
3.2.1. Substituent Effects in Cyclohexanes-Conformational Analysis
We will now consider substituent effects on ring stability in mono- and disubstituted cyclohexane rings. We will investigate unfavorable ‘steric hindrance’ problems in certain conformations caused by substituents. Finally, we will consider conformations with as little steric hindrance as possible – we consider these to be the most stable conformations and therefore the more commonly found conformations in nature.Steric hindrance is a term that you are going to be hearing (and using) a lot in this course, so let us explore its meaning now. MethylcyclohexaneWith a single -CH3 substituent, methylcyclohexane can potentially exist in two chair forms:• when the -CH3 group is axial (A).• when the –CH3 group is equatorial (B).These chair forms are shown in Figure 2.The important, destabilizing effect here is known as the 1,3-diaxial interaction; the interaction refers to the repulsion between the axial methyl group and each of its neighboring axial hydrogen atoms. When substituents are this close to each other they “push” or repel each other away a little bit. This raises the energy of the conformation – and that decreases the structure’s stability[4,10].RULE #1: 1,3-diaxial interactions are only possible when the interacting substituents are in the_______________ position. (fill in the blank and rewrite the rule in your lab notebook) On the other hand, the conformation in which the methyl group is equatorial, is much more stable. Why is this? In this conformation, the methyl group is further away from the cyclohexane ring and is not in an axial position. Thus, no 1,3-diaxial interactions are present. One may quantify the difference in free energy (ΔG in kJ/mol) between the two conformations A and B if we know the concentrations of the conformers A and B at equilibrium by taking advantage of the relationship between ΔG and the equilibrium constant K, given by equation 1: | (1) |
where Keq = KAB =[B]/[A], R=8.314x10-3 kJ/mol.K, T=temperature in K.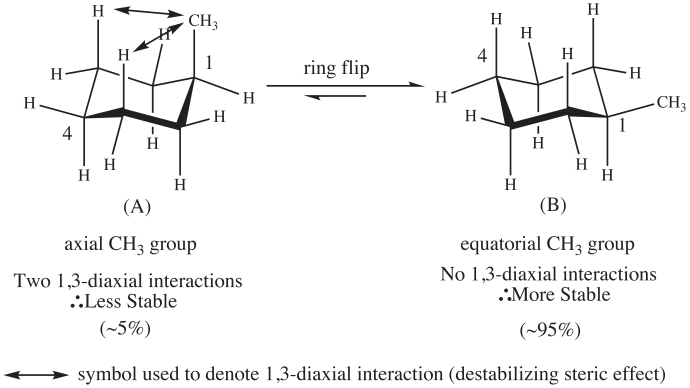 | Figure 2. Chair Forms of Methylcyclohexane |
ACTIVITY A: Calculate ΔG for the equilibrium between the chair conformations of methylcyclohexane, given the following equilibrium concentrations at 298K:[A] =0.05M;[B] = 0.95M.Substituents other than methyl also cause 1,3-diaxial interactions. Generally speaking, the larger the substituent, the greater the interaction and therefore, the greater the strain energy. Table 1 lists the steric strain arising from 1,3-diaxial interactions associated with a variety of common substituents[4,5].Table 1. Cyclohexane Steric Strain Energies 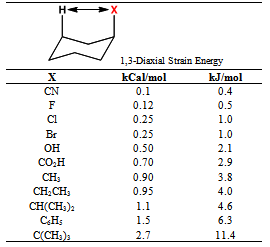 |
| |
|
ACTIVITY B: Build the following molecules with your model kit and determine which conformation (axial or equatorial) is the MOST stable configuration. Copy and fill out Table 2. below in your lab notebook, placing an X in the column that describes the most stable configuration for that molecule. Then, using the data from Table 1, calculate the total steric strain for each configuration. Table 2. Substituted Cyclohexane Steric Strain 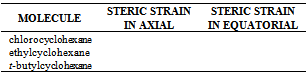 |
| |
|
What do you notice about the results of your Activity B data table? Which conformation is the most stable for each molecule? Describe in your lab notebook why you chose the configuration that you did.RULE #2: The larger the substituent, the ______________ the repulsive interaction when it occupies an axial orientation. (fill in the blank and rewrite the rule in your lab notebook) This means that the larger the 1,3-diaxial interactions, the more unstable the molecule becomes. This is particularly evident when looking at t-butyl substituents. These are so big and bulky, with correspondingly large 1,3-diaxial interactions, that axial t-butyl groups in cyclohexane rings are quite rare as indicated in Figure 3: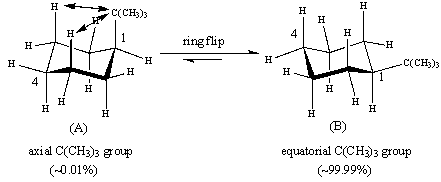 | Figure 3. Chair Conformations of t-Butylcyclohexane |
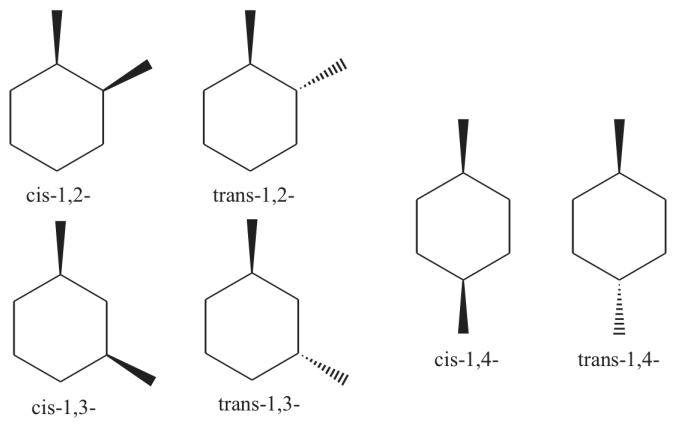 | Figure 4. Stereoisomeric Dimethylcyclohexanes |
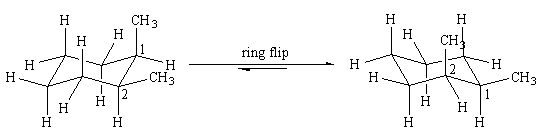 | Figure 5. Chair Conformations of cis-1,2-Dimethylcyclohexane |
ACTIVITY C: Calculate Keq for the equilibriumA
B between the chair conformations of t-butylcyclohexane, given a value of ΔG = -22.82 kJ/mol at 298K.
3.2.2. Stereochemistry of Cyclohexane Derivatives – Cis/trans Isomerism and Stability, Polarimetry and Optical Activity
Cis-trans Isomerism and Stability - Disubstituted CyclohexanesConsider the six stereoisomeric dimethylcyclohexanes shown in Figure 4:For each of these isomers, we can draw the two chair conformations. We can also predict which of those conformers is the more stable in each case, if any. Let us look at the two chair conformations of cis-1,2 -dimethylcyclohexane: Which is the more stable? Look for the number of 1,3-diaxial interactions in each conformation. If there are the same number of these interactions, then the conformations are equally stable. Exactly the same procedure can be performed for the remaining isomers.ACTIVITY D: Using the Avogadro molecular modeling program, construct cis-1,2-dimethylcyclohexane and trans-1,2-dimethylcyclohexane. Determine the overall energies of the isomers and identify which is more stable. Follow the steps below.1. On your computer, open the Avogadro application. There should be several icons along the top of the Avogadro window along with 2 buttons, (1) “Tool Settings…” and (2) “Display Settings…”. Click the buttons (1 and 2) a couple times to see what they do. They should make tool frames appear and disappear. Most students like to use those frames, so when you are done, leave those frames open.2. Make a structure.a. To make dimethylcyclohexane, you can either build the structure atom by atom, or start from a template structure. Here we describe the latter, which requires an active internet connection or the template structure saved to disk. Click on File > Import > Fetch chemical structure… A dialog box should appear with an entry field. In it, type “cyclohexane”, then click “OK”. A cyclohexane molecule should appear in the main field of the main Avogadro window.b. Click on the navigation tool (or F9) or manipulation tool (or F10) and orient the molecule to make it easy for you to see. It could be good at this point to mouse-over each of the icons along the top of the main window to see what they do, and then click on them and practice with them. Click on the draw tool (or F8). Make sure the “Element” is “Carbon”, and “Bond Order” is “Single”. The “Adjust Hydrogens” box should be selected. Then, to maketrans-1,2-dimethylcyclohexane, click on two hydrogens which are next to one another and trans. Click on the navigation tool or manipulation tool and rotate the molecule in space and verify that you have made trans-1,2-dimethylcyclohexane.3. Optimize the structure. The molecule described above does not have optimal bond length and bond angles. In order to “optimize” it, first click on Extensions> molecular Mechanics>Setup Force Field and verify the foce field is set to MMFF94, then click on Extensions> Optimize Geometry.4. Calculate a configuration energy. Click on Extensions > Molecular Mechanics > Calculate Energy. Record the energy.5. Repeat steps (2) and (3) above to make the cis-1,2-dimethylcyclohexane structure.Example Avogadro output files for the structures and energies of the 1,2-dimethylcyclohexane isomers students obtain are shown in Figure 6.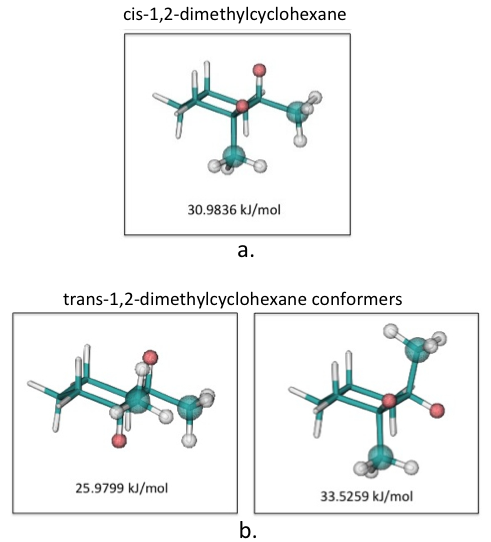 | Figure 6. 1,2-Dimethylcyclohexane Isomer Avogadro Output Files |
ACTIVITY E: Build with your model kit, and then draw the two chair conformations for the 1,3- and 1,4- isomeric dimethylcyclohexanes. Then show, for each pair, which conformation is more stable (if any). Clearly explain your answer in each case. Organize your results in a table. Exactly the same line of reasoning can be used to describe relative stabilities of chair forms when the substituents are different. Just remember that the larger the substituent, the larger the 1,3-diaxial interactions when that substituent is in an axial orientation. This results in a less stable conformation.ACTIVITY F: For cis-1-methyl-4-t-butylcyclohexane and trans-1-methyl-4-t-butylcyclohexane, show which is the more stable. Clearly explain your answer, with structures/ diagrams or numbers to support your answer.Measuring Optical Activity in Cyclohexane Derivative Stereoisomers by PolarimetryCyclohexane derivatives which have one or more stereogenic centers may exhibit a unique property called optical activity (the ability to rotate a plane of polarized light). This property can be measured through use of an instrument called a polarimeter. For a known molecule which exhibits optical activity, a solution of the compound at known concentration may be prepared and the specific rotation,[α], determined using the following equation and parameters:
The instrument we will use for this part of the experiment is the Azzota Disc Polarimeter. Figure 7 shows the major components and the sample tubes.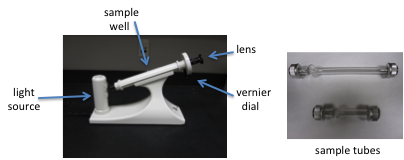 | Figure 7. Azzota Polarimeter and Sample Tubes |
ACTIVITY G: In this experiment, you will examine one of four unknown optically active cyclohexane derivatives, measure the angle of rotation with the polarimeter and identify it based on its specific rotation. The possible compounds are shown in Figure 8 and are labeled as compounds A-D: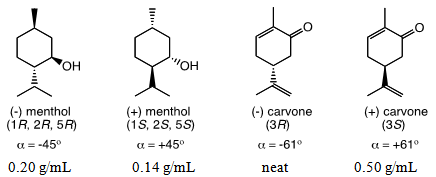 | Figure 8. Optically Active Compounds and Concentrations |
The specific rotation (α) values given above for (+/-) menthol and (+/-) carvone are given in Figure 8. The observed optical rotation that you will measure is based on the concentration of the solutions in the polarimeter sample tube.Follow the steps below to determine the angle of rotation (Q) for your optically active unknown by polarimetry.1. Make sure the polarimeter has been turned on for at least 10 minutes before attempting to make a measurement.2. Adjust the vernier (rotary hand wheel) dial until the reading is at or near 0. 3. Place one of the unknown sample tubes into the polarimeter under the guidance of the instructor.4. While looking through the lens, you will observe one of the patterns of light shown in Figure 9: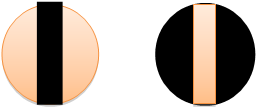 | Figure 9. Azzota Polarimeter Light Patterns – Pre-Measurement |
5. Adjust the rotary hand wheel dial slowly until you obtain a pattern similar to that shown in Figure 10: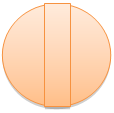 | Figure 10. Azzota Polarimeter Light Pattern – Point of Measurement |
6. Observe the measured angle of rotation (Q) (0-180) through the magnifying lens. Values < 90 indicate “+” isomers. Values > 90 indicate “-” isomers and Q is determined by subtracting 180 from the observed rotation value. For example, if the observed value is 110, then Q = 110 - 180 = - 70.7. Using the formula supplied above, calculate the specific rotation,[α] and write the result in your lab notebook.8. Determine your unknown stereoisomer by comparing your specific rotation to the values in the laboratory manual.
4. Conclusions
This marks the second semester that this experiment has been in use. Overall, students have found use of the Avogadro computational chemistry program in conjunction with molecular models much more beneficial inunderstanding stability in molecules than models alone. The addition of polarimetry provides practical application of the theory of optical activity learned in class while helping students gain exposure to another type of instrumentation.
ACKNOWLEDGEMENTS
The authors thank the Georgia Gwinnett College School of Science and Technology STEM II Initiative Grant for funding this work.
References
[1] | University System of Georgia STEM Initiative Phase II, 2011. http://www.usg.edu/educational_access/stem |
[2] | J. Sloop, R. Pennington, M. Tsoi, J. Paredes, D. Pursell, P. Coppock, G. Giles, Laboratory Manual for Chemistry 2211K/2212K. School of Science and Technology, Georgia Gwinnett College: 2012. |
[3] | S. Abhyankar, “Introduction to Teaching Green Organic Chemistry, “ in Green Organic Chemistry in Lecture and Laboratory, A. P. Dicks, ed. CRC Press, Boca Raton, FL, 2012, 1-29. |
[4] | J. Smith, “Alkanes,” in Organic Chemistry, 3rd edition, McGraw-Hill: New York, NY, 2011, 125-147. |
[5] | J. Sloop, R. Pennington, M. Tsoi, J. Paredes, D. Pursell, P. Coppock and G. Giles, “Conformational Analysis, Modeling, Stereochemistry and Optical Activity of Cyclohexane Derivatives,” in Laboratory Manual for Chemistry 2211K/ 2212K. School of Science and Technology, Georgia Gwinnett College: 2012, 42-50. |
[6] | Avogadro: an open-source molecular builder andvisualization tool. Version 1.0.3 http:// avogadro. openmolecules. net/ |
[7] | M. Hanwell, D. Curtis, D. Lonie, T. Vandermeersch, E. Zurek, G. Hutchison, “Avogadro: An advanced semantic chemical editor, visualization, and analysis platform,” J. Cheminformatics, 2012, 4, 1-17. |
[8] | Operating Instructions, Disk Polarimeter, Azzota Corporation, Randolph, NJ, 2012, 1-7. |
[9] | Polarimeter video, (http://podcaster.gcsu.edu/podcastdata/ GGC/Channel_6888/podcast_ 474572/474572.mov) |
[10] | J. Sloop, “Stability of Carbon Compounds and Reactive Intermediates,” in Succeeding in Organic Chemistry: A Systematic Problem-Solving Approach to Mastering Structure, Function and Mechanism, AuthorHouse Publishing: Bloomington, IN, 2010, 36-39. |