Hasan Md Anamul , Jenifer Rahman , Mufrad Tanvir
Department of Civil and Environmental Engineering (CEE) Shahjalal University of Science and Technology, Sylhet, 3114, Bangladesh
Correspondence to: Hasan Md Anamul , Department of Civil and Environmental Engineering (CEE) Shahjalal University of Science and Technology, Sylhet, 3114, Bangladesh.
Email: |  |
Copyright © 2012 Scientific & Academic Publishing. All Rights Reserved.
Abstract
Municipal waste collection is included to solid waste management, involving recycling, incineration, dump etc. To minimize the hazards, incineration of medical waste is a significant alternative way for disposal of this category of waste. But relatively less attention has been given to bottom ash. Now bottom ash is dumped on the soil which mixes in to the soil as diffuse pollutant. In this study bottom ash samples were collected from a typical Municipal waste incinerator and typical pollutants including heavy metals (Zinc and Nickel) and nutrients (Calcium and Magnesium) concentration were examined by following standard extraction and detection procedure. In our test, the concentration of Zn (578mg/kg to 1108mg/kg), Ni (105 mg/kg to 410 mg/kg) exceeded the tolerable level of Zn (300mg/kg) and Ni (40mg/kg).The concentration of the nutrients Ca(435mg/kg to 754mg/kg) and Mg(372mg/kg to 1103mg/kg) were found. Pollution Load Index , Ecological Risk Index, Enrichment Factor and Geo-accumulation Index were applied to assess the level of heavy metal contamination. Co-relation factors of those heavy metals were also established. Ash samples were subjected to solidification/stabilization studies, by making blocks in different sand-ash ratios, using Ordinary Portland Cement (OPC) as binder. The Compressive strength of blocks is obtained through tests using American Standard Testing Machine (ASTM). The leaching characteristics of solidified ash in to block, were also examined by standard testing procedure. The ash can be recycled as construction material (fine aggregate), and can be used in low mortar strength applications, such as rings of pit latrines, pavement tiles for footpaths, wall tiles etc.
Keywords:
Bottom Ash, Diffuse Pollutant, Heavy Metal, Leaching, Compressive Strength, Nutrient
Cite this paper:
Hasan Md Anamul , Jenifer Rahman , Mufrad Tanvir , "Zn and Ni of Bottom Ash as a Potential Diffuse Pollutant and Their Application as “Fine Aggregate”", Journal of Civil Engineering Research, Vol. 2 No. 6, 2012, pp. 64-72. doi: 10.5923/j.jce.20120206.03.
1. Introduction
One of the medical waste treatments used worldwide is medical waste incineration. Unfortunately, incineration is not a clean process. Emissions of heavy metals and organic pollutants from these facilities cause significant environmental harm. The chief environmental contaminants of concern in the emissions are Zinc & Nickel. Certain conditions in the incineration — high temperature, organic matter, source of chlorine, metals tact as chemical catalysts. Although incineration can reduce the weight of waste by more than 70%, large amounts of combustion residues, especially bottom ash, still remained after incineration. In some densely populated big cities, disposal of the waste ash is becoming increasingly difficult, owing to high cost, diminishing land availability, more stringent regulation, and frequent public opposition to the sifting of new landfills. This type of material has the potential to be reused in the interests of achieving sustainable development This type of ash can be used as mortar in various low strength structural components.
2. Objectives
The main objectives of our study: 1. assess the diffuse pollutants in the incinerated medical waste. 2. Examine their possible impact on soil. 3. Investigate the remediation from those metals by solidification. 4. Examine the possibility of utilizing bottom ash as mortar.
3. Scope
The environmental impact of medical waste incinerators has become the subject of public debate. The scenario is more complex for medical waste as these contain not only pathological waste but also hazardous waste which includes radioactive and pharmaceuticals waste. The major byproduct of this incineration process of medical waste is bottom ash which also contains noticeable amount of heavy metals. In Matuail Municipal Waste Landfill Area, located in Damra, Narayanganj, Bangladesh generates bottom ash from medical waste incinerator that was studied. Quantity of heavy metals Zn and Ni as well as nutrient Ca and Mg in the bottom ash was determined. Metal to metal co-relationship was stabilized. Four indexes were used to determine the impact of pollution load. These are1. Pollution load index. 2. Ecological risk index 3. Enrichment Factor. 4. Geo-accumulation Index.Solidification was done to minimize this pollution. Also measured the toxicity of leaching of those metals and compared with standard.
4. Literature Review
Toxic metals such as Zn and Ni are contained in medical waste that cannot be removed by incineration if incinerators are not properly designed and operated. This paper is to summarize the findings from this study regarding the behavior of metals in incineration processes. Highlights of these findings are: (1) Zn is highly toxic heavy metal that is present in medical waste as large amounts of zinc may cause stomach cramps, nausea, and vomiting. It can also cause anemia, pancreas damage, and lower levels of high density lipoprotein cholesterol (beneficial cholesterol). Breathing large amounts of Zn can cause a specific short-term disease called metal fume fever, especially found in bandages or needles. (2) Ni is another toxic heavy metal that has been detected in our experiment. Exposure to Ni metal and soluble compounds (as Ni) should not exceed 0.05 mg/cm3 (8-hour time-weighted average per 40-hour work week). Nickel sulfide fume and dust is recognized as being potentially carcinogenic. (3)Nutrient Ca & Mg are also detected in our experiment. (4) Strength test of mortar block to reduce heavy metal contamination from ashes of medical waste.
4.1. Definition of Medical Waste
Medical waste, also known as clinical waste, normally refers to waste products that cannot be considered general waste, produced from healthcare premises, such as hospitals, clinics, doctor’s offices, labs and other types of health-care facilities. Medical waste defined - as "any solid waste that is generated in the diagnosis, treatment, or immunization of human beings or animals, in research pertaining thereto, or in the production or testing of biologicals." This definition includes, but is not limited to: 1. Blood-soaked bandages 2. Culture dishes and other glassware. 3. Discarded surgical gloves. 4. Discarded surgical instruments. 5. Discarded needles used to give shots or draw blood (e.g., medical sharps). 6. Dultures, stocks, swabs used to inoculate cultures. 7. Removed body organs (e.g., tonsils, appendices, limbs). 8. Discarded lancets.
4.2. Classification of Medical Waste
The following medical waste is commonly regulated by states: (1) Infectious waste: waste from autopsies, animal bodies, and other waste items that have been inoculated, infected, or in contact with such agents is called highly infectious waste. That contains pathogens (bacteria, viruses, parasites, or fungi) in sufficient concentration or quantity to cause diseases in susceptible hosts. (2) Pathological and Anatomical Waste: Tissues, organs, body parts, and body fluids removed during surgery and autopsy.(3) Human Blood and Blood Products: Also known as “liquid medical waste”, this waste stream usually encompasses waste blood, serum, plasma, blood products, and other potentially infectious materials.(4) Cultures and Stocks of Infectious Agents: Also called “microbiological waste”, this waste stream includes specimens from medical and pathology laboratories. Includes culture dishes and devices used to transfer, inoculate, and mix. Also includes discarded live and attenuated vaccines.(5) Sharps: Contaminated hypodermic needles, syringes, scalpel blades, Pasteur pipettes, and broken glass.(6) Isolation waste: Generated by hospitalized patients isolated to protect others from communicable disease. (7) Contaminated Animal Carcasses, Body Parts, and Bedding: Also called “animal waste”, this waste stream is relevant to animals or materials in contact with animals intentionally exposed to pathogens in research, biological production, or in vivo pharmaceuticals testing.(8) Genotoxic waste: Genotoxic waste is highly hazardous and may have mutagenic, teratogenic, or carcinogenic properties. It raises serious safety problems, both inside hospitals and after disposal, and should be given special attention. Genotoxic waste may include certain cytostatic drugs, vomit, urine, or faeces from patients treated with cytostatic drugs, chemicals, and radioactive materials. Cytostatic drugs, the principal substances in the category, have ability to kill or stop the growth of certain living cells and are used in chemotherapy of cancer.
4.3. Waste with High Content of Heavy Metals
Waste with a high heavy-metal content represent a subcategory of hazardous chemical waste, and are usually highly toxic. Mercury waste are typically generated by spillage from broken clinical equipment but their volume is decreasing with the substitution of solid-state electronic sensing instruments (thermometers, blood-pressure gauges, etc.). When- ever possible, spilled drops of mercury should be recovered. Residues from dentistry have high mercury content. Cadmium waste comes mainly from discarded batteries. Certain reinforced wood panels containing lead are still used in radiation proofing of X-ray and diagnostic departments. A number of drugs contain arsenic, but these are treated here as pharmaceutical waste.
4.4. Emission from Incinerator
Most energy-recovery facilities use sophisticated combustion-control systems designed to optimize combustion, minimize ash for disposal, and optimize clean burning by reducing the formation of products of incomplete combustion (PICs). Several are reported as possible human carcinogens or toxins, including Cd, Cr, Ni, Pb, Hg, As, Ba, and Be. Al, Cu, Fe, Pb, Ti and Zn are found largely in slag. More volatile elements such as Cd, Pb, Sb, Se and Sn are vaporized and condense on fine particles, which are either trapped or escape to the atmosphere as suspended particulates. Volatile chlorides of elements including As, Cd, Ni, Pb, Sb, and Zn are also formed, which greatly increase their presence in fly ash and suspended particulates. Over 80% of inputted Hg, largely from Hg batteries, is estimated to be released in gas phase as halides. Some of the waste that goes through the incineration process, however, might exit the system in one of the following forms(1) Combustion gases-can exit through the stack if they are not completely removed by air-pollution- control devices.(2) Particulate emissions-lightweight particles can exit the combustion chamber along with combustion gases, if they are small enough to get past pollution-control devices.(3)Fly ash-toxic particles light enough to be borne upward with combustion gases; a portion of these might not be heavy enough to fall or might not be large enough to be captured by pollution - control devices before exiting the stack. Comprising approximately 25% of all incinerator ash, fly ash often contains high levels of heavy metals, acid gas constituents, and PICs such as dioxin. (4) Bottomash—un-combusted waste such as glass and metal, generally considered nontoxic; approximately 75% of all incinerator ash.
4.4.1. The Bottom Ash from Medical Waste Incinerators
Incinerator ash is divided into two categories: Bottom ash and fly ash. Most of the ash is bottom ash that is the residues inside the burner after incineration. Fly ash settles on post burner equipment such as scrubbers. Bottom ash is the residue resulting from the incineration of waste. Bottom ash is processed to remove ferrous materials and screened into two sizes to remove all unacceptable material. Recent paper reported that metals, such as Ni and Zn in bottom ashes from a medical waste incinerator were with high leach ability. The useful ways to get is to put ashes in construction materials, or in cement, or to solid waste landfills. Bottom ash is reported with less contaminated with heavy metals than fly ash. Previous studies have shown that bottom ash from incineration of municipal solid waste (MSW) might be a valuable resource because it can be used as a secondary aggregate in roads and construction materials. Due to the chemical compositions of medical waste ash and MSW ash are similar; medical waste ash might be reusable in the same way. However, medical waste bottom ash has some special characteristics that must be taken into consideration before it can be reused. medical waste contains large amounts of disposal metallic or plastic materials. Therefore, the bottom ash from medical waste incineration may contain a large proportion of toxic metallic elements or organic compounds that might hinder its reuse. Previous studies have indicated that medical waste bottom ash contains higher amounts of heavy metals such as Ni, and Zn than does MSW bottom ash. In addition, a recent report shows that even though bottom ash is regarded as a non-hazardous material, its TCLP and PBET leachate also showed bio-toxicity. Currently, there are large amounts of low-standard medical waste incinerators are being operated by some rural and urban medical institutions, which are lack of air pollution control devices and without secondary combustion chamber and burning temperature are usually not so high. Thus, the composition and distribution of toxic elements in bottom ash from these incinerators may quite different from that generated from well equipped large incinerator. I observed this by field survey and collected the actual data.Therefore, the properties of medical waste bottom ash must be extensively investigated before this type of special waste can be reused. The objective of the current study was to obtain basic information about medical waste bottom ash by examining heavy metal and ion concentrations, cement solidification and leaching behavior from it. This information should be useful for evaluating utilization possibilities of medical waste bottom ash.
4.5. Impacts of Zn and Ni
The sources of Ni are arsenide and sulphide ores. Ni is used for alloying, as a catalyst in chemical reactors, for battery making and metal plating. It is deposited in medical waste. Its route of exposure to humans, animals, and birds is through drinking contaminated water and dust from the atmosphere. Its health effects include; the disturbance of respiratory system and asthma, birth defects, vomiting and damage to Deoxyribonucleic Acid (DNA) at high concentrations. Lawrence et al (2004) reported negative effect of Ni on abundance of phototropic organisms like Algae and Cyanobacteria.Zn is a metal used in galvanizing and alloying. It is also used in manufacturing electric goods, dying, insecticides and cosmetics. Leach ability of metals (Zn and Ni) assesses risk of landfills to human health and environment. The pollution can be evaluated by leaching effect of the heavy metals. So leaching of heavy metal and stabilization of leaching effect should be considered toxic in medical waste management.
5. Methodology for the Treatment of Sample
5.1. Sample Collection & Preparation
Bottom ash samples were collected from a typical incineration facility of Matuail Municipal Waste Landfill Area, located in Damra, Narayanganj, Bangladesh. This incinerator is a special type of medium-scale one, which combined more than ten small fixed grate furnaces. Part or all of the furnaces are operated according to the amount of medical waste collected. Three mixed samples were collected from the incinerator every 10 days during a month then conducted triplicate analyses. The samples were dried at 105℃ for 24 h and then ground to a particle diameter of <0.25mm in an agate mortar for analysis of heavy metals. The pH was measured in solutions after 24 h of agitation with distilled water at a liquid to solid ratio of 10.
5.2. Determination of Heavy Metals
5.2.1. Extraction of Ni and Zn
In standard method, first, the sample, ashes of medical waste was taken and dried. Then it was weighted. 5gm of it was taken. 50 ml aquaregia were mixed with 5 gm sample. This mixer was kept for 24 hours after mixing properly. Then after 24hours sample was mixed and digested in to the heat Menthol. Then it was filtered. After filtration, sample was ready for detection.
5.2.2. Detection Procedure
Two machines were used for the detection of heavy metals and cations: 1. UV meter was used for the detection of Zn and Ni 2. Atomic Absorption Spectrometer(AAS) was used to detect Ca and Mg.
6. Methods for Estimating Pollution Impact
6.1. Pollution Load Index
Tomlinson’s pollution load index (PLI)(12-14) of the samples was calculated using the heavy metal data and concentration of metal calculation for the world shale average as the background value(8). As a concentration factor (CF) of each metal with regarding to the background value in the sediment the PLI is obtained by applying the following equation:
Where, CF = contamination factor and n = number of metals.CF = Cmetal/Cbackground.By calculating the nth root from the n number of obtained CF for all the metals(15) the ash PLI can be achieved. The standard categorization of PLI is shown in Table 1 where the values vary from 0 to 10.Table 1. Categorization of PLI |
| PLI value | Soil quality | 8-10 | extremely polluted | 6-8 | strongly polluted | 4-6 | significantly polluted | 2-4 | moderately polluted | 0-2 | unpolluted to slightly polluted |
|
|
6.2. Ecological Risk Index
The summation of the change undergoes in metals with respect to background values considering toxicological factor is defined as Ecological risk index (RI)(17). The mathematical relation of RI can be shown as
Where, n is the number of heavy metals, Ti is the toxic-response factor for a given substance (for Hg, Cd, As, Cr and Zn 40, 30, 10, 2 and 1 accordingly; and 5 for Pb, Cu and Ni (10)Ci gives metal content in soil and C0 is the of heavy metals regional background value. Because of the regional background values of measured heavy metals were not available, the metal concentrations for the world shale average were chosen as the background value. Three contamination categories are recognized on the basis of the ecological risks. RI < 300, 300 ≤ RI ≤ 600 and RI > 600 state low to moderate, high (RIh) and extremely high ecological risks (RIe)(10-11) in heavy metals successively.
6.3. Enrichment Factor
Enrichment Factor is defined as the minimum factor by which the weight percent of mineral in an ore-body in this case the samples is greater than the average occurrence of that mineral in the Earth's crust. It can be used to compare the necessary enrichment of different types of minerals for their recovery to be economically viable. Enrichment factor (EF) of an element in the studied ash samples was based on the standardization of a measured element against a reference element. Based on low occurrence variability a reference element is often characterized. The EF is expressed below as
Where the metal to K ratio in the samples of interest is represented by (Cx/K)soil, and (Cx/K)background is the natural background value of the metal to K ratio. In this study, the concentration of K+ is assumed as K. Admittedly, as K and heavy metal background values for our study area are not available, the average continental shale metal values have been taken. There is no accepted pollution ranking system or categorization of degree of pollution on the enrichment ratio and/or factor methodology. There is no accepted pollution ranking system or categorization of degree of pollution. The proposed EF classes along with the sediment quality at various values are shown in Table 2.Table 2. Categorization of EF |
| Level of enrichment | EF value | EF class | extremely high enrichment | > 40 | 6 | very high enrichment | 20-40 | 5 | significant enrichment | 5-20 | 4 | moderate enrichment | 2-5 | 3 | minimal enrichment | 1.5-2 | 2 | enrichment entirely from crustal materials | 0.5-1.5 | 1 | enrichment from point and non-point sources (Ep) | < 0.5 | 0 |
|
|
6.4. Geo-accumulation Index
The Geo-accumulation index (Igeo) was originally defined by Müller (1979) for a quantitative measure of the metal pollution.The Igeo was calculated using Muller18 and Abrahim & Parker3 method as follows: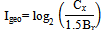
7. Mortar Block
To find out whether the ash can be used with sand as a fine aggregate a certain amount of laboratory experiments have been conducted. The sand and ash was mixed with various ratios and compared with each other. For this purpose 50mm x 50mm mortar block has been tested. The ratios that have been considered to determine the strength of mortar was 1:2.75:4 (cement: sand: water), in which the ratio was further divided according to the various ratios of sand and ash, those were 1:(90%+10%), 1:(80%+20%), 1:(70%+30%) ; cement: (sand%+ash%).The mortar blocks were prepared by following the standard procedure. After preparation, the blocks were stored undisturbed for 24 hours. After 24 hours those specimens were released from the mould and were further cured in water. After specific days the specimens were taken out from the water and dried for half an hour. The specimens were tested to determine the crushing strength by using ASTM compressive testing machine.The peak load and strength was obtained from the machine and the obtained result was analyzed and compared to each other. Depending on the comparison of the results discussion was prepared.
7.1. Leaching from Mortar Block
To detect the leaching from block, three blocks of three different ratios were kept in 2000 ml of 0.1N HCl water. Then 100 ml of that acidic solution was taken to detect heavy metals for leaching effect every three alternate days. Additional 0.1N HCl of same quantity water was also added.Leaching is a very important mechanism and further in-depth experiments are needed regarding leaching mechanism. In my test, total duration of leaching test was 12 days although it is not sufficient. I conducted test to get heavy metal concentrations for leaching in 12 days period.
8. Result & Discussion
8.1. Physical Characteristics of Bottom Ash
Physical tests were performed on individual bottom ash samples from each sampling day to determine the pH value.
8.2. Values of pH
The Ph value detected for each samples from January to June. b is lowest and highest value is 9.64 with a mean value of 9.19. This defines the samples are caustic in nature.The values of pH are shown figure-1.
8.3. Chemical Property
Mg & Ca are the nutrients found in this study. The presence of these cations is beneficial for soil.
8.3.1. Ca
The concentration of Ca has been found within the range of 435 to 754 Mg/kg. The highest value is found in the sample collected at June and the lowest value is found in the sample from March.
8.3.2. Mg
The concentration of Mg has been found within the range of 372 to 1103Mg/kg. The detail values of concentration of heavy metals & nutrient are shown in figure-2.
8.4. Results of Heavy Metals Analysis in Bottom Ash
After pH analysis, the bottom ash samples were investigated for heavy metals’ concentration (zinc, Ni) which is shown in Figure 4. The maximum and minimum values of heavy metal & nutrient are also shown on figure-3.Details of the analysis are given below
8.4.1. Zinc
The concentration of zinc in bottom ash was found to be in the range of 578-1372 mg/kg. From Figure 4, the highest concentration of zinc was found to be in May. The lowest concentration was found to be in march with a mean value of 994 mg/kg .
8.4.2. Nickel
The concentration of silver in bottom ash was found to be in the range of 105- 410 mg/kg which is from sample collected at May and January respectively. The data is shown in table 3. The mean value of nickel is found 237 mg/kg. Concentration of Zn & Ni is shown in the diagram. Here, it has been also shown that all the values of Ni exceed the tolerable level of 40mg/kg and Zn is also higher than the tolerable of 300mg/kg according to the soil standard.Table 3. Heavy metal concentration |
| Sample | Heavy Metal (mg/kg) | | Ni | Zn | January | 240 | 1108 | February | 356 | 967 | March | 410 | 578 | April | 190 | 854 | May | 105 | 1372 | June | 120 | 1087 |
|
|
8.5. Pollution Load Index Analysis
CF values of the mentioned below heavy metals varies between 6.084 to14.442 mg/kg for Zinc and it is 5.645 to 19.140mg/kg for nickel. The mean value is 10.467mg/kg and 12.733mg/kg for Zn and Ni respectively indicating that contamination with Ni in the soil is the highest in comparison to Zn, however the Nickel CF shown a large variance phenomenon in its concentration.
8.5.1. Enrichment Factor Evaluation
Enrichment factors were calculated from the concentrations of the heavy metals in the bottom ash. EF of the heavy metals in ash samples showed that Zn had values varies from 15.91 to 29.99 with a mean 25.67. And for Nickel with an average of 34.63, the EF ranges from 8.94 to 57.63. This is shown in table 4Table 4. EF factor of heavy metal |
| Bottom ash samples | EFZn | EFNi | Class ofEF | EF Contamination level | JANUARY | 23.38 | 25.87 | 5 | Very high enrichment | FEBRUARY | 29.99 | 56.39 | 6 | extremely high enrichment | MARCH | 15.91 | 57.63 | 6 | extremely high enrichment | APRIL | 42.08 | 47.81 | 6 | extremely high enrichment | MAY | 22.86 | 8.94 | 5 | very high enrichment | JUNE | 19.76 | 11.14 | 4 | Significant enrichment |
|
|
8.5.2. Ecological Risk Index
Ecological risk index for Zinc has been found in the range of 6.08 to 11.66 at an average value 10.47 while for Ni the minimum value has been found 28.23 and the maximum value 110.22 at an average value 63.67. The summarized RI index varied at the range of 42.67 to 116.30 and the min value is found 74.13It can be said that in the perspective of RI index, Zn & Ni both are at low to moderate level as all RI values are less than 300 according to contamination category.
8.5.3. Geo-Accumulated Risk Analysis
Table 5. GAI of heavy metal |
| Bottom ash samples | Igeo | Class | Bottom ash Quality | Zn | Ni | JANUARY | 2.96 | 3.10 | 4 | Strongly contaminated | FEBRUARY | 2.76 | 3.67 | 4 | Strongly contaminated | MARCH | 2.02 | 3.88 | 4 | Strongly contaminated | APRIL | 2.58 | 2.77 | 3 | Moderately to strongly contaminated | MAY | 3.27 | 1.91 | 4 | Strongly contaminated | JUNE | 2.93 | 2.10 | 3 | Moderately to strongly contaminated |
|
|
Geo-accumulation Index for Zn shows a variety in their values from 2.02 to 3.27 with an average value 2.74 and 1.91 to 3.88 for Ni with an average value of 2.91. From the evaluation we can define that the Ni contributes more contamination than Zn in perspective of Geo-accumulation Index. It is shown in table 5
8.6. Correlations
Correlation between the heavy metals and nutrients are shown in the table 6. Between Ni & Mg no significant correlation exists. And the correlation is two tailed in characteristics.Table 6. Correlation between heavy metals |
| | Ca | Mg | Zn | Ni | Ca | 1 | | | | Mg | .074 | 1 | | | Zn | .268 | .303 | 1 | | Ni | .151 | -.643 | .775 | 1 |
|
|
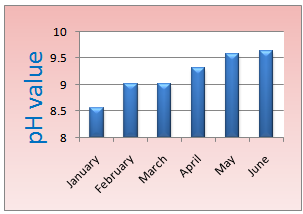 | Figure 1. pH value |
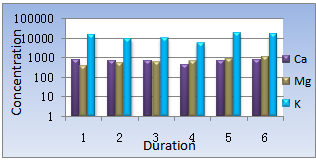 | Figure 2. concentration of heavy metals & nutrient |
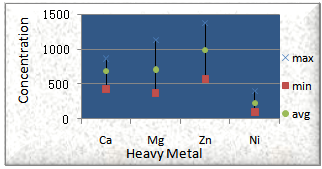 | Figure 3. Maximum & minimum value of heavy metal & nutrient |
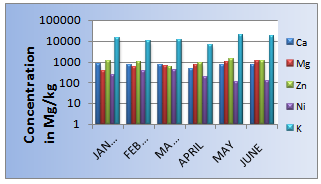 | Figure 4. Concentration of heavy metals |
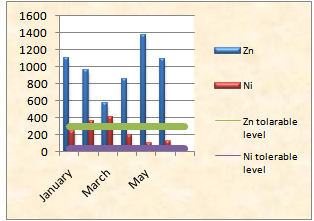 | Figure 5. Concentration of Zn & Ni and tolerable limit of Zn & Ni |
8.7. Compressive Strength of Mortar Block
From the laboratory experiment we found compressive strength of mortar (50 mm x 50mm) for 1:2.75:4 for the various mixing ratio of sand and ash. The obtained results are shown in a tabular from below. Figure 6From the study it is evident that the compressive strength for the cement- sand mortar and for the mixture of sand and ash is quite different.Fig 6 shows the compressive strength of the mortar of different ratio. It can be seen that after 7 days the compressive strength of mortar (50mm x 50mm) for the ratio of 1:2.75:4(cement: sand:water) is 17.4 MPa and that for a ratio of (1:90%+10%) (cement: sand+ash) is 14.54 MPa. Again the strength for the ratio (1:80%+20%) and (1:70%+30%) are 9.46 MPa and 6.36 Mpa respectively. For 14 days the maximum strength is obtained for 1:2.75 (cement: sand) ratio is 17.56 MPa and that for a ratio of 1:90%+10% (cement: sand +ash) is 14.8 MPa. The strength for the ratio (1:80%+20%) and (1:70%+30%) are 10.43 MPa and 7.88 Mpa respectively.
8.8. Leaching
8.8.1. Zinc
The concentration of zinc in the samples for toxicity tests were in the range of 8.19 to 10.51 mg/L, with the mean concentration at 9.34 mg/L. The typical data of zinc concentrations in simulated leachate are shown in Figure 7. Heavy metals concentrations in simulated leachate were very low when compared to heavy metals in bottom ash. Because the leachability tests were undertaken under laboratory conditions the bottom ash in block as a proxy of fine aggregate and also in a low percentage .Water in contact with bottom ash produced alkaline solutions rather than acidic ones. This made the heavy metals concentrations in bottom ash leachate much less than in bottom ash.[7] The leachability of metals adsorbed on bottom ash particles is enhanced, since the heavy metals largely occur in the smallest size fraction and concentrated at or near the surface of the particle. The small particle size increases available surface area exposed to leaching.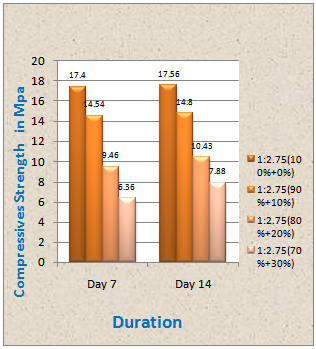 | Figure 6. compressive strength vs. duration graph |
8.8.2. Nickel
The concentration of Nickel in the solutions was in range of 1.2-2.03 mg/L, with the mean at 1.72 mg/L. The typical data of Nickel concentrations in simulated leachate are shown in Figure 7. From figure 7 the leaching phenomenon can be described as, with the time increases the contamination of the Zn and Ni and concentration of other metals increases. But it should be also mentioned that the contamination rate decreases as more time passes by. Leaching of heavy metals is very less considering Indian compost quality standards 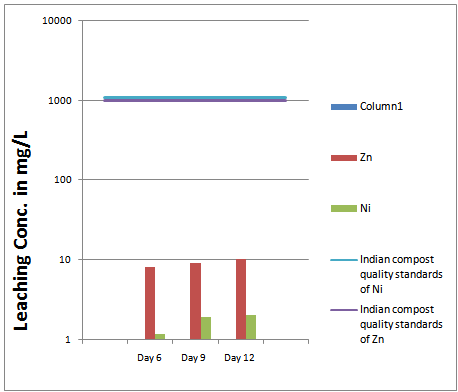 | Figure 7. leaching concentration for heavy metal |
9. Conclusions& Recommendation
9.1. Conclusions
The hazardous nature of bottom ash generated by incineration of medical waste due to the presence of heavy metals is demonstrated by the results of this study. These metals are not destroyed by Incineration but simply disperse them via the incinerator stack and, as demonstrated in the current study, concentrate them into bottom ash. Far from solving a waste problem, a new and more toxic one is simply created by incineration. Based on the results these studies, following conclusions are being made: A high level of toxic heavy metals is being contained with medical waste bottom ash, which is indicated by our results: (1) Serious environmental problems can be caused by this type of waste ash if not properly managed.(2) High concentrations of metallic elements, such as Zn and Ni were determined, and most of these metals were associated with the stable residual fraction. (3) Nutrients Ca and Mg were also assessed. (4) A solution for safe management of hazardous MW bottom ash is being tried to find out, and the compressive strength of mortar blocks were satisfactory. (5) Leaching of heavy metals were found lower as per Indian compost quality standards. Therefore, it can be concluded that ash can be recycled as construction material (fine aggregate), and can be used in preparing mortar for less strength required purposes, such as rings of pit latrines, pavement tiles for footpaths.
9.2. Recommendations
Following recommendations are made for the future research on heavy metals in medical waste incinerators bottom ash: (1) A detailed chemical waste characterization must be undertaken to determine whether a medical waste incinerator bottom ash should be classified as hazardous and what leachable constituents are present. (2) In order to protect ground water, medical waste incinerator bottom ash should be disposed by properly designed-engineered treatment methods. (3) More investigation should be carried out on the bottom ashes produced from waste incinerators in various hospitals with regards to their hazardous nature due to the presence of heavy metals. (4)Potential reuse of incinerator ashes in civil engineering applications should be evaluated. However, it is necessary to address the engineering, environmental and economic concerns before large scale use of the ash considered.
APPENDIX
Index CalculationPollution load index: For June Zn and Ni conc. were 1087 mg/Kg and 120 mg/Kg. We know,Concentration factor,
Now, CNzn= (1087/95) =11.442& And CNNi = (120/18.6) =6.452
So the PLI value for June is found 8.59Enrichment factor: The concentration of Zn and Ni were 1372 mg/Kg and 105mg/kg in May and K was 18097 mg/kg.EFZn = (1372/18097) ÷ (95/28650) = 22.86EFNi = (105/18097) ÷ (18.6/28650) = 8.94The highest value is taken for metal enrichment of ash sample. For May enrichment factor is 22.86Ecological Risk Index:For March Zn and Ni conc. were found 578 mg/kg and 410mg/kg.We know, Ecological Risk Index,
Now, RI Zn =1*(587/95) =6.08and RINi =5*(410/18.6) =110.22Now, ∑RI =RIZn + RINi = (6.08+110.22)=116.30So for March the Ecological Risk Index is 116.30Geo-accumulation Index:For January Zn and Ni concentration were found 1108 mg/kg and 240 mg/kg
Now, IgeoZn= log2(1108/1.5*95)=2.96IgeoNi = log2(240/1.5*18.6)= 3.10The highest value, 3.10 for January, among this two is taken for Igeo Index evaluation.
References
[1] | Aren, Mandal; and Sengupta, D. (2003). Radioelemental study of Kolaghat, thermal power plant, West Bengal, India: possible environmental hazards. Environ. Geol., 44, pp. 180–186. |
[2] | Fengyuan, Piao; Fanyin, Cheng; Haibo, Chen; Gang, Li4; Xiance, Sun;,Shuan, Liu; Received January 12, 2007 and accepted May 22, 2007 Effects of Zinc Coadministration on Lead Toxicities in Rats5 |
[3] | Hang, Yongming; D. Peixuan, C. Junji and E.S. Posmentier (2006). Multivariate analysis of heavy metal contamination in urban dusts of Xi’an, Central China, Sci. Total Environ., 355, pp. 176–186. |
[4] | Kurtus,Ron. Explanation of the pH scale for measuring acids and bases. |
[5] | Kabir, Imran, H. Lee, G. Kim and T. Jun, Q IWA Publishing 2010, Water Science & Technology—WST |
[6] | L. Hakanson (1980). An ecological risk index for aquatic pollution control: a sedimentological Approach. Water Research, 14, pp. 975–1001. |
[7] | Lijuan, Zhao; Fu-Shen, Zhang; Mengjun, Chen; Zhengang, Liu; Da Bo JianzhiWu Typical pollutants in bottom ashes from a typical medical waste incinerator. |
[8] | Min-yo, Lee; Sang-il, Choe;Jae-yong, Lee; Kang-kun, Lee and Jae-wo, Park. Soil and Groundwater Environment (Tho-yang Jihasu Hwan-gyeong), Donghwa Publication Co. Ltd., Seoul, Korea 2006, p. 224. |
[9] | Müller, G. (1969). Index of geoaccumulation in the sediments of the Rhine River. Geojournal, 2, pp. 108–118. |
[10] | MOEF (2000). “Municipal Solid Waste Management and handling rules.” Ministry of Environment and Forests, Govt. of India. |
[11] | Narayana, A. C; Priju, C. P. (2007). Heavy and trace metals in Vembanad lake sediments. Int. J. Environ. Res., 1(4), pp. 280–289. |
[12] | Priju, C. P. and Narayana, A. C. (2007). Heavy and trace metals in Vembanad lake sediments. Int. J. Environ. Res., 1(4), pp. 280–289. |
[13] | Study of the typical solid waste composition in Phuket Province of Thailand, (Ministry of public health, 1997). |
[14] | Tomlinson, D. L.; Wilson, J. G.; Harris,C. R. and Jeffney, D. W. (1980). Problems in the assessment of heavy metals levels in estuaries and the formation of pollution index. Helgol. Wiss. Meeresunters, 33, p. 566. |
[15] | Xinwei, Lu; Lijun, Wang; Kai, Lei; Jing, Huang and Yuxiang Zhai (2009). Contamination assessment of copper, lead, zinc, manganese and nickel in street dust of Baoji, NW China. Journal of Hazardous Materials, 161, pp. 1058–1062. |
[16] | Xinwei, Lu; Lijun, Wang; Kai, Lei; Jing, Huang and Yuxiang Zhai (2009). Contamination assessment of copper, lead, zinc, manganese and nickel in street dust of Baoji, NW China. Journal of Hazardous Materials, 161, pp. 1058–1062. |
[17] | Zhifeng, Yang; Ying,Wang; Zhenyao, Shen; Junfeng, Niu and Zhenwu, Tang (2009). Distribution and speciation of heavy metals in sediments from the mainstream, tributaries, and lakes of the Yangtze River catchment of Wuhan, China. Journal of Hazardous Materials, In Press. |
[18] | Zhifeng, Yang; Ying,Wang; Zhenyao, Shen; Junfeng, Niu and Zhenwu, Tang (2009). Distribution and speciation of heavy metals in sediments from the mainstream, tributaries, and lakes of the Yangtze River catchment of Wuhan, China. Journal of Hazardous Materials, In Press. |