Vladimir M. Petruševski, Miha Bukleski, Marina Stojanovsk
Department of Chemistry, Faculty of Natural Sciences and Mathematics, Ss Cyril & Methodius University, Skopje, 1000, Republic of Macedonia
Correspondence to: Vladimir M. Petruševski, Department of Chemistry, Faculty of Natural Sciences and Mathematics, Ss Cyril & Methodius University, Skopje, 1000, Republic of Macedonia.
Email: |  |
Copyright © 2012 Scientific & Academic Publishing. All Rights Reserved.
Abstract
An aqueous solution of sulfur dioxide in a sealed tube is stable. If, however, the solution contains iodide ions (in the form of KI that was dissolved previously) a slow disproportionation reaction occurs. This was interpreted in terms of the catalytic activity of the iodide anions. A possible chemical explanation was also offered. The disproportionation reaction was found to be much faster (by 2 orders of magnitude) if the solution contained H+(aq), and this is in line with simple chemical reasoning.
Keywords:
Sulfur Dioxide, Potassium Iodide, Catalyzed Disproportionation, Marathon Experiment
Cite this paper: Vladimir M. Petruševski, Miha Bukleski, Marina Stojanovsk, On the Catalyzed Disproportionation of SO2 in Aqueous Solution of KI: A Marathon Classroom Demonstration, Journal of Laboratory Chemical Education, Vol. 1 No. 1, 2013, pp. 1-4. doi: 10.5923/j.jlce.20130101.01.
1. Introduction
It is well known that alkali halogenides are soluble in liquid sulfur dioxide[1, 2]. Their solubility increases as the effective ionic radius of the anion increases. This solubility is to a certain extent possible as a result of the formation of clathrates between the alkali halogenide and the sulfur dioxide. Consequently, alkali iodides will be the most soluble halogenides in liquid sulfur dioxide. The solubility of typical ionic compounds (like the above-mentioned) is facilitated by the fact that SO2 is a polar substance. Also, in liquid SO2 (analogously as in liquid water) there exists self-ionization, according to the equation[3]: | (1) |
Knowing that the conductivity of liquid SO2 is much lower than that of pure water, it is easy to conclude that the number of charge carriers (ions) in SO2 is much lower than in water (actually, the difference is by a few orders of magnitude). This can be checked using a relatively simple equipment[4]. When sulfur dioxide is dissolved in distilled water, various hydrates are present (all having the general formula SO2·nH2O). The species that are formed between SO2 and H2O can vary depending on the concentration of SO2, the temperature of the system and the pH of the solution. The ions present in the system are: H3O+, HSO3−, S2O52− and some traces of SO32− as well[2]. At 0 °C a cubic clathrate hydrate forms with a composition ~ SO2·6H2O.Upon dissolution of potassium iodide in liquid SO2, the color of the solution becomes brownish-orange to brownish-red[2, 4], depending on the concentration of KI. This color is due to the formation of KI(SO2)2 and KI(SO2)4 clathrates[2]. These clathrates are relatively stable in water solutions as well (where the color is yellow). However, when these aqueous solutions are sealed in glass ampoules[5] and are left for several months, the yellow color disappears. A colorless liquid is obtained instead, and some pale yellow powder-like solid could be identified at the bottom of the ampoule.In order to explain the above preliminary observations, we decided to investigate the behavior of the closed system obtained by dissolving sulfur dioxide in an aqueous solution of potassium iodide. This experiment can last for more than half a year; hence it can serve as an example of a marathon classroom demonstration[6]. The idea was to explain the chemical changes that occurred in the above system on the grounds of some basic chemistry knowledge.
2. Experimental
2.1. Generation of SO2
Five grams of sodium sulfite are put into a side-armed flask connected to a glass tube bent at right angle that ends in a test-tube. A separation funnel is mounted on the neck of the flask for controlled addition of concentrated sulfuric acid diluted with equal volume of water (concentrated hydrochloric acid could be used as well, but sulfuric acid was chosen instead, in order to prevent traces of gaseous HCl to pass to the receiver). Upon addition of the acid, a reaction occurs, producing colorless gas (sulfur dioxide, SO2).
2.2. Preparation of SO2-saturated Solutions
The test-tube (the receiver) is placed in a beaker filled with ice-water, in order to enable more of the sulfur dioxide to be dissolved (at 0 °C, 22 g of SO2 can be dissolved in 100 g H2O). The gaseous SO2 is bubbling for about 10 minutes through the aqueous solution of potassium iodide with concentration of ≈ 1 mol/L. The liquid content of the test tube (which is by now pale yellow or yellow, if the solution is SO2-saturated) is transferred to a previously prepared test-tube (stretched with a narrow neck) and is immediately sealed in an ampoule[5]. One possible chemical equation for the gas-generation process is: | (2) |
Another ampoule was prepared, containing distilled water only (also saturated with SO2). The second ampoule was prepared as a control solution. The two ampoules were left for several months and the visible changes were monitored.
2.3. Planned Chemical Checks (~ 7 Months after Sealing the Ampoules)
When the entire amount of the yellowish solid is precipitated at the bottom of the ampoule containing dissolved KI (the blank remains clear, and at least seemingly, homogeneous), the ampoules are broken at the top. A few milliliters of the solution are taken by droppers from both ampoules. Then the solutions were tested for the presence of sulfate groups using an aqueous solution of barium chloride containing a small amount of hydrochloric acid. Formation of a white precipitate proves the presence of SO42− anions. Only the test-tube containing KI gave positive evidence for SO42−.The precipitate from the bottom of the ampoule containing KI was dried and an attempt was made to dissolve it in carbon disulfide. It dissolved, so it became clear that the solid is sulfur. This was further confirmed by evaporation of the CS2 in a hood and examination of the residue. The microscopic yellowish crystals were visually identical to what is known as rhombic sulfur. After these planned checks, the ampoules could be left open for a couple of days (to witness the changes described at the end of the paper), or be immediately disposed using proper chemical disposal techniques.
3. Results
When the gaseous sulfur dioxide bubbles through the colorless aqueous solution of potassium iodide, the liquid turns yellow (Fig. 1). This color is likely to be a result of the clathrates formed between the sulfur dioxide and the potassium iodide (the color is less intense than that of KI dissolved in liquid sulfur dioxide). These clathrates (as we mentioned above) are relatively stable in water. After four-to-five months, the solution in the test tube turns hazy (Fig. 2). This is a result of the formation of elemental sulfur. The sulfur is finely dispersed throughout the solution. Within two and a half months, the dispersed sulfur precipitates onto the bottom of the test tube and the remaining solution becomes clear and colorless (Fig. 3).Knowing the results of the planned chemical checks, i.e. that sulfate anions exist in the test-tube containing KI and that the pale yellow solid is elemental sulfur, we offer an explanation based on the following facts:a) Sulfur is oxidized from +4 to +6.b) Sulfur is reduced from +4 to 0.c) The above changes occur only when the solution contains KI.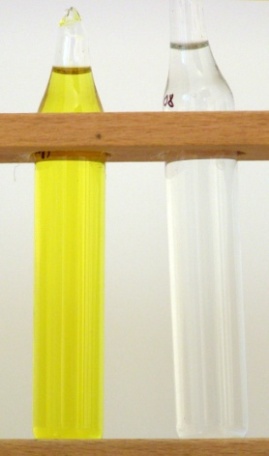 | Figure 1. Left test tube: SO2 dissolved in aqueous solution of potassium iodide; right test tube (a blank): SO2 dissolved in distilled water |
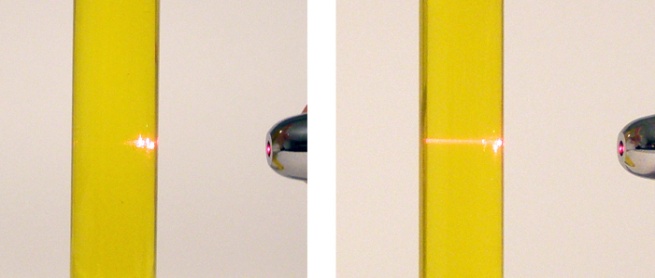 | Figure 2. a) Immediately after dissolving the SO2 the solution is clear and does not scatter the laser light (true solution); b) After 4–5 months the solution becomes hazy and heterogeneous disperse system has formed (the light of the laser pointer is scattered) |
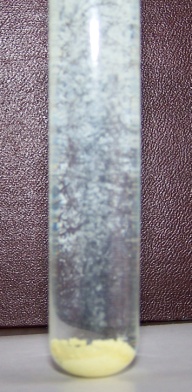 | Figure 3. Left test tube: after another two and a half months the solution becomes colorless and clear and the sulfur completely precipitates at the bottom (see a better photo at the far right). No changes occur in the right test tube (the blank) containing only SO2(aq) |
It was assumed at this point that the dissolved KI (or, the I− ions) acts as a catalyst. Since the system is (at the beginning) homogeneous, one is tempted to look for a feasible chemical explanation for the role of the catalyst and the probable chemical reactions it takes part in.It seemed reasonable that the processes taking place in the sealed ampoule containing KI can be described by the chemical equations: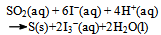 | (3) |
 | (4) |
or, due to suppressed second ionization of H2SO4: | (4a) |
If equations (3) and (4) are summed (2 × eq. 3 + eq. 4), one gets: | (5) |
or, due to suppressed second ionization of H2SO4: | (5a) |
Obviously the summary equation says nothing about the role of the catalyst. It is also incapable of explaining the entire chemistry in this system: if it were valid, then identical changes should occur in the system not containing KI, but they do not.Is elemental iodine (I2) really formed, or it is just “virtual”? Isn’t it possible that the yellow color is partly due to the iodine (the color being slightly different from that of the aqueous solution of iodine, perhaps because it is masked by the pale yellow color of the above mentioned clathrates)?To answer these questions we performed the experiment once again, exactly as it was described except that some starch “solution” was added to the distilled water. Not even traces of a blue color appeared as it would if I2 had actually been present at a reasonable concentration! Both the iodine (intermediary product, written as I3− due to the excess of iodide) and the corresponding reactions leading to its formation and consumption should be treated as “virtual”. In other words, the iodine is consumed at the very instant of its formation. The word “virtual” here is used much in the same sense as it is used in particle physics[7].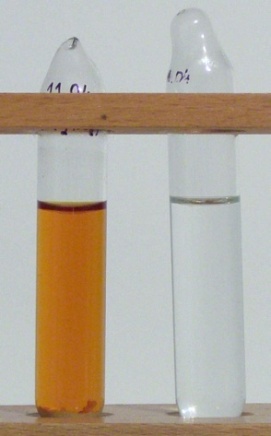 | Figure 4. After the ampoules were opened for a short period and sealed again, the color of the solution in the left ampoule becomes brown as a result of the formed elemental iodine, i.e. the complex I3−(aq) (due to oxidation of I− by air oxygen) |
Finally, when the ampoule is broken and part of the liquid is used, the remaining liquid in the ampoule soon turns brown (cf. Fig. 4), due to the chemical changes giving rise to “real iodine” (unlike the virtual one) that could be described by the following equation: | (6) |
4. Literature Checks, Conclusions and Decisive Experiments
In order to possibly make few additional checks for the above-offered explanation (whether one indeed deals with virtual reactions and virtual substances), a literature search on redox potentials was consulted[8]. The values for the following half-reactions seemed to be relevant: | (7) |
 | (8) |
 | (9) |
A few things now become obvious:a) The spontaneous disproportionation reaction of the aqueous solution of SO2 is definitely a feasible one (cf. the values of the redox potentials of eqs. 7 and 8).b) Despite the previous conclusion, the uncatalyzed reaction does not seem to proceed at any measurable rate.c) The redox potential for the iodine–iodide pair (i.e. the I3−
I−) equilibrium is just 30 mV higher than the oxidation potential of SO2. The role of the iodide as a catalyst is most probably to transport electrons necessary for the process (cf. eqs. 8 and 9). This might perhaps be achieved as a result of energy fluctuations from the ‘thermal bath’ (the aqueous solution). The latter could occasionally overcome the barrier equivalent to 30 mV. Let us mention in passing that the energy shortage corresponding to 30 mV is less than the mean-square translational energy of ideal gas at ≈ 300 K.d) Our electrochemistry expert, Mirčeski[9] turned our attention to yet another possibility. He pointed to the fact that, owing to the size of the iodide ion, it may serve as a centre for adsorption of different chemical species; in our case most probably SO2 molecules. These weakly bound structures might take the role of intermediary compounds often met during processes of homogeneous catalyses. The reduction of SO2 to S in the presence of catalyst, we propose, might occur through formation of such intermediary compounds of SO2 adsorbed on I−. e) Once the iodide anions transfer their charge (i.e. the electrons) onto the adsorbed SO2, the formed virtual iodine is immediately consumed by the excess SO2 (this is, in a way, analogous to saying that the catalyst has lowered the barrier posed by the activation energy of the process). SO2 is instantaneously back-oxidized by the virtual iodine to HSO4− and the iodide is restored. The former reaction (oxidation of iodide) is expected to be further catalyzed if the concentration of H+(aq) is increased (following the simple logic of Le Chattelier as applied to eqs. 3 or 8).With the latter idea in mind (i.e. that the H+ ions are expected to further catalyze the limitation step of the process) the experiment was repeated, but now the solutions also contained about 1 mL of 1 mol/L HCl(aq). Again, no changes could be registered in the blank. However, the ampoule containing potassium iodide and SO2(aq) hazes after 2 days and the reaction is finished (there is a complete loss of color and rich sulfur precipitate) in a total of 6 days!Finally, what should one expect if KI is substituted by KBr? It is known that the redox potential for the bromine–bromide equilibrium is | (10) |
and one notes immediately that now the energy barrier (or energy shortage) is proportional to 590 mV. That is, the barrier is now almost 20 times higher and the energy of the molecules from the ‘thermal bath’ is much too low. Furthermore, the radius of the bromide ion is significantly smaller than that of the iodide, and its adsorption properties are much less pronounced. One would say that, given the explanation for the I−(aq) catalyst is correct, bromide anions will not be capable to catalyze the disproportionation reaction. An experiment was set in an ampoule containing SO2(aq), HCl(aq) and KBr(aq). There was no evidence of a chemical reaction, not even after many months. It seems obvious that bromide anions are not capable of serving as a catalyst and the latter finding further strengthens the above offered explanations for the iodide-catalyzed reaction.Let us mention in the end that chemical reactions describing disproportionation of sulfur(IV) are far from well-known not only in the chemistry education literature, but also in the chemistry literature in general. The only source that we are familiar with is the work by Kubas[10]. The system described here is, however, much simpler from a chemical viewpoint.
5. Safety Issues
Sulfur dioxide is toxic in high concentration and is a severe respiratory irritant at lower concentration. The typical exposure limit is 2 parts per million (ppm), a level which can readily be exceeded in confined spaces such as a laboratory if ventilation is poor. Some people, especially those prone to asthma, may be especially sensitive to sulfur dioxide. In the presence of moisture, sulfur dioxide forms an acidic, corrosive solution; contact may lead to burns to the skin or eyes. In case of eye or skin contact immediately wash with plenty of water. If inhaled, call for immediate medical help if the patient shows any signs of distress, or has a history of respiratory problems. The gas generation is best to be done in a hood. Acids, in general, are extremely corrosive agents, which can provoke metal’s corrosion and tissue damage. Furthermore, their possible volatility and oxidation ability can enhanced the process of corrosion. Sulfuric acid is one of the strongest oxidizing and dehydrating substances in the chemical laboratory, yet used in so many experiments. Its vapor can be toxic, inflammable and, even, explosive. Contact of sulfuric and hydrochloric acid with the eyes or skin can cause serious permanent damage. Always wear safety glasses. Wear safety gloves if handling the concentrated acid. In case of eye contact immediately rinse the eye with plenty of water for few minutes. If the acid is spilled on the skin wash the area with plenty of water and remove any contaminated clothing. If ingested, drink plenty of water and call for immediate medical help. Do not induce vomiting. All solutions and compounds should be disposed off properly.
ACKNOWLEDGEMENTS
The authors express their sincere thanks to Prof. Valentin Mirčeski (for his interest in the work, for the useful suggestions he made and for critical reading of the manuscript).
References
[1] | B. V. Njekrasov, A Course in General Chemistry, 6th ed. Belgrade, Yugoslavia: Naučna knjiga, 1976, pp. 227–228 (translation from Russian into Serbian). |
[2] | N. N. Greenwood, A. Earnshaw, Chemistry of the Elements, 2nd ed. Oxford, UK: Butterworth-Heineman, 1998; pp. 700–701. |
[3] | C. Chambers, A. K. Holliday, Modern Inorganic Chemistry, London, UK: Butterworth & Co., 1975; p. 290. |
[4] | M. Najdoski, V. M. Petruševski, The Experiment in the Teaching of Chemistry, Skopje, Macedonia: Magor, 2002, pp. 289–291. |
[5] | Bukleski, M., Petruševski, V. M. 2009, Preparation and Properties of a Spectacular Themochromic Solid., J. Chem. Educ., 86(1), 30. |
[6] | G. Fowles, Lecture Experiments in Chemistry, Fifth, Revised & Enlarged Edition, London, UK: G. Bell & Sons, 1959; p. 3. |
[7] | J. Trefil, From Atoms to Quarks, New York, USA: Anchor Books/Doubleday, 1994; pp. 47–52. |
[8] | http://en.wikipedia.org/wiki/Table_of_standard_electrode_potentials (last checked January, 2013). |
[9] | V. Mirčeski, Private communication. |
[10] | Kubas, G. J. 1994, Chemical Transformations and Disproportionation of Sulfur Dioxide on Transition Metal Complexes., Acc. Chem. Res., 27(7), 183–190. |