Gbenga Matthew Ayininuola, Peter Oluseyi Oladotun
Department of Civil Engineering, University of Ibadan, Ibadan, Nigeria
Correspondence to: Gbenga Matthew Ayininuola, Department of Civil Engineering, University of Ibadan, Ibadan, Nigeria.
Email: |  |
Copyright © 2016 Scientific & Academic Publishing. All Rights Reserved.
This work is licensed under the Creative Commons Attribution International License (CC BY).
http://creativecommons.org/licenses/by/4.0/

Abstract
Soil encountered in many sites are either sound enough to withstand designed load from proposed structures to be erected upon them or there may be need to have the soil modified. One major method of enhancing soil strength capacity is to reinforce the soil with natural or artificial fibers. Coconut coir fiber was used as an additive material to improve the strength properties of soils such as the maximum dry density, the cohesion, angle of internal friction and the California bearing ratio CBR. Three different soil samples were sourced and mixed with different percentage proportions of coconut fiber (0.1%, 0.3%, 0.5%, 0.7%, 0.9%, 1.0%, 1.2% and 1.5%). Results of the experimental study revealed that all the soil parameters tested attained highest values at 1.2% coconut coir fiber addition. Addition of about 1.2% coconut fiber to the soil will enhance its strength.
Keywords:
Coconut coir fiber, Soil reinforcement, Soil shear strength, Geotechnical properties
Cite this paper: Gbenga Matthew Ayininuola, Peter Oluseyi Oladotun, Geotechnical Properties of Coconut Coir Fiber Soil Mixture, Journal of Civil Engineering Research, Vol. 6 No. 4, 2016, pp. 79-85. doi: 10.5923/j.jce.20160604.01.
1. Introduction
Stabilization is a process of improving subsoil engineering properties prior to construction. This can be accomplished in several ways such as preloading of the grounds involving application of high energy impacts, use of sand drains, prefabricated wick drains and chemical additives [1]. Reinforcement consists of incorporating certain materials with some desired properties within other material which lacks those properties [2]. Therefore, soil reinforcement is defined as a technique adopted to improve the engineering characteristics of soil in order to improve the soil properties such as shear strength, compressibility, density; and hydraulic conductivity [3]. Consequently, reinforced earth is a composite material consisting of alternating layers of compacted backfill and man-made reinforcing material [4]. The primary purpose of reinforcing soil mass is to improve its stability, increase its bearing capacity, and reduce settlements and lateral deformation [5].In ancient times, fiber was used for reinforcing soils. Early civilizations added straws and plant roots to soil bricks and cob wall to improve their properties although their mechanisms were not fully understood. However, modern geotechnical engineering has focused on the use of planar reinforcement. The reinforcing of soil with discrete fibers is still a relatively new technique in geotechnical projects [6].The concept of fiber-reinforcement in geotechnical projects originally involved the use of plant roots as reinforcement. Most researchers reported that plant roots increased the shear strength of the soil and the stability of natural slopes. With development of polymeric fiber since the late 1980s, triaxial compression tests, unconfined compression tests and direct shear tests have been conducted to study the effect of synthetic fiber-reinforcement on shear strength. Additionally, fiber-reinforcement previously was used to improve the road structure. Fiber reinforced subgrade (coir fiber and synthetic fiber) had notable influence on unconfined compression strength of silty sand [6]. Previous research has shown that fiber-reinforcement could increase the peak shear strength and limited post peak reductions in shear resistance and decreased the stiffness of the soil. Most of the experimental studies were conducted using granular soils. The mechanisms of fiber-reinforcement on dry sand reinforced with different types of fiber by using direct shear tests have been studied [7]. Fibers were placed at different specific orientations with respect to the shear plane. The fiber content, orientation of fibers, and modulus of fibers were found to influence the contribution of fibers to the shear strength development. The behavior of sand reinforced with discrete randomly oriented inclusions among other factors on particle shape and size of the sand has been investigated [8]. The effect of fiber-reinforcement was found to be more significant in fine sand with sub rounded particles than in medium grained sand with sub angular particles. The extensibility of the fibers was also found to influence the soil-fiber interaction. Fiber-reinforcement was reported to increase the shear strength of cohesive soils. The effect of two different forms of reinforcement such as coir mat and coir fiber as reinforcement in sand was explored [9]. Direct shear results with coir mat of different size openings and coir fiber of different lengths and at different percentages were added to determine the effect of their inclusion on shear strength of sand. It was found that the coir mat yielded higher value of angle of internal friction when compared with coir fiber. The use of waste fiber materials such as scrap tire rubber, polyethylene, and polypropylene fiber for the modification of clayey soils under unconfined compression, shear box, and resonant frequency tests was evaluated [10]. Waste fibers improved the strength properties and dynamic behaviour of clayey soils. Some researchers have studied the use of fibers to improve the ductility of cement-stabilized soils. Fiber-reinforcement increased the peak and residual shear strength of cement-treated soil, and changed their brittle behavior to ductile behavior was reported [11]. Behavior observed when using fibers with soils stabilized with cement or fly ash have been examined [12].The results of compaction tests on silty clay soil specimens reinforced with fibers were reported [13]. They concluded that the presence of fibers caused decrease in the density of soil. Unlike the case of sandy gravel, the test results showed that increment in the fiber content caused a modest increase in the maximum dry unit weight. The optimum moisture content was found to decrease with increasing in fiber content. The results of compaction test on palm fiber reinforced silty sand shown that the maximum dry density decreased and optimum moisture content increased with increasing in fiber content [14]. So far not much work has been reported on the use of coconut coir fiber. The present study is set out to investigate the behavior of coconut coir fiber in soil visa-vis its geotechnical properties.
2. Materials and Methodology
Soil samples were collected from three different locations namely: Location I- Academy area, Iwo road with Latitude 7°24’19.316”N and Longitude 3°56’47.229”N, Location O- Olorunda Abaa area with Latitude 7°28’05.861”N and Longitude 3°58’42.888”N and Location M- Mokola area with Latitude 7°24'43.931"N and Longitude 3°53'28.361"E in Ibadan, Nigeria as shown in Figure 1. The soil samples were air dried and stock-piled for laboratory work. Coconut were bought from coconut vendor in Ibadan, processed so that the fibers were extracted manually and separated into strands of about 4cm long (Figure 2).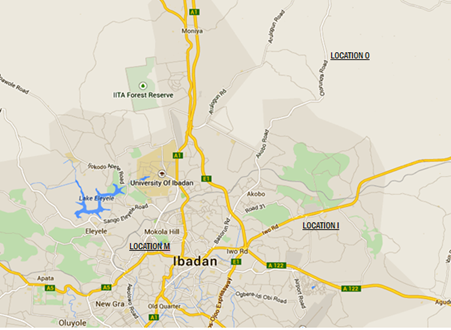 | Figure 1. Map showing the 3 samples locations I, M and O. Source: Google Earth |
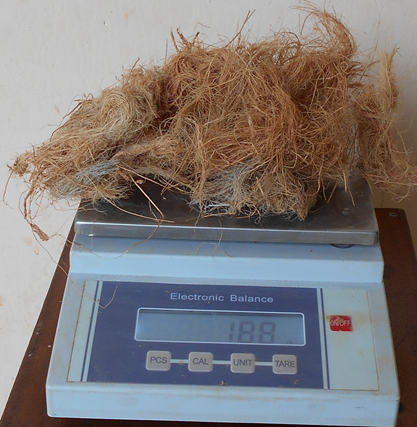 | Figure 2. Weighing the fiber to be compacted with the soil |
This particle size analysis was performed to determine the percentages of different grain sizes contained within each soil sample. The sieve analysis was performed to determine the distribution of the coarser, larger-sized particles in the soil, while the hydrometer method was used to determine the distribution of the fine particles in the soil samples. The test was carried out in accordance with standard [15]. Further laboratory test was carried out to determine the plastic and liquid limits of the soil samples. The tests were carried out in accordance with standard [16]. Compaction test was carried out to determine the optimum moisture content at which the maximum dry unit weight was attained for all the three soil samples using standard Proctor apparatus. In addition, both the California bearing ratio and shear strength test were carried out on the soil samples with sample specimens for the tests prepared based on the outcome of the compaction test results. Varying percentages of coconut fiber (0.1%, 0.3%, 0.5%, 0.7%, 0.9%, 1.0%, 1.2% and 1.5%) by weight were mixed with each soil sample separately as shown in Figure 2. Altogether aside control samples, twenty four samples of various percentages of coir coconut fiber were prepared. The samples geotechnical properties such as optimum moisture content, maximum dry density, CBR and shear strength parameters were determined following the procedures used to measure the control samples’ geotechnical properties determination. All experiments carried out were done with four replicates.
3. Results and Discussion
All control tests were tests conducted on samplies with 0% of fiber. Sample obtained from Academy Area, Iwo road was represented as I, Mokola Area represented as M and Olorunda Abaa Area represented as O. Particle size analysis test, Atterberg limits test, California bearing ratio (CBR), compaction test and triaxial test were performed in accordance with ASTM Standards as shown in Figures 3 to 5. Sieve analysis tests were performed on control samples I, M and O.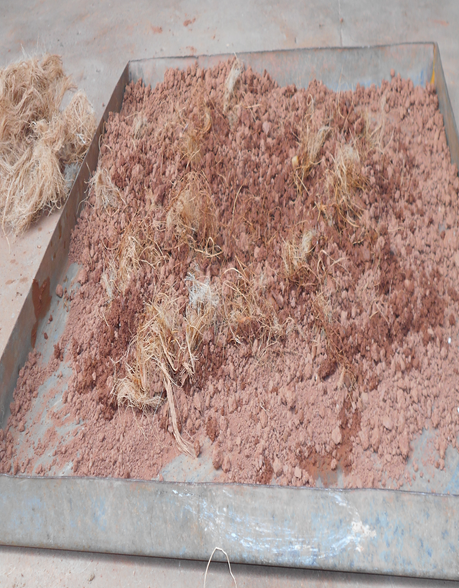 | Figure 3. Properly mixing the fiber-soil with water |
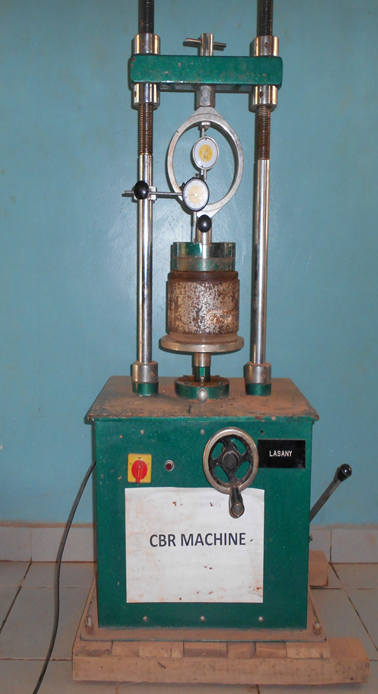 | Figure 4. CBR machine set-up |
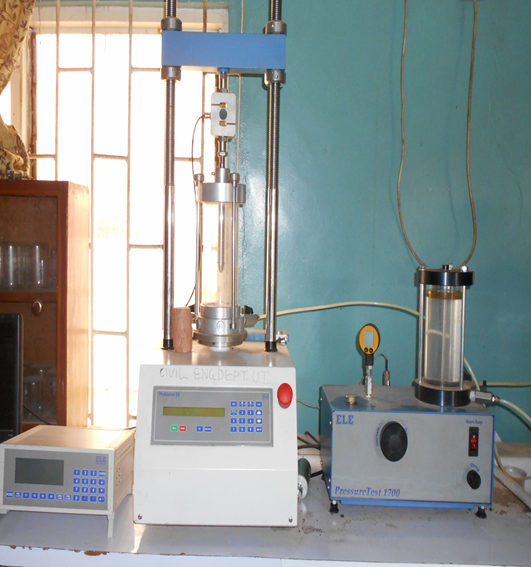 | Figure 5. Multiplex 50 set up for shear strength test |
According to Unified Soil Classification System, samples I and M were clayey sand (SC) while sample O was poorly graded sand with clay (SP-SC). The coefficients of uniformity, Cu of control samples I, M and O were 200, 100, 120 respectively and coefficient of curvature, Cc of control samples I, M and O were 16, 30 and 23 respectively. Figure 6 shows grading curves of all three control samples.The liquid limit (LL), plastic limit (PL) and plasticity index (PI) of control samples I, M and O were 43%, 18%, 25; 43%, 27%, 16; and 40%, 21%, 19 respectively. Table 1 and Figure 7 display the maximum dry density (MDD) pattern of the three soil samples when mixed with different percentages of coconut coir fiber (CCF). It was observed that sample I has MDD of 1.792 g/cm3 at 0% CCF inclusion, increased to 1.863 g/cm3 at 1.2% CCF and decreased to 1.801 g/cm3 at 1.5% CCF addition. Likewise, sample M has MDD value of 1.904 g/cm3 at 0% CCF addition, increased to 2.243 g/cm3 at 1.2% and decreased to 1.982 g/cm3 at 1.5% CCF percentage. Sample O has a MDD value of 1.664 g/cm3 at 0% CCF, increased to 1.916 g/cm3 at 1.2% CCF percentage and decreased to 1.877 g/cm3 at 1.5% CCF percentage. The three soil samples attained their maximum dry density at 1.2% CCF. The increase in the dry density observed was as a result of coconut fiber content added to fill up the interlocking spaces within the soil matric and held the particles together. Also, Table 1 contains the values of the optimum moisture content (OMC) of the three soil samples measured at different percentages of CCF addition. It was noticed that the OMC increased as CCF increased from 0% to 1.5%. The CCF is hygroscopic in nature and possesses high tendency to absorb water. Sample I has the highest OMC while sample O has the lowest OMC.Table 1. Shear strength test results 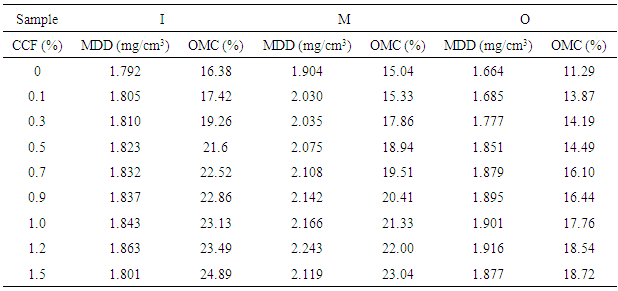 |
| |
|
The laboratory test carried indicated that the CBR values of soil samples I, M and O increased as the fiber percentages increased from 0% to 1.2% and experienced reduction thereafter as shown in Figure 8. Soil sample I has the CBR values of 31.5%, 59.4% and 57.6% at 0%, 1.2% and 1.5% CCF respectively. On the other hand, the corresponding values for soil sample M were 32.8%, 60.9% and 60.6%. Sample O has the CBR values of 30.2%, 58.2% and 57.9% at 0%, 1.2% and 1.5% CCF respectively.The presence of CCF in the right proportion up to 1.2% held the soil particles together. The CCF increased the resistance of plunger of the CBR machine penetration that invariably led to increase in soil CBR. All the soil samples attained peak values of CBR at 1.2% CCF. This shows that there is limit quantity of CCF that each soil needs to hold it particles together. Beyond this peak value, excess CCF present will form weak bond that creates ease passage for cone plunger to penetrate into the soil mass. This action will lead to reduction in soil samples CBR.Cohesion values of the three soil samples increased as the fiber content increased from 0% to 1.2% and decreased as CCF increased from 1.2% to 1.5% as shown in Figure 9. Basically, sample I has cohesion values of 27.7 kN/m2, 29.5 kN/m2 and 29.4 kN/m2 at 0%, 1.2% and 1.5% CCF respectively. Sample M has cohesion values of 29.5 kN/m2, 30.3 kN/m2 and 30.1 kN/m2 for 0%, 1.2% and 1.5% CCF respectively. Sample O has cohesion values of 28.8 kN/m2, 29.7 kN/m2 and 29.5 kN/m2 for 0%, 1.2% and 1.5% CCF addition respectively as shown in Figure 7. All the three soil samples attained highest cohesion at 1.2% CCF which coincided with that of CBR. The two geotechnical properties are tools used to determine soil ability to resist external loading.The internal friction angle of soil samples I, M and O measured using triaxial machine increased until the percentage of CCF added to the soil reached 1.2% and beyond this value, the angle of friction declined. The peak angle of friction was reached at 1.2% CCF which is similar to the pattern obtained for soil cohesion as detailed in Table 1 and Figure 8. The reduction in the soil internal angle of friction and cohesion as a result of increasing CCF content beyond 1.2% could be attributed to large quantum of fiber present in the soil. The excess CCF increased the void present as well as soil water content since CCF has strong tendency to absorb water. The excess void and water content would reduce forces of attraction among soil particles and also the cohesive forces present in the soil matrix. 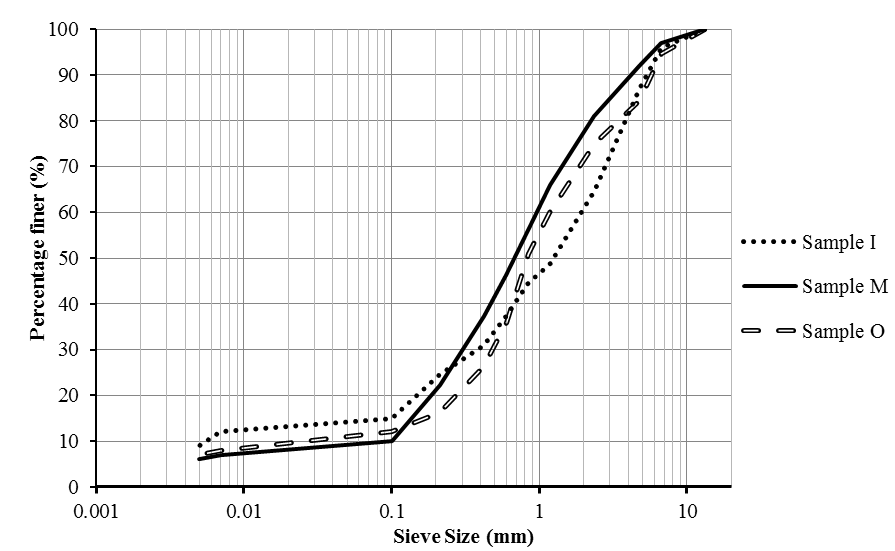 | Figure 6. Particle distribution curve of the three soil samples |
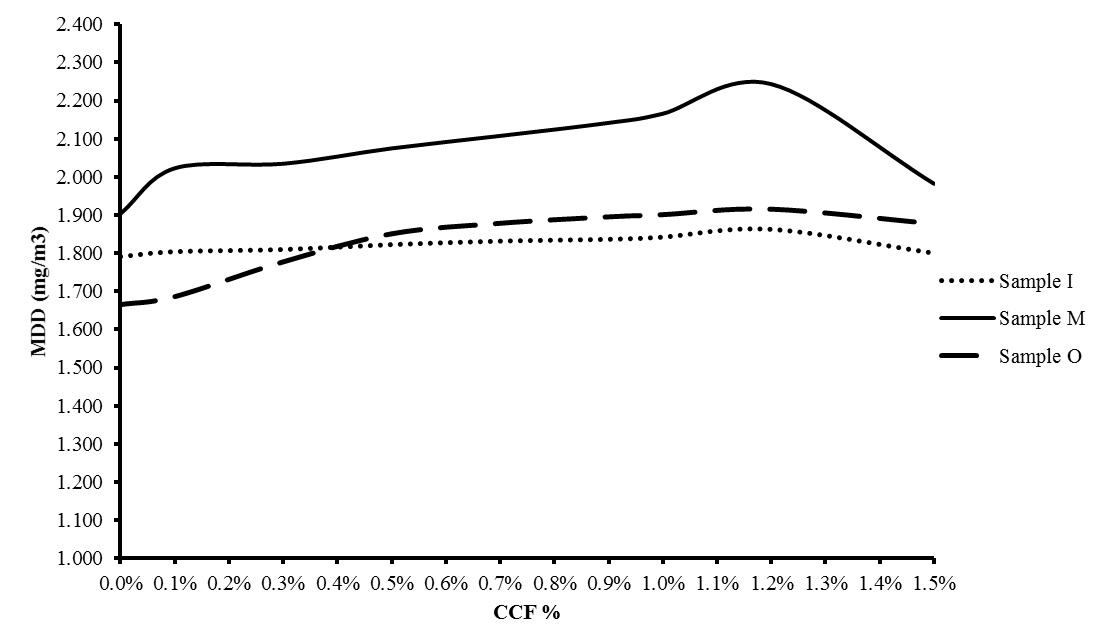 | Figure 7. Curve of soil samples maximum dry density against different percentages of coconut coir fiber |
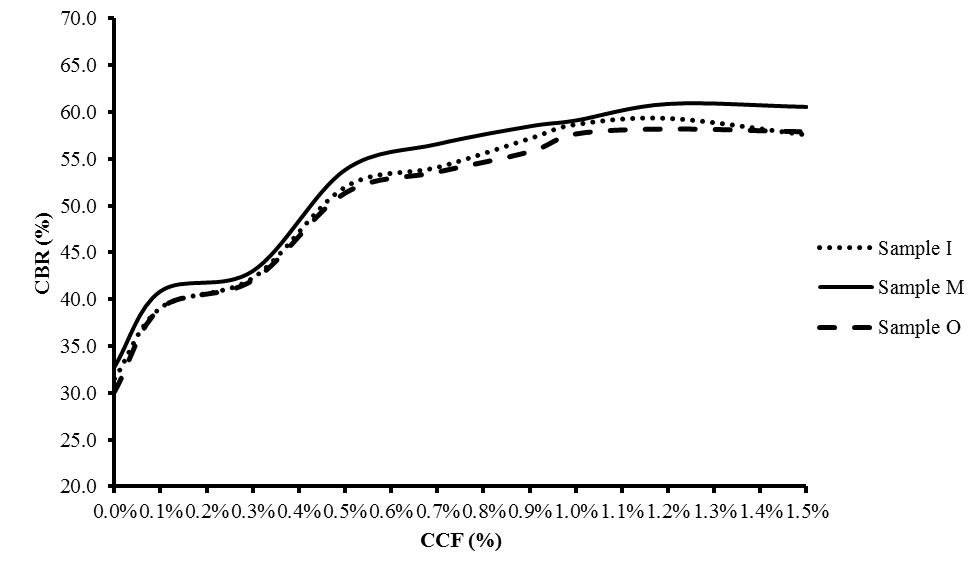 | Figure 8. CBR values of Samples I, M and O at different fiber percentages |
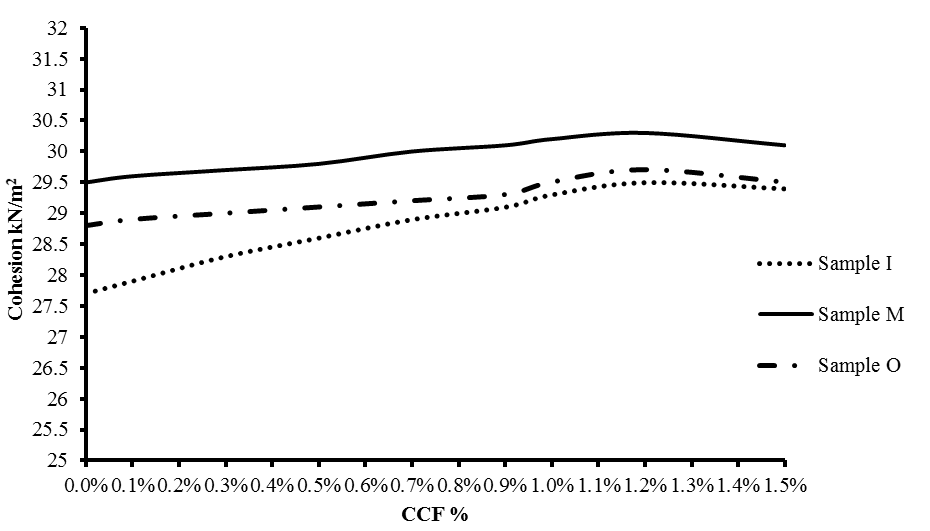 | Figure 9. Cohesion values of the 3 samples at different fiber percentages |
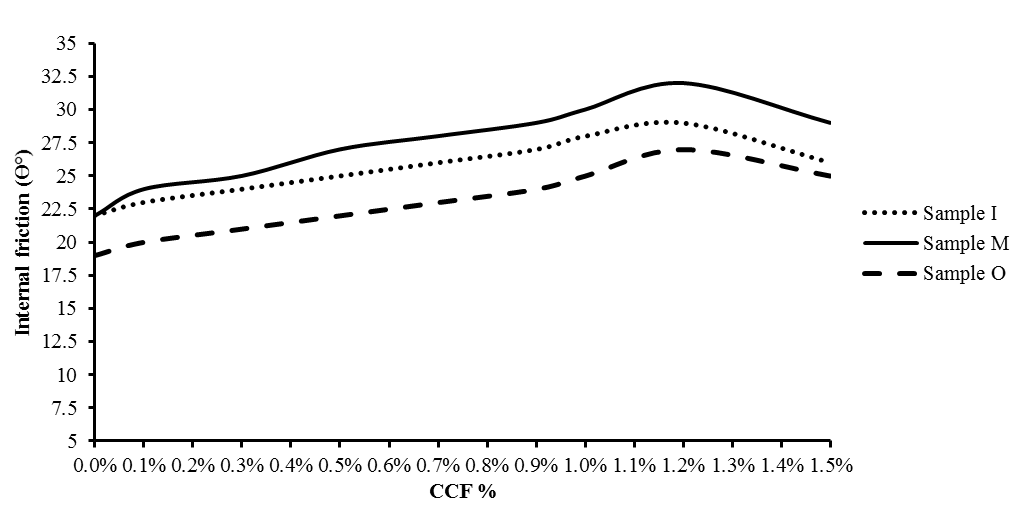 | Figure 10. The different internal friction angles of Samples I, M and O at different percentages of CCF |
4. Conclusions
The in-depth experimental study revealed that the soil samples geotechnical parameters reached peak values when the percentage of coir coconut fiber added reached 1.2%. This showed that enough quantity of fiber is in the soil to hold its particles together to supplement the resistance of soil against external load.
ACKNOWLEDGEMENTS
The authors wish to express our profound gratitude to the members of staff of the soil laboratory of the Department of Civil Engineering University of Ibadan. They rendered high level of assistance during the experimental set up of the research.
References
[1] | Aziz M.A. 2013. A new method of stabilization of soft soils. First international conference on case histories in geotechnical engineering. Missouri University of Science and Technology. |
[2] | Jones M. 1999, Mechanics of composite materials. Taylor and Francis. 2nd Ed. |
[3] | Kazemian S., Huat K., Prasad A. and Barghchi M. 2010. A review of stabilization of soft soils by injection of chemical grouting. Journal of Basic and Applied Science, 4(12):5862 - 5868. |
[4] | Ola, A., 1989, Stabilization of lateritic soils by extensible fiber reinforcement. Engineering Geology. 26: 125-40. |
[5] | Binici, H., Aksogan, O., and Shah, T., 2005, .Investigation of reinforced mud brick as a building material. Construction Building Material. 19:313 - 318. |
[6] | Farshid, B., 2009, Stabilization mechanisms of oil palm empty fruit bunch (OPEFB) fiber reinforced silty sand. Ministry of Science, Technology and Innovation (MOSTI), Malaysia. |
[7] | Gray, D. H., and Ohashi, H., 1983, Mechanics of fiber reinforcing in sand. Journal of Geotechnical Engineering, ASCE, 109(3): 335 - 353. |
[8] | Al-Refeai, T .O., 1991, Behavior of granular soils reinforced with discrete randomly oriented inclusions. Geotextiles and Geomembranes, 10(4): 319 – 333. |
[9] | Kumar, M. T. P., and Shridhar, R., 2013, Effect of coir mat and coir fiber reinforcement on shear strength of dry sand. Proceedings of Indian Geotechnical Conference, Roorkee. |
[10] | Akbulut, S., Arasan, S., and Kalkan, E., 2007, Modification of clayey soils using scrap tire rubber and synthetic fibers. Applied Clay Science, 38: 23 – 32. |
[11] | Consoli, N., Heineck, K., Casagrande, M., and Coop, M., 2007, Shear strength behavior of fiber-reinforced sand considering triaxial tests under distinct stress paths. Journal Geotechnical Geoenvironmental Engineering, 133(11): 1466 - 1469. |
[12] | Kaniraj, S. R., and Havanagi, V. G. 2001, Behavior of cement-stabilized fiber-reinforced fly ash-soil mixtures. Journal Geotechnical and Geoenvironmental Engineering, 127(7): 574 - 584. |
[13] | Fletcher, C., and Humphries, W., 1991, California bearing ratio improvement of remoulded soils by the addition of polypropylene fiber reinforcement. Transportation Research Record 1295, Washington, DC, USA, 80-86. |
[14] | Marandi, S., Bagheripour, M., Rahgozar, R., and Zare H., 2008, Strength and ductility of randomly distributed palm fibers reinforced silty-sand soils. American Journal of Applied Sciences 5 (3): 209-220. |
[15] | ASTM, D422 2000, Standard test methods for particle-size analysis of soils. ASTM Standard. |
[16] | ASTM, D4318. 2000, Standard test method for liquid limit, plastic limit, and plasticity index of soils. ASTM standards. |