Gbenga Matthew Ayininuola, Idowu Olumuyiwa Ayodeji
Department of Civil Engineering, University of Ibadan, Ibadan, Nigeria
Correspondence to: Gbenga Matthew Ayininuola, Department of Civil Engineering, University of Ibadan, Ibadan, Nigeria.
Email: |  |
Copyright © 2016 Scientific & Academic Publishing. All Rights Reserved.
This work is licensed under the Creative Commons Attribution International License (CC BY).
http://creativecommons.org/licenses/by/4.0/

Abstract
The water treatment process generates sludge that must be disposed of in an environmentally friendly manner. This research presented the use of sludge ash as a soil stabilizing agent to serve as an alternative means of disposing sludge. The raw sludge was obtained from the University of Ibadan water treatment plant, burned at 1120°C and grinded to powder. The sludge ash of various proportions (0%, 3%, 5%, 7%, 10% and 15%) by weight was mixed separately with three soil samples obtained within the University of Ibadan. The geotechnical properties of the soil samples such as particle size analysis, Atterberg limits, compaction test and triaxial test were monitored. The results revealed that all the samples attained least optimum moisture content, maximum dry density and highest shear strength at 7% stabilization. Beyond this percentage, the dry density and shear strength depreciated. The ash in moist medium reacted with soil to form Cementous compound that acts as binding agent to improve soil particles bonding and its strength. In excess, the ash contributed to the soil void and caused reduction in its strength.
Keywords:
Sludge ash, Soil stabilization, Shear strength, Geotechnical properties
Cite this paper: Gbenga Matthew Ayininuola, Idowu Olumuyiwa Ayodeji, Influence of Sludge Ash on Soil Shear Strength, Journal of Civil Engineering Research, Vol. 6 No. 3, 2016, pp. 72-77. doi: 10.5923/j.jce.20160603.04.
1. Introduction
The study of engineering behaviour of soil is extremely important to civil engineers because every engineering structure such as building, road, bridge, monument, etc. will have to be rested and founded on the ground. The sub structure or the foundation portion of every civil engineering structure will have to be taken below the ground and rested on the foundation soil, in such a manner that the structure does not get tilted or settled, or damaged throughout its life time. The strength of the soil to withstand loads, under different site conditions, therefore becomes an important factor. When a road or railway embankment is to be constructed on unsound soil, some form of improvement is usually needed to avoid settlement and stability problems. Soil stabilization is the permanent physical and chemical alteration of soils to enhance their physical properties. Stabilization can increase the shear strength of a soil and/or control the shrink-swell properties of a soil, thus improving the load-bearing capacity of a sub-grade to supports pavements and foundations. Stabilization can be used to treat a wide range of sub-grade materials from expansive clays to granular materials [1]. Soil stabilization has a long history. As early as 2000 years ago the Romans used lime to stabilize clay soils [2]. Since the late 1980s, the dominating binding agents have been combinations of lime and cement [3]. Cement and lime are binding agents. They bind and strengthen the soil without the need to add an activator [4]. Also, it is reported that by applying foamed bitumen it is possible to produce a semi-rigid layer of stabilized soil [5]. In stabilization soil by deep mixing, the binder, combination of cement and lime has been found to give good stabilization effectiveness [6]. Cement provides rapid, high and robust growth in strength. The slaking of the lime also provides momentary drying and heat evolution which accelerates the reaction of the cement. On the other hand, sludge is obtained from the processes of chemical coagulation and softening at water treatments plants and from the unstable organic nature that readily undergoes active microbial decomposition with consequent generation of nuisance odors. Sludge has common characteristic of high water content, usually greater than 95% by weight. It can also be said to be an inescapable by-product of water treatment processes. Sludge typically contains mineral and humic matters removed and precipitated from the raw water, together with the residues of any treatment chemicals used as coagulant (commonly aluminium or iron salts) and coagulant aids (mostly organic polymers). In the practical context, alum sludge and ferric sludge refer respectively to the sludge generated when aluminium or iron salt is used as the coagulant.Available literature estimates that whopping sum of 10000t of waterworks sludge is produced daily [7]. Several million tons of waterworks sludge is produced every year and this may be doubled by next decade raising considerable concerns over their disposal and associated costs [8]. Oftentimes, the cost of handling the enormous quantities of waterworks sludge can account for a significant part of the overall operating costs of water treatment works which is likely to increase due to increasingly stringent regulations. While considerable development is been made, waterworks sludge are sometimes discharged into nearest drainage course or water body and promptly forgotten. Amongst possible explanation for this earlier line of thought could be the fact that waterworks sludge is regarded as inorganic even though they have some organic content, albeit somehow very low, and consequently may not exert any worrisome oxygen demand on water bodies. Therefore it is only a matter of time before the waterworks sludge issue becomes worrisome. The limited land available for waterworks sludge disposal and the possible environmental liabilities that may arise if disposed of in sanitary landfill sites, altogether makes it a considerable worry for water purification authorities.It has been advocated that waterworks sludge could be a potential recyclable product, offering one of the greatest commercial potential for reuse [9, 10]. In a review of sludge bricks, it was discovered that waterworks sludge especially ferric sludge provided some energy savings in brick making by acting as a fluxing agent [9]. In general, recycling of waterworks sludge in the cement industry can be a practical alternative as reported [11], in that the waterworks sludge is virtually non-hazardous, and the chemical composition of the inorganic sludge is similar to the clay used in cement production. The feasibility of sludge incorporation as a filler material in bituminous mixtures for use in general pavement works has been reported [12]. It was recommended that sludge should be thermally treated to at least a temperature of 450°C to volatize all the organic components. It has been discovered that waterworks sludge might be suitable for use as soil substitutes since it predominantly contains humic substances and sediments from the raw water, which makes it similar to fine textured soils [13].The investigation work on the effect of waterworks sludge application on four South African soils was carried out. The result showed that an application rate of between 0-1280Mg WTR ha and an incorporation depth of 0.20m, the saturated hydraulic conductivity of the soils was increased linearly with the application rate. Also, the total porosity increased due to a decrease in bulk density [14]. The use of alum sludge as raw material for ceramic products was also reported [15]. Properties of the alum sludge based fired bodies were comparable to similar products, offering a potential opportunity for recycling and reuse of alum sludge. This study presented another engineering means of recycling sludge as soil stabilizer.
2. Materials and Methodology
Three soil samples were collected from three different soil zones within the University of Ibadan, Ibadan, Nigeria. The collected soil samples were air dried and stock-piled throughout the tests duration as shown in Figure 1. Geotechnical properties of soil samples such as particle size analysis, Atterberg limits, compaction and triaxial tests were performed on the soil samples prior to addition of sludge ash in accordance with standard [16]. The results obtained were regarded as control samples’ results for the three soil samples labelled A, B and C. The raw sludge used was obtained from the University of Ibadan water treatment plant. The sludge was sun dried (Figure 2), burned in furnace at 1120oC for about 5 hours to whitish powder and grinded (Figures 3 and 4). The chemical properties of the sludge ash were determined at Hegada Scientific Services Limited, Ibadan, Nigeria. The percentages of oxides of silicon oxide (SiO), aluminium oxide (Al2O3), and iron oxide (Fe2O3) present in the sludge ash were determined using atomic absorption spectrometer.Each soil sample was subdivided into five parts. Each part was mixed with single proportion by weight of sludge ash. The sludge ash proportions investigated were 3%, 5%, 7%, 10% and 15%. The soil-ash mixture geotechnical properties such as compaction and shear strength tests were monitored. The compaction test was carried out using proctor machine in accordance with standard [16] as shown in Figure 5. The compaction test yielded soil optimum moisture content and its maximum dry density. These two parameters were utilized to prepared specimens for triaxial machine in order to determine soil sample consolidated undrained shear strength parameters: cohesion and angle of friction as spelt out in [16] as shown in Figure 6. All the soil samples for experiments (Atterberg limits, compaction test and triaxial shear strength) were prepared with four replicates.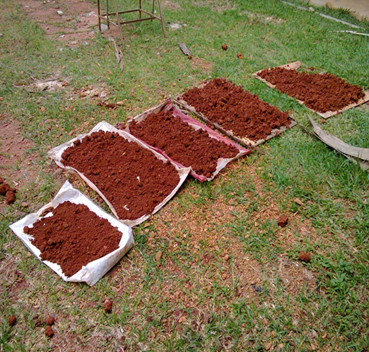 | Figure 1. Open air drying of soil samples |
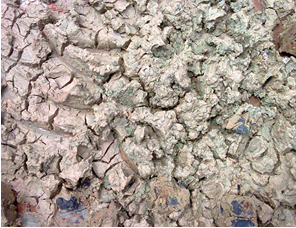 | Figure 2. Sun drying of raw sludge on sand bed |
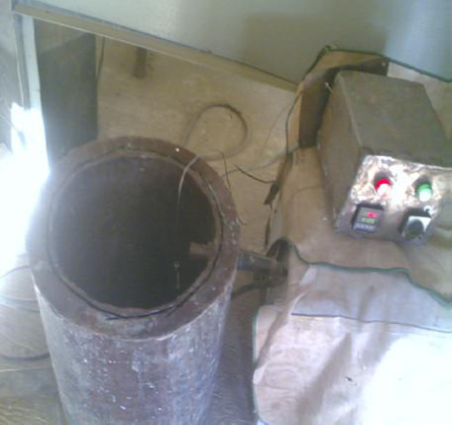 | Figure 3. Electric furnace for burning the sludge to whitish powder |
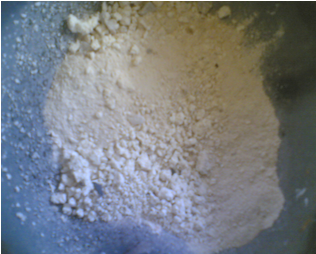 | Figure 4. Grinded whitish sludge ash |
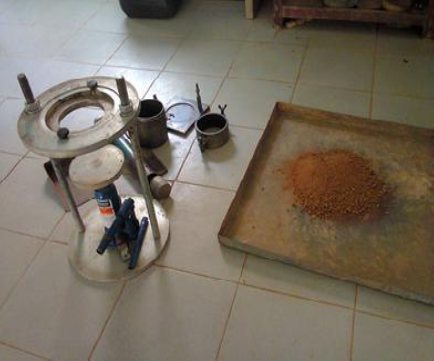 | Figure 5. Compaction test set up |
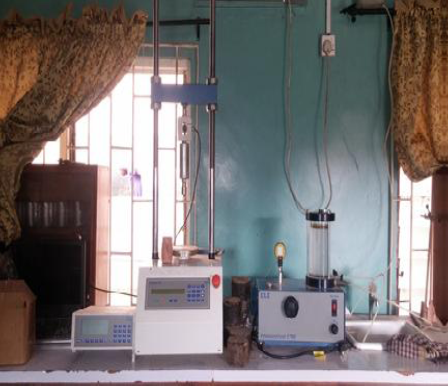 | Figure 6. Multiplex 50 set up for shear strength test |
3. Results and Discussion
The results of the chemical analysis of the sludge ash were summarized as follows: SiO2 (25.97%), Al2O3 (15.39%), CaO (0.921%) and Fe2O3 (0.714). The particle size analysis result of the three soil sample is shown in Figure 7. The soil samples liquid limit (LL), plastic limit (PL), plasticity index (PI) and soil natural moisture content (NMC) are displayed in Table 1. The results obtained from the Atterberg limits test revealed that sample A and B have more clay content than sample C, as evidenced by their PI values. Soil samples A and B were classified as sandy clay of high plasticity (CH) while sample C was categorized as well graded sand with silt (SW-SM) in accordance with the Unified Soil Classification System (USCS). 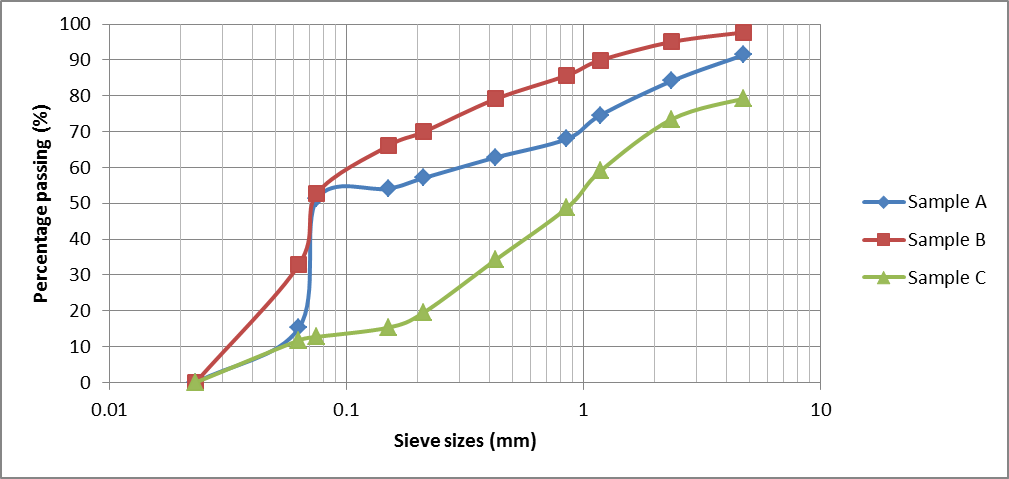 | Figure 7. Soil samples particle size analysis curves |
Table 1. Soil samples Atterberg’s limits 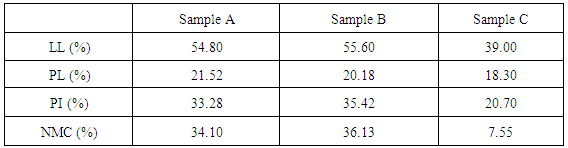 |
| |
|
The compact test was carried out to determine the optimum moisture content (OMC) and maximum dry density (MDD) of the samples when they were not stabilized and also when stabilized with sludge ash. The results are shown in Figures 8 and 9. The optimum moisture contents of the soil samples initially decreased as the ash content increased up to 7% stabilization. Thereafter, appreciable increase in OMC of all the samples was recorded. The initial decrease in OMC could be attributed to the absorption capacity of the sludge ash during the alkali aggregate reaction to enhance the bonding of particle together. At the lowest OMC point, the water needed for the reaction reached its maximum value and the right or required quantity of ash was present in the soil. Beyond this point of least OMC (Figure 8), further addition of ash would absolve water but only fill the void in the soil matrix since it is not reactive. The water absorbed would form part of the OMC. This accounts for further increase in OMC observed in Figure 9. The relationship between MDD of all samples with sludge ash is shown in Figure 9. It was observed that the MDD curves initially sloped upwards, indicating an increase in MDD with increase in sludge ash content. After attaining maximum values at 7% sludge ash stabilization, the maximum dry densities of the samples began to drop. The initial increase in the dry densities of the samples (0 to 7% stabilization) represented stage of effective alkali-aggregate reaction of ash with soil. The 7% stabilization was the state at which the right quantity of ash was present to maximize the alkali-aggregate reaction. Beyond this stage, the added ash only filled the cavity but not participating in the reaction that improves binding potential of the soil particles to form dense soil. 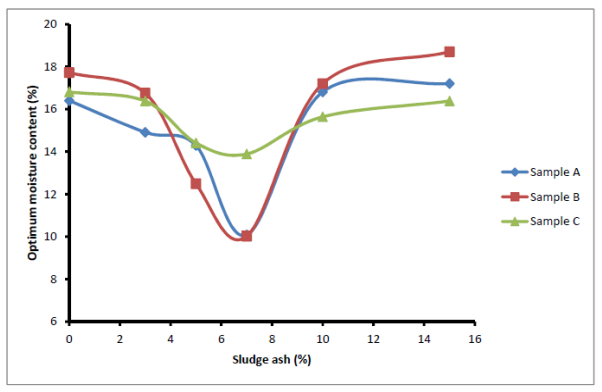 | Figure 8. Effect of sludge ash on soil optimum moisture content |
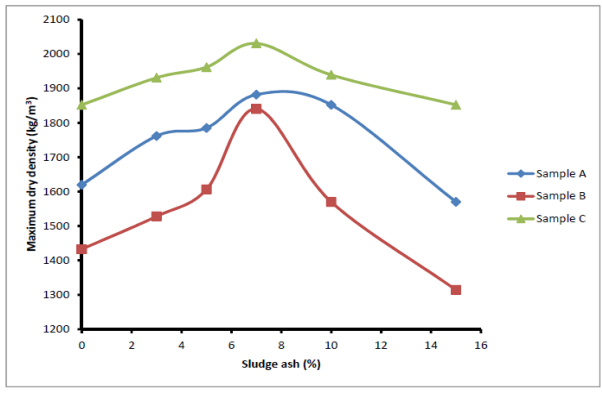 | Figure 9. Effects of sludge ash on maximum dry density |
Soil derives its strength from two sources: cohesion among particles and friction among particles. The values of cohesion and angle of friction obtained from Mohr circles were used to estimate soil shear strength and are shown in Table 2 and Figure 10. The values of shear strength increased as ash content increased and got to peak at 7% stabilization. Beyond 7% stabilization, the shear strength values declined. The alkali-aggregate reaction of ash with soil in presence of moisture was responsible for the increase in the bond among soil particles and also its shear strength. The maximum value of ash needed for the reaction was reached at 7% stabilization which was responsible for the peak in the values of shear strength obtained. The initial increase in the shear strength is expected because of the gradual formation of Cementous compounds between the soil and sludge ash in presence of moisture. The excess ash added beyond 7% only filled the void and brought about reduction in soil shear strength.Table 2. Shear strength test results 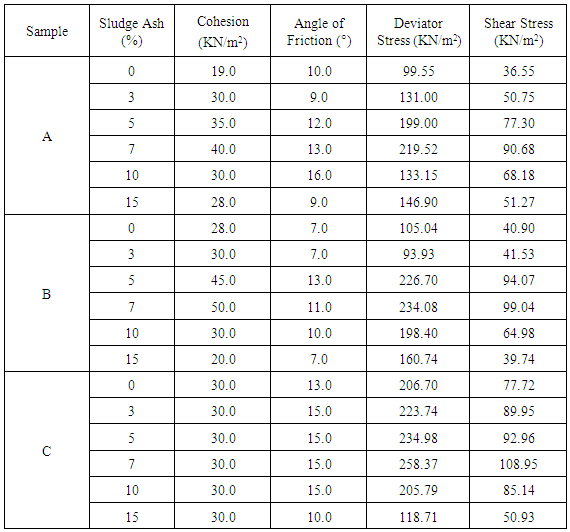 |
| |
|
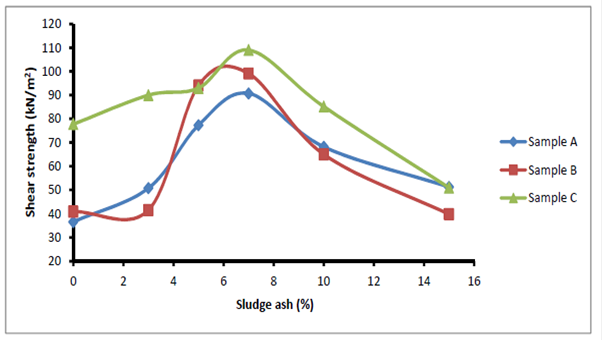 | Figure 10. Effects of Sludge ash on soil shear Strength |
4. Conclusions
The study investigated the influence of sludge ash on three soil samples. It was deduced that the optimum moisture contents of the soils attained lowest level at 7% ash content. Also, the soil samples maximum dry densities and shear strength reached highest values at 7% stabilization. The reduction in optimum moisture content and the increase in soil shear strength were as a result of pozzolanic nature of sludge ash in soil in the presence of moisture. The alkali aggregate reaction of ash with soil in moisture medium produced Cementous compound responsible for the increase in soil strength observed. Therefore, 7% ash obtained from sludge can be added to soil to enhance its strength.
ACKNOWLEDGEMENTS
The authors wish to express our profound gratitude to the management of the University of Ibadan Water Treatment Plant for the assistance rendered during the collection and subsequent processing of sludge to ash used for the research. Also, we wish to thank the laboratories staff of the University of Ibadan and Obafemi Awolowo University both in Nigeria for the help received during the experimental setup of the research.
References
[1] | Wikipedia, “Fly ash”, http://en.wikipedia.org/wiki/Fly_ash. Accessed 20 September 2013. |
[2] | P. Lindh, “Soil stabilization of fine-grained till soils - the effect of lime and hydraulic binders on strength and compaction properties’. Dissertation, Department of Geotechnology soil mechanics and foundation engineering, Lund University, 2000. |
[3] | H. Åhnberg, “Strength of stabilized soils – a laboratory study on clays and organic soils stabilized with different types of binder”, Dissertation, Department of construction sciences, Lund University, 2006. |
[4] | R. Andersson, K. Axelsson, and S. Johansson, “Stabilizing of organic soils by cement and puzzolanic reactions – feasibility study”, Svenk Djupstabilisering, Sweden, 2002. |
[5] | K. J. Jenkins, “Mix design considerations for cold and half-warm bituminous mixes with emphasis on foamed bitumen”, Dissertation, University of Stellenbosch, South Africa, 2002. |
[6] | Kujala, G and Ravaska, O., 1996. Settlement calculation of deep stabilized peat and clay, Proc. International conference on ground improvement geosystems, Tokyo 1: 551 – 555. |
[7] | H. B. Dharmappa, A. Hasia, and P. Hagare, “Water treatment plant residuals management”, Wat. Sci. & Tech. 35(8): 45 – 56, 1997. |
[8] | Bassibuyuk M, and Kalat, D.G., 2004, The use of waterworks sludge for the treatment of vegetable oil refinery industry waste water. J. Environ. Techno. 25(3): 373 – 380. |
[9] | P. Goldbold, K. Lewin, A. Graham, and P. Barker, “The potential reuse of water utility products as secondary commercial materials”, WRC Technical Report Series, No UC 6081, 2003. |
[10] | Rensburg V.L. and Morgenthal T.L., 2003, Evaluation of water treatment sludge for ameliorating acid mine waste. J. Environ.Qual. 32: 1658 – 1668. |
[11] | Pan, J. R, Huang, C. and Lin, S., 2004, Reuse of fresh water sludge in cement making. Wat. Sci. & Tech. 50(9): 183 – 188. |
[12] | Carvalho M, and Antas A 2005, Drinking water sludge as a resource. Proceedings of IWA specialized conference on management of residues emanating from water and wastewater treatment, Johannesburg, South Africa. |
[13] | Dayton, E.A., and Basta, N.T., 2001, Characterization of drinking water treatment residuals for use as a soil substitute. Water Environ. Res. 73 (1): 52 – 57. |
[14] | Moodley, J.M., and J.C. Hughes, “The effects of a polyacrylamide-derived water treatment residue on the hydraulic conductivity, water retention and evaporation of four contrasting South African soils and implications for land disposal”. Proceedings of IWA specialized conference on management of residues emanating from water and wastewater treatment, Johannesburg, South Africa Owen, 2005. |
[15] | Vicenzi J, Bernardes A.M., and Bergmann C.P., 2005, Evaluation of alum sludge as raw material for ceramic products. J. Industrial Ceramics 25(1): 7 – 16. |
[16] | British Standards 1377, 1998, Methods of test for Soils for civil engineering purposes. British Standards Institute, UK. |