Jin-Won Nam 1, Alex Hak-Chul Shin 2
1Department of Civil and Environmental Engineering, Southern University and A&M College
2Department of Civil and Environmental Engineering,Louisiana State University and Southern University
Correspondence to: Alex Hak-Chul Shin , Department of Civil and Environmental Engineering,Louisiana State University and Southern University.
Email: |  |
Copyright © 2012 Scientific & Academic Publishing. All Rights Reserved.
Abstract
Thisresearch aims tothe development of combined damage model of concrete material under impact and thermal loading conditions. Down-scaled experimental works were carried out for the quantitative evaluation of combined damage from impact and thermal loading. The experimental results of this research include measurement of weight loss of concrete, chemical composition analysis, and strength degradation after impact and fire exposure. The damage of concrete under high temperature and impact loading conditions was studied with experimental measurements. Weight loss of concrete specimen shows the range of 1 % to 8 % with three different levels of temperatures: 200℃, 500℃, and 800℃. At low and intermediate temperature levels, damage from impact loading was recovered and strength of concrete increased slightly after thermal loading. Rehydration might occur during the thermal loading. At high temperature level, damage of impact was combined with damage of thermal loading and strength of concrete decreased by 80 %.
Keywords:
Concrete Damage, Thermal Loading, Impact Loading
Cite this paper:
Jin-Won Nam , Alex Hak-Chul Shin , "Damage Assessment of Concrete in Thermal and Dynamic Loadings", Journal of Civil Engineering Research, Vol. 2 No. 6, 2012, pp. 49-56. doi: 10.5923/j.jce.20120206.01.
1. Introduction
Accidental events such as traffic accidents, fires, explosions, and natural disasters can happen at any time.Among those accidental events, it is acknowledged that the most frequent are fire and traffic accidents. Even though the event of an explosion is rare, the most catastrophic lasting eventsare of the World Trade Center, and Alfred P. Murrah Federal Building in Oklahoma City. In the perspective of structural engineering, the loads of abnormal event should be considered during the design process within the data of the relationship between abnormal loading events and structural damage. However, there has not been systematic information of the structural damage from those abnormal loads. There have only been studies for the damage from separated abnormal loading conditions, not from the combined conditions[1 to 4]. In real life instances, it is possible to have combined conditions such as impacts with fire, earthquakes with fire, explosive blast pressure waves of high temperature, etc. The impulsive and thermal loadings are considered as the representative abnormal loads.The impulsive loadings involve not only impact loadings but also abnormal high temperatures in the hemisphere of the detonation. The combined load of impulsive and thermal conditions can damage the infrastructures severely in material properties and structural safety. Once the damage occurs, the repair or reconstruction of the structure is needed. There are always consequential sacrifices in money, time, and inconvenience. To minimize consequential effects, it is desirable to systemize the damage evaluation and repair method, which enable the rehabilitation of the structure in a short time. The systematic damage evaluation for the abnormal loading conditions can be developed based on numerous experimental data and reasonable numerical models for the combined impulsive and thermal loadings [5-8]. For the systematic experimental data, it is needed to categorize the damage level of the structure and assess the damage of the structural members according to loading levels. Regardingthe construction materials of structures, steel has a much more distinct behavior under impulsive and thermal loadings compared to concrete. Since there is no specific melting point and yielding behavior for concrete, the prediction and modeling of concrete behavior under impulsive and thermal loading are considered a challenge[9].A research planhas been developed to evaluate the damage of concretestructural members subjected to impact and thermal loads. Figure 2 shows the five steps of the research plan to assess the impulsive and thermal damages of concrete members in qualitative and quantitative data and to develop a damage model for concrete subjected to impact and fire loads. With the experimental data and developed damage model, an effective and reasonable analysis of concrete structures under impact and fire loadings can be developed. This research is the first step of the research plan to assess material damage.The down- scaled experimental works werecarried out for the evaluation of damaged concrete members. The experimental procedure is detailed in Figure 3. The experimental results of research include measurement of weight loss of concrete and strength degradation after impact and fire exposure.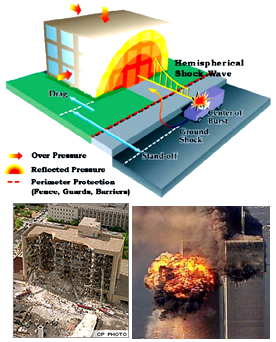 | Figure 1. Catastrophic Failures under Impulsive and Fire Loadings |
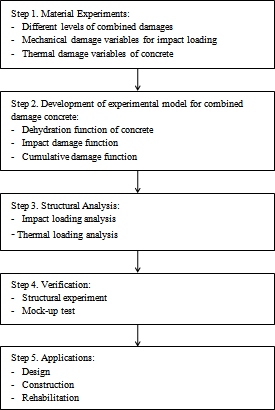 | Figure 2. Research Plan |
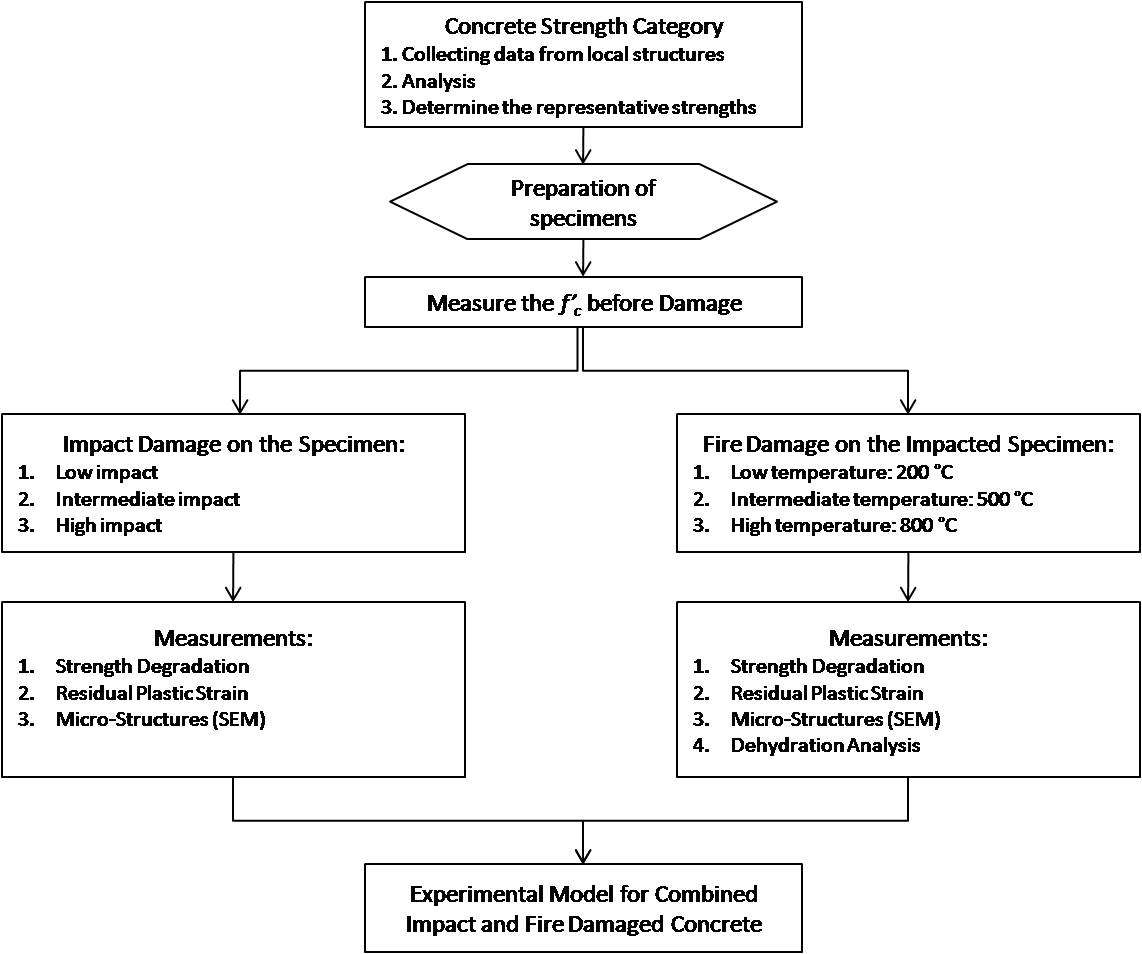 | Figure 3. Experimental Frame |
2.Materials and Specimen Fabrication
Normal Portland cement and river gravel were used for concrete specimens. A water cement ratio of 0.50 was used and the design compressive strength of34.5 MPa was used for the concrete. Cylindrical specimens (100 mm x 200 mm)were fabricated at the beginning of the project. After 28 days of the curing, the specimens were cut into three pieces with identical height because of a space limitation of furnace as shown in Figure 4. The specimens were damaged under impact loading of 4 m/s and thermal loading up to 800℃. Three different levels of combined loadings with impact and fire damage were considered. The results of cylindrical specimens are only presented in this paper.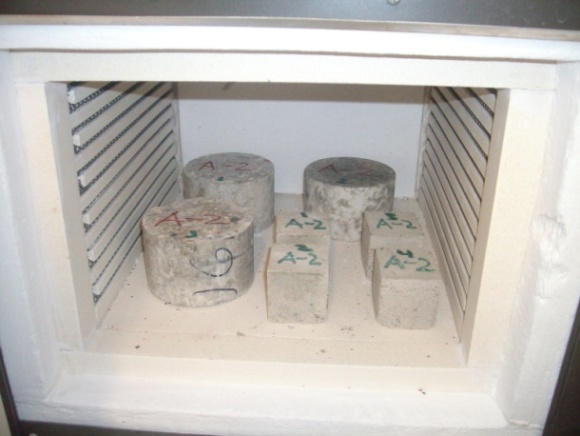 | Figure 4. Cut specimens and furnace |
Thermal LoadingThermal loading with various temperatures affects on the mechanical properties of concrete. In lower level of temperature, thermal energy can increase concrete strength. However, at the higher temperature, concrete can be damaged due to its chemical bond failure. From the previous researches, the critical temperature affecting the properties of concrete can be considered in three levels[2, 7, and 10]. In this study, concrete specimens were thermally loaded in three different temperatures respectively: 200℃, 500℃, and 800℃. The furnace (NEYTECH 185P) used in the test is shown in Figure 5. After the temperature inside of the furnace reached in the designated temperature, the specimens were placed in the furnace for one hour. The specimens were removed from the furnace and cooled down in ambient temperature before compression test. The weight and compressive strength of all specimens were measured before and after heating up as shown in Figure 6. 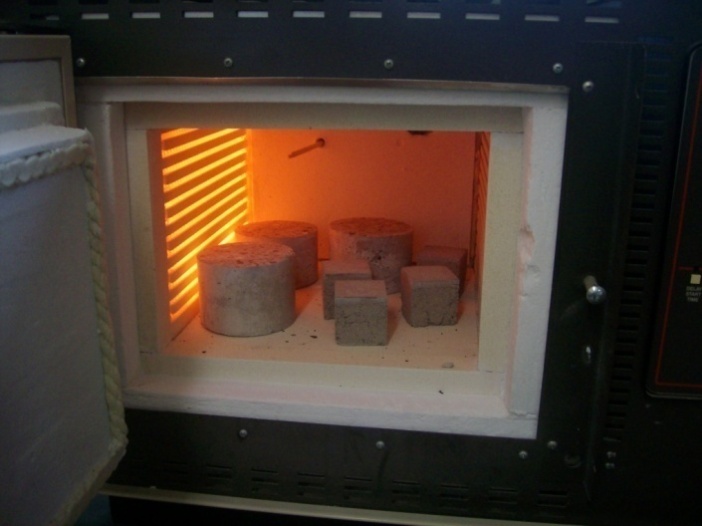 | Figure 5. Specimens under thermal loading in furnace |
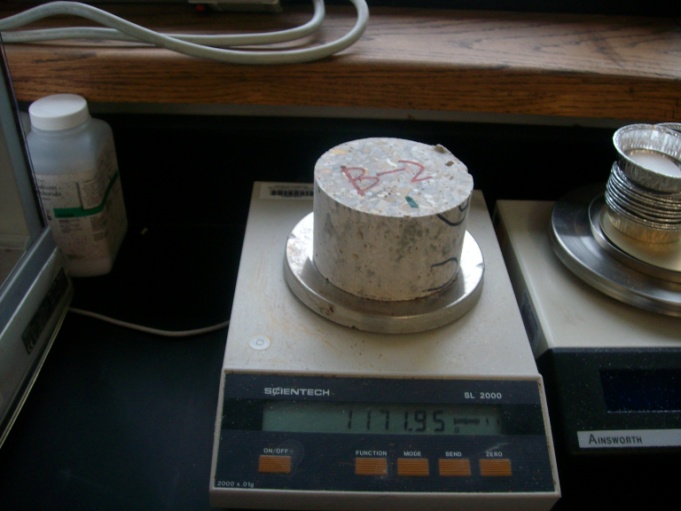 | Figure 6. Measurement of specimen weight |
Impact LoadingInstronDynatup loading frame was used to apply impact loading on the conditioned specimens. (Figure 7) Impact loading tests were performed by placing a specimen in the loading frame and applied impact load using impact hammer. Because the impact hammer is based on free drop from the maximum height of 1 m, the maximum velocity is not more than 4.43 m/s. The range of impact loading rate was 4.0 ± 0.1 m/s and total dynamic energy was 53 ± 0.5 J. To find the relationship between accumulated damage and energy dissipation, total deflection and the deflection at maximum load were measured. By comparing the compressive strength of specimens before and after combined loadings, the effect of impact energy on the thermal damage of concrete can be deduced. 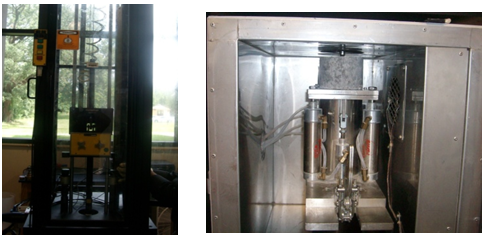 | Figure 7. InstronDynatup and specimen on the measuring equipment |
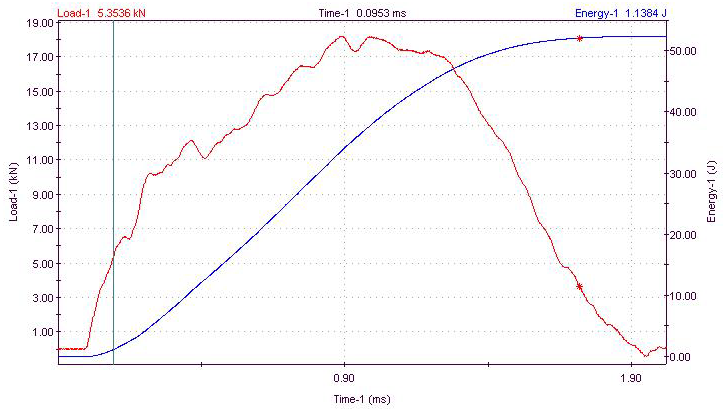 | Figure 8. Measurement of impact energy |
Table 1. The results of impact test |
| Total energy | Total time | Maximum load | Time to max load | Energy to max load | Deflection at max load | Total deflection | Impact velocity | 53.10 J | 2.23 ms | 16.83 kN | 1.17 ms | 37.10 J | 3.80 mm | 5.26 mm | 4.02 m/s |
|
|
Impact Energy and DeflectionThe impact energy and deflection of specimen were measured as shown in Figure 8 and the results are presented in Table 1. Each term has average value and the sample size is relatively small based on the central limit theorem. The standard deviations of total energy, energy to maximum load, total deflection, and deflection at maximum load are 0.46 J, 7.24 J, 0.86 mm, and 1.07 mm. The confidence level of the average total energy and impact velocity are 48.4 % and 99.8 %, respectively. The difference between total energy and energy at maximum load is due to the difference between total deflection and deflection at maximum load. The energy difference can be considered as internal energy accumulated in the specimen. The internal energy might be converted to overall damage of specimen and should have effect on the material damage process under the following thermal loading stage.Weight LossThe weight loss of concrete specimen due to thermal loading was measured. The weight loss of concrete in low and intermediate temperature is mainly from the loss of free water inside concrete, while the weight loss of concrete in high temperatures over 800℃ is due to the combined loss of free water and fixed water. The loss of fixed water is related to chemical reaction and decomposition of hydrates. In most cases, the weight ratio of free water to concrete is in the range of 2 – 5 %. The weight losses of specimens at three different temperature levelsare shown in Figure 9. The weight losses before and after the thermal loading are compared in the figures. At low and intermediate temperatures of 200℃(level A) and 500℃ (level B), 1 % and 6 % of weight loss occurred after thermal loading as shown in Figure 10. At a high temperature of 800℃ (level C), 8 % of weight loss was observed.Due to the small size (100 mm x 66.7 mm) of the specimen after cutting, the heating anddehydration process was faster than typical concrete structures, and the different size effect should be further studied.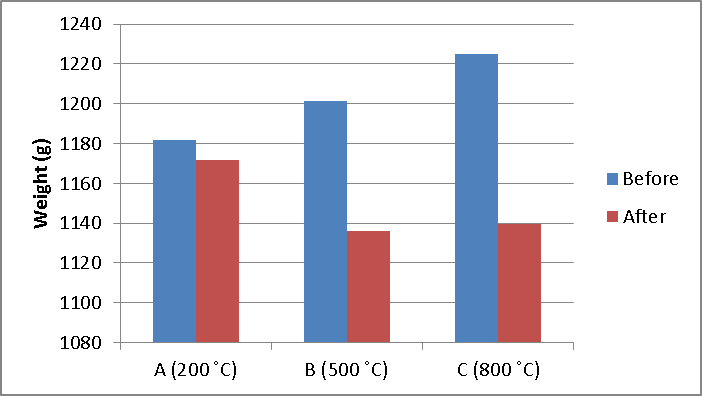 | Figure 9. Weight of specimen before and after thermal loading |
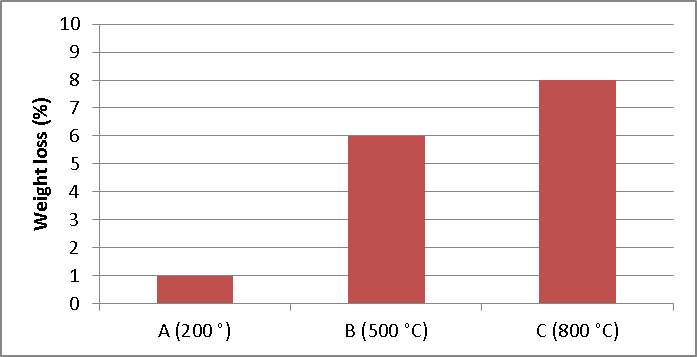 | Figure 10. Weight loss (%) after thermal loading |
Strength DegradationThe strength degradation of concrete specimens was measured after impact loading and consecutive applied thermal loading, respectively. The results are shown in Figure 11. Strength loss after impact loading was in the range of 4-20 % as shown in Figure 12. Severe strength loss occurred after high temperature exposure, while no distinct strength loss occurred after low and medium temperature exposure. Some increase in strength was observed after medium temperature exposure. The reason for strength loss of concrete at high temperature is dehydration of calcium-silicate-hydrate (C-S-H) gels in hardened cement paste. It is known that cement paste starts to dehydrate at about 500℃. But complete decomposition of the C-S-H gel occurs at about 900℃. Since the peak temperature in the furnace was 800℃, decomposition of C-S-H might not be fully completed. In other aspects, it needs additional time to complete the decomposition process. The heating up process can be considered to be as an energy providing process. If the concrete specimen is not over-heated, thermal energy can function as healing solution for the re-hydration and strength up. On the other hand, if the concrete specimen is over-heated at a high temperature, thermal energy plays a role in decomposition of the hydrates. In the tests, the damage from impact might be healed or cured through the relatively low and intermediate temperature exposure, but be affiliated with additional severe damage at the relatively high temperature. As shown in Figure 13, crazing and cracking are observed on the surface of specimen after impact and followed by thermal loading of 800℃.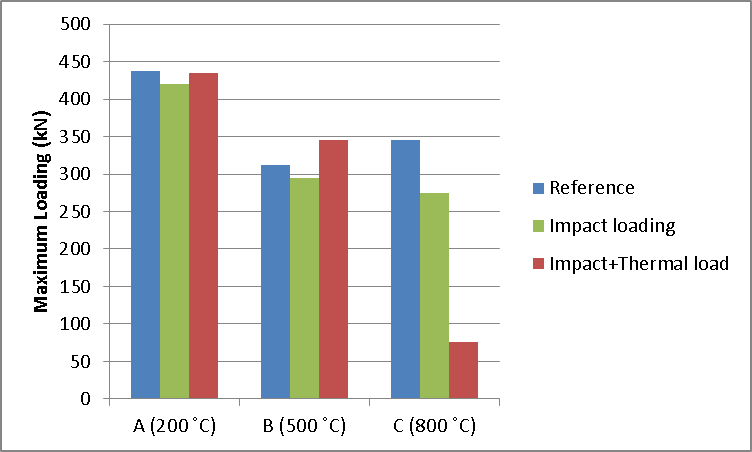 | Figure 11. Strength degradation of specimens after impact and thermal loading |
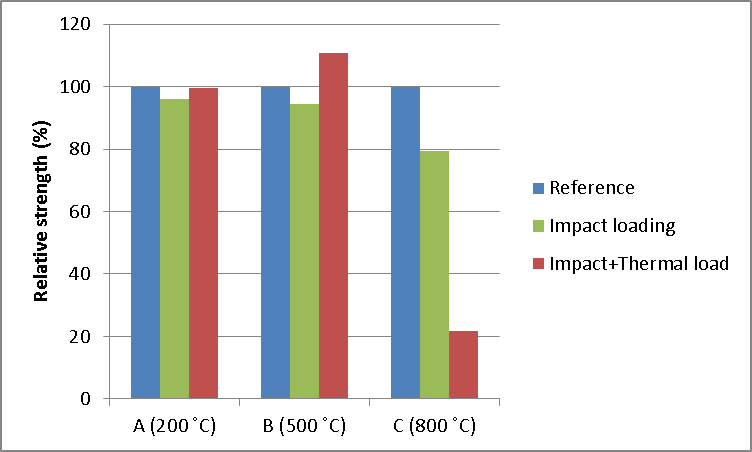 | Figure 12. Relative strength after impact and thermal loading |
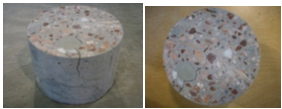 | Figure 13. Cracking after combined loading of impact and 800℃ heating |
SEM (Scanning Electronic Microscopy) AnalysisChemical components of sound concrete and damaged concrete specimens were inspected by Energy-dispersive X-ray spectroscopy (EDAX) analysis combined with scanning electronic microscopy (SEM) as shown in Figure 14. Major components of both specimens such as Si, O, and Ca have similar peak values, while minor components such as Al and Mg are not observed in the thermally damaged specimen. In the aspect of amount, Al and Mg are considered as minor components. However, hydrates from those components can be major components in the aspect of strength. The chemical components analysis is shown in Figure 15. 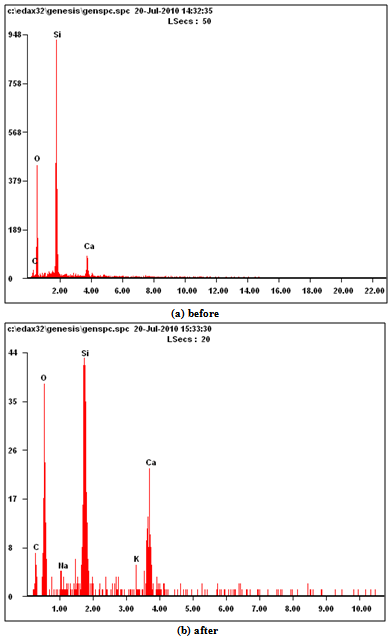 | Figure 14. SEM-EDAX analyses |
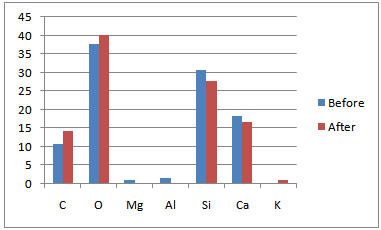 | Figure 15. Chemical components of concrete specimens |
3. Discussions
The results of experimental tests are well matched with the expected trend. However, some data is not in the typical value range. The result of weight loss test shows typical trend and reasonable data, while the result of strength degradation test does not show reasonable data. The errors in strength degradation test are due to several weak points of the test procedure such as the size of specimens, fabrication of specimen, and limitation of equipment. Additional tests should be performed with recalibrated and adjusted methods in further researches. 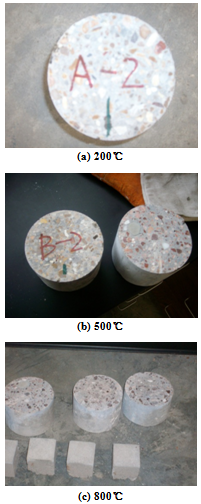 | Figure 16. Color comparison of sections of concrete specimen under different thermal loadings |
Regarding the size of specimens, the specimen height was reduced from 8 inches to 2.5 inches. In the beginning, the size of specimen (100mm x 150 mm) was selected for down scaled test and was supposed to be increased to structural elements such as beams and columns for the test of localized damage on structures in the next research step. However, down scaled specimens were further down scaled due to the space limit of furnace. Impact and thermal energy capacity of specimen is too small to have cumulative material damage inside the specimen and show distinct trend in data. Even more the impact velocity could not be raised up to the supposed range of 5 to 20 m/s due to the limitation of impact equipment. Reduced height of specimen also has size effect which causes inaccurate data during strength measurement. In both ends zone of specimen, there is confinement effect which results in increment of strength of damaged specimen. The data of strength degradation in this research can be overestimated and the absolute value is not highly reliable. Instead, the relative damage index in Figure 12 can be used for combined damage model. Color image analysis can be adopted in the next step of research. Section color of each specimen is shown in Figure 16. Thermal energy can cause chemical reaction inside concrete and results in color change of concrete. Pink was observed in the section of specimen at 500℃ and white-grey was observed in the section of specimen at 800℃. With high quality images, the relationship between damage and sectional color can be obtained.
4. Conclusions and Further Studies
The damage of concrete under high temperature and impact loading conditions was studied with experimental measurements. Weight loss of concrete specimen shows the range of 1 % to 8 % with three different levels of temperatures: 200℃, 500℃, and 800℃. At low and intermediate temperature levels, damage from impact loading was recovered and strength of concrete increased slightly after thermal loading. Rehydration might occur during the thermal loading. At high temperature level, damage of impact was combined with damage of thermal loading and strength of concrete decreased by 80 %. The strength degradation is due to chemical decomposition in concrete and it was verified with SEM-EDAX analysis result. Additional analysis methods such as color image analysis, petrographic analysis, ultrasonic analysis, and CT analysis are considered for further researches to develop the combined damage model.
ACKNOWLEDGEMENTS
The authors would like to acknowledge support from the Louisiana Board of Regents with research grant (NSF(2010)-PFUND-209). The authors would also like to thank Mr. Justin Foster for his assistance in producing the PCC mixtures and completing the hardened concrete testing.
References
[1] | Smith PD, Mays GC. Blast effects on buildings: Design of buildings to optimize resistance to blast loading, 1995, Thomas Telford. |
[2] | Georgali B, Tsakiridis PE. Microstructure of fire-damaged concrete, Cement & Concrete Composites 27 (2005) 255–259. |
[3] | Hertz KD, Sørensen LS. Test method for spalling of fire exposed concrete, Fire Safety Journal 40 (2005) 466–476. |
[4] | Van der Heijden GHA, van Bijnen LP, Huinink HP. Moisture transport in heated concrete, as studied by NMR, and its consequences for fire spalling, Cement and Concrete Research 37 (2007) 894–901. |
[5] | Ingham JP. Application of petrographic examination techniques to the assessment of fire-damaged concrete and masonry structures, Materials Characterization 60 (2009) 700-709. |
[6] | Kodur V, Dwaikat M, Raut N. Macroscopic FE model for tracing the fire response of reinforced concrete structures, Engineering Structures 31 (2009) 2368-2379. |
[7] | Short NR, Purkiss JA, Guise SE. Assessment of fire damaged concrete using colour image analysis, Construction and Building Materials 15 (2001) 9-15. |
[8] | Cioni P, Croce P, Salvatore W. Assessing fire damage to r.c. elements, Fire Safety Journal 36 (2001) 181-199. |
[9] | Zeiml M, Lackner R, Leithner D, Eberhardsteiner J. Identification of residual gas-transport properties of concrete subjected to high temperatures, Cement and Concrete Research 38 (2008) 699–716. |
[10] | Li M, Qian CX, Sun W. Mechanical properties of high-strength concrete after fire, Cement and Concrete Research 34 (2004) 1001-1005. |