C. Celiktas1, M. Bayburt2, S. Bayburt3
1Ege University, Faculty of Science, Physics Department, 35100, Bornova, Izmir, Turkey
2Ege University, Institute of Nuclear Sciences, 35100, Bornova, Izmir, Turkey
3Dokuz Eylul University, Faculty of Engineering, Mechanical Engineering Department, 35100, Bornova, Izmir, Turkey
Correspondence to: C. Celiktas, Ege University, Faculty of Science, Physics Department, 35100, Bornova, Izmir, Turkey.
Email: |  |
Copyright © 2012 Scientific & Academic Publishing. All Rights Reserved.
Abstract
By means of a timing technique, in this work, the energy resolution of a CdTe detector was improved by using the 59.5 keV-energy gamma peak of an 241Am radioisotope. First, this gamma peak was recorded in a multichannel analyzer without timing process in the experiment. Then, the timing discrimination method was applied on this peak to eliminate the non-peak radiations such as background, scattered and backscattered etc. together with the electronic noise component. Energy resolution of the detector was calculated before and after the timing method. Finally, obtained results were compared. It was concluded from the obtained results thatbetter signal-to-noise ratio (SNR), which is an important factor for medical imaging, would be able to have through the timing technique without cooling the detector. This method was successful on the improvement of the energy resolution of the detector.
Keywords:
241Am, Timing Method, Energy Resolution, CdTe Semiconductor Detector
Cite this paper: C. Celiktas, M. Bayburt, S. Bayburt, Energy Resolution Improvement of a CdTe Detector By Means of a Timing Technique, International Journal of Instrumentation Science, Vol. 2 No. 1, 2013, pp. 6-12. doi: 10.5923/j.instrument.20130201.02.
1. Introduction
CdTe is the commonly used semiconductor material for the detection of X-rays and low-energy gamma rays. The basic operating principle of the detectors composed of semiconductors is analogous to gas ionization devices. The passage of ionizing radiation creates electron-hole pairs which are then collected by an electric field. The advantage of the semiconductor is that the average energy required to create an electron-hole pair is some ten times smaller than that required for gas ionization. Thus, the amount of ionization produced for a given energy is an order of magnitude greater resulting in increased energy resolution. Moreover, because of their greater density, they have a greater stopping power than gas detectors. They are compact in size and can have very fast response times[1]. An energy resolution of 1 keV for 6 keV X-rays (Fe kα) and better than 3 keV for 122 keVγ-rays (57Co)is recorded for detectors with a sensitive volume of one cubic centimetre. High counting rates (105/s) can be accommodated by CdTe detectors. Even higher rates may be investigated by using a current working mode instead of a pulse working mode. Depending on the growth method and compensation use, CdTe can be prepared with resistivity up to about109 Ω.cm. Theroomtemperature performance of detectors built onsuch material can become comparable to results obtained with Ge at 77 K for gamma spectrometry [2].Different from the CdTe, today, various type of semiconductor detectors such as HgI2, CdZnTe, Si(Li) and Ge(Li) are widely used in medicine,security, industrial processes, monitoring, astronomy, high energy physics, radioactive waste management, environmental remediation and elementalanalysis of materials.CdTe is a semiconductor which has very advantageous properties as compared to the other semiconductor chalcogenides of Cd, such as comparatively great mobility,a simple controllability of the conductivity from (n)to (p) type and conversely, so that CdTe may be used in semiconductor devices such as crystal diodes or transistors. It is also known that CdTe is photosensitive to many kind of radiation, for example, to infrared and visible radiation and X-radiation, so that it may be used in photosensitive devices such, forexample, as photodiodes or as photoconductive bodies or infrared telescopes, image intensifiers, camera tubes and photoelectric cells, X-ray dosimeters and the like[3].The medical field represents certainly the best opportunity for CdTe detectors thanks to their high absorption cross section, ability to work at rather high temperatures >70℃, low applied bias voltage, and small size (<1mm possible), allowing precise localization. Since the earlier development by Meyer et al.[4] of CdTe probes for 75Se gamma rays in rat’s eyes for diagnosis, a great many studies, instruments, and production of CdTe based probes have appeared on the market and in the literature for various medical applications[3]. Eisen et al.[5] inspected the CdTe detectors for medical and industrial imaging systems. The CdTe detectors in nuclear medicine were handled by Scheiber[6]. Scheiber and Giakos[7] reviewed the medical applications of CdTe semiconductors between 1992 and 1996 years. Ogawa[8] studied the feasibility on an ultra-highresolution SPECT with CdTe detectors in his paper. A comparison between scintillation and semiconductor detectors such as CdTe and CdZnTe with respect to their timing properties for triple coincidence measurements and imaging was carried out by Abuelhia et al.[9]. Energy resolution improvement of CdTe detectors was performed by Jones and Woollam[10] and Richter and Siffert[11] as well. In addition, various works have been published in relation with the CdTe detector performance[12-15].The energy resolution of a detector is given through the full width at half maximum (FWHM) of an energy peak.The FWHM is defines as the width of the distribution at a level that is just half the maximum ordinate of the peak[16].Timing measurement focuses on the determination of the time when a particular event has happened (i.e., a charge particle has crossed the detector), with a certain accuracy. The information on the energy associated with the event has a lesser priority or may even be disregarded. This fact fundamentally changes the way timing radiation systems are designed and optimized.In principle, a system designed for timing measurements transforms the detector signal that corresponds to the desired event into an unambiguous digital signal, separating it from other types of events and from the background noise[17].This work was presented to improve the energy resolution of a spectrometer composed of a CdTe semiconductor detector by eliminating the unwanted signals through a timing detection technique.
2. Material and Methods
In the experimental set-up, pulse shape discrimination (PSD) or rise time discrimination technique was used. This technique can be expressed briefly as follows.When the different types of particles with different energies interact with the detector material, they produce the signals with different amplitudes and rise times. Rise time corresponds to the time between 10-90% duration in the leading edge of a signal. This method is based on the principle that distinguishes the particles according to their rise times. The following setup was constituted for this method.The experimental setup was composed of an Ortec 401A BIN, a CdTe semiconductor detector with its integrated preamplifier, an Ortec 472 spectroscopy amplifier (AMP.), an Ortec 552 pulse shape analyzer/timing single channel analyzer (PSA), an Ortec 437A time to amplitude converter (TPHC), an Ortec 425A delay (D), an Ortec 427A delay amplifier (DA) and an Ortec Trump 8K multichannel analyzer (MCA) card mounted in a computer. The schematic view of the set-up is illustrated in Fig. 1.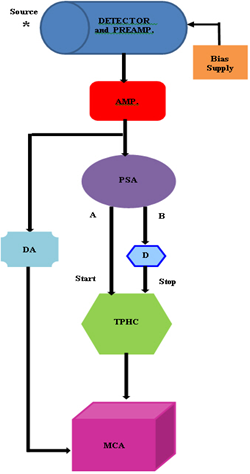 | Figure 1. Schematic view of the used setup |
The radioactive source (10 µCi) used in the experiment was an241Am point source embedded in a mylar sheet with a thickness of 0.5 mm. It was placed in front of the detector window.The detector crystal from Ritec Ltd.[18] had the volume of 3x3x2 mm3, the density of 5.85 g/cm3, the energy band gap of 1.52 eV, the resistivity of 109 Ω.cm, the diameter of 13 mm, the length of 23 mm and the energy resolution of about 1% FWHM at 662 keV. Also, bias voltage of 100 V was applied to the detector.The gamma rays were detected by the detector and the signals from the detector were amplified by the preamplifier and further amplified by the main amplifier. The output of the main amplifier was split into two branches. The first branch was directly connected to the MCA through DA. The other branch was sent to the PSA to have an analog logic timing signal. The PSA produces two timing signals according to the PSD technique. In the PSA, the first signal (A) is generated when the input signal rises 10% of its maximum, and this signal forms the ‘start’ input of the TPHC. The second signal (B) is produced when the input signal rises 90% of its maximum, and this signal is the ‘stop’ input of this device. The time between start and stop input signals is measured by the TPHC. This time interval equals to the rise time of the input signal, which nearly corresponds to decay time of the detector. If any particle having different energy from 59.5 keV is detected by the detector in this period, the signal of this particle is recorded with a different shape in the TPHC output. Thus, the signals of the 59.5 keV-energy particles can be separated from the other signals. This procedure enables us to improve the energy resolution of the spectrometer in this connection. In other words, since the signals of the 59.5 keV-energy source particles have nearly the same rise times and the signals of the particles with different energies have different rise times, the signal elimination of the latter ones can be possible by this way. After coincidence connection of theenergy signals from the main amplifier with the rise time filtered timing signals from the TPHC, thus, the energy resolution improvement will be possible.
3. Results and Discussion
The spectra, which were acquired in run times of 1,800 s at room temperature of 297 K, were collected in the MCA card where it was divided into 1,024 channels and then stored in a computer. The energy spectrum of the radioisotope from the main amplifier is shown in Fig. 2.The 59.5 keV-energy peak of the isotope was gated via PSA and this peak was dealt with in the measurement to improve the energy resolution of the detector.In Figs. 3a and 3b, the analog signal shape from the main amplifier and the logic output from the TPHC are shown, respectively. Time spectrum from the presented method can be seen in Fig. 4. The time range of the TPHC was adjusted to 200 ns for optimum timing performance.Time resolution of 688 ps was obtained from the time spectrum above. This result was quite effective on the improvement of energy resolution. As it is known, if better time resolution is obtained, better energy resolution improvement can be available.After dealing the 59.5 keV-energy peak in Fig. 2 with the introduced timing method mentioned in the previous section, the final energy spectrum in Fig. 5 was obtained.This spectrum was acquired coincidently by connecting the delayed slow output of the amplifier with the fast timing signal from the TPHC in the MCA. Hence, the MCA has accepted solely the energy spectrum triggered by the timing signals from the TPHC. It can be notable from the figure that the non-peak radiations were discriminated from the 59.5 keV-energy peak.Fig. 6 compares the Figs. 2 and 5.From the comparison in Fig. 6, a decrease in the total counts in the peak area was noticed. Since the PSA was operated in 10-90% interval of the leading edge of its input signal, an output loss occurred on the signals related to this energy region. This output loss normally gave rise to count degradation in the final spectrum.Fig. 7 illustrates the background spectrum of the used detector.From Fig. 5, gross count rate between 42-54 ch.of 1,399.30 s-1 was calculated from the MCA’s software. In addition, background count rate between 10-200 ch.of 0.79 s-1 was found from Fig. 7. It can be expressed from theseresults that the background counts did not affect the final spectrum in Fig. 5 since background counts were too small. Because, furthermore, the count rate from the spectrum in Fig. 5 was below 5,000 s-1 i.e. it was slow count rate[19], it can be concluded that this spectrum did not contain the piled-up signals[19]. The fact that the obtained dead time value (0.83%) from the MCA (Fig. 4) was below the threshold value of 30-40%, moreover, supported this argument[16]. These revealed that the acquired results were reliable.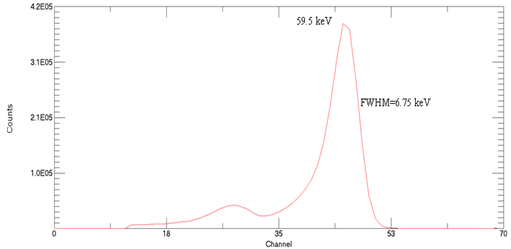 | Figure 2. Obtained energy spectrum of 241Am before timing process |
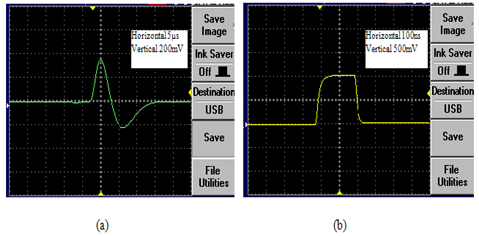 | Figure 3. Signal shapes: (a) Analog bipolar signal shape, (b) logic output |
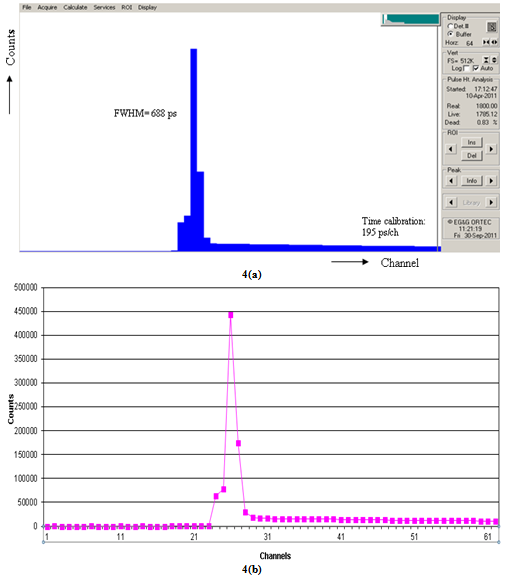 | Figure 4. Time spectrum obtained from the used method: (a) MCA screen view, (b) Graphical view |
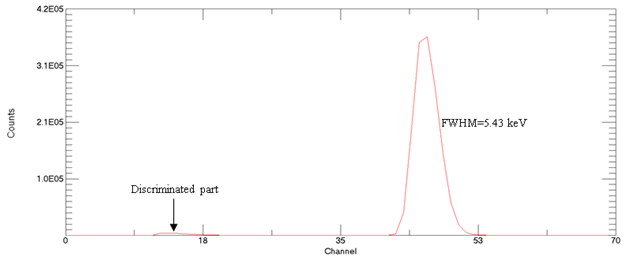 | Figure 5. Final energy spectrum after timing process |
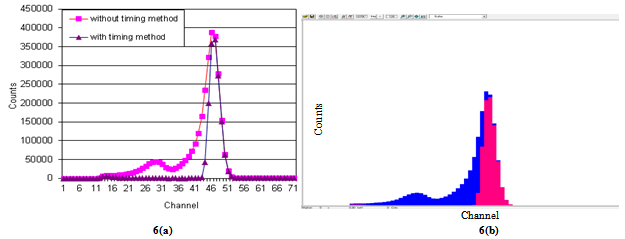 | Figure 6. Comparison of the energy spectra given in Figs. 2 and 5. (a) Graphical view, (b) MCA screen view (Blue: before timing process, Pink: after timing process) |
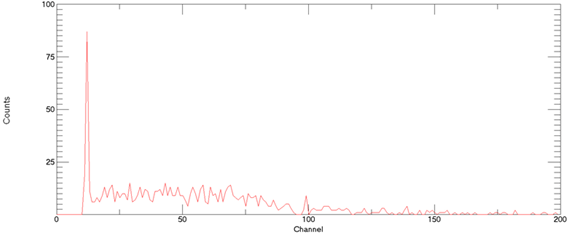 | Figure 7. Background spectrum |
Full width at half maximum (FWHM) value of a peak in an energy spectrum is determined to characterize the energy resolution of a detector as is known. The FWHM value of the detector for 59.5 keV-energy peak in Fig. 2 was calculated by MCA software as 6.75 keV. However, this value was found to be 5.43 keV for the same peak after timing method as shown in Fig. 5. These results showed that a resolution improvement of 24.3% was acquired on the energy spectrum via the used method without cooling the detector. It was believed that better energy resolution would be acquired if cooling of the detector were available.
4. Conclusions
The timing discrimination method was applied to the 59.5 keV-energy peak of an241Am radioisotope to improve the energy resolution of a CdTe detector. Gamma-ray energy spectra with and without timing method were obtained from the developed spectrometer, and the improvement of the resolution was successful without cooling the detector.It was deduced from the experimental results that one of the better ways to increase the energy resolution of a detector was to gate the energy interval to be examined. Moreover, the used timing technique was more successful in the improvement of energy resolution when long data acquisition time was set.It was also concluded from the experimental results that the spectrometer through the timing process described in the present work could be used to improve the energy resolution of any detector.SinceCdTe is one of the commonly used detectors in medical imaging,it was believed that the presented timing method would be helpful in medical imaging process. Furthermore, it is difficult to distinguish the border between healthy tissues and lesions in medical imaging. When the signals from healthy tissues and lesions deal with different signals, they can be distinguished from each other by the timing process, in addition to the energy resolution improvement by means of the denoted method. Moreover, the signal-to-noise ratio (SNR) is quite an important factor in nuclear imaging systems and high SNR values are preferred for clear imaging[21, 22]. Since the electronic noise of the system can be filtered via the used timing method, this method may contribute to better SNR values and fine imaging. The present study was the first step for this purpose. The authors’ future work will concentrate on this subject.
ACKNOWLEDGEMENTS
This work was supported by TUBITAK, the Scientific and Technical Research Council of TURKEY under Project No. TBAG-1683(197T087) and by EBILTEM, Center of Science and Technology, Ege University under Project No. 99 BIL 001. The Authors are indebted to Dr. S. Akdurak for his precious helps.
References
[1] | R.W. Leo, ‘Techniques for Nuclear and Particle Physics Experiments’, Springer–Verlag Berlin Heidelberg, Germany, 1987. |
[2] | J.P. Ponpon, ‘Semiconductor Detectors’ in ‘Experimental Techniques in Nuclear Physics’ Edited by D.N. Poenaru and W. Greiner, Walter de Gruyter, Berlin, 1997. |
[3] | M.H. Ali and P. Siffert, ‘Semiconductors for Room Temperature Nuclear Detector Applications’ Academic Press, San Diego, California, 1995. |
[4] | E. Meyer, M. Martini, J. Sternberg, ‘Measurement of the Disappearance Rate of 75-Se Sodium Selenite in the Eye of The Rat by a CdTe Medical Probe’, IEEE Trans.Nucl. Sci.,vol. 19, no. 3, pp. 237-243, 1972. |
[5] | Y. Eisen, A. Shor, I. Mardor, ‘CdTe and CdZnTe Gamma Ray Detectors for Medical and Industrial Imaging Systems’,Nucl. Instr. Meth. A vol. 428, no. 1, pp. 158-170, 1999. |
[6] | C. Scheiber, ‘CdTe and CdZnTe Detectors in Nuclear Medicine’, Nucl. Instr. Meth. A,vol. 448, no. 3, pp. 513-524, 2000. |
[7] | C. Scheibersnd G.C. Giakos ‘Medical Applications of CdTe and CdZnTe Detectors’, Nucl. Instr. Meth. A,vol. 458, no. 1-2, pp. 12-25, 2001. |
[8] | K. Ogawa, ‘Feasibility Study on an Ultra-High-Resolution SPECT with CdTe Detectors’, IEEE Trans.Nucl. Sci., vol. 57 no. 1, pp. 17-24, 2010. |
[9] | E. Abuelhia, K. Alzimami, M. Alkhorayef, Z. Podolyak, N.M. Spyrou, ‘Measurement of Coincidence Timing Resolution of Scintillation Detectors Compared to Semiconductor Detectors to Image Three-Photon Positron Annihilation’, J.Radioanal.Nucl. Chem., vol. 278, no. 3, pp. 767-771, 2008. |
[10] | L.T. Jones and P.B. Woollam, ‘Resolution Improvement in CdTe Gamma Detectors using Pulse Shape Discrimination’, Nucl. Instr.Meth., vol.124, no. 2, pp.591-595, 1975. |
[11] | M.Richter and P. Siffert, ‘High Resolution Gamma Ray Spectroscopy with CdTe Detector Systems’, Nucl. Instr.Meth. A, vol.322, no. 3, pp.529-537, 1992. |
[12] | L. Abbene, G. Gerardi, S. Del Sordo, G. Raso, ‘Performance of a Digital CdTe X-ray Spectrometer in Low and High Counting Rate Environment’, Nucl. Instr.Meth. A, vol.621, no. 1-3, pp.447-452, 2010. |
[13] | L. Abbene and G. Gerardi, ‘Performance Enhancements of Compound Semiconductor Radiation Detectors Using Digital Pulse Processing Techniques’, Nucl. Instr. Meth. A,vol. 654, no. 1, pp. 340-348, 2011. |
[14] | M. Richter and P. Siffert, ‘High Resolution Gamma Ray Spectroscopy with CdTe Detector Systems’, Nucl. Instr. Meth.A , vol. 322, no. 3, pp. 529-537, 1992. |
[15] | C. Bargholtz, E. Fumero, L. Martensson, ‘Model-based Pulse Shape Correction for CdTe Detectors’, Nucl. Instr. Meth. A, vol. 434, no. 2-3, pp. 399-411, 1999. |
[16] | G.F Knoll, ‘Radiation Detection and Measurement’, John Wiley and Sons, New York, 2000. |
[17] | S. Martoiu andA. Rivetti, ‘Electronics for Radiation Detection’, CRC Press, Boca Raton, 2011. |
[18] | http://www.ritec.lv Accessed 01.11.2013 |
[19] | T.J. Paulus, ‘Timing Electronics and Fast Timing Methods with Scintillation Detectors’, IEEE Trans.Nucl. Sci., vol. 32, no. 3, pp. 1242-1249, 1985. |
[20] | N. Tsoulfanidis, ‘Measurement and Detection of Radiation’, Taylor and Francis, Washington, 1995. |
[21] | S.Y. Molloi and C.A. Mistretta, ‘Quantification Techniques for Dual-energy Cardiac Imaging’, Med. Phys., vol 16, no. 2, pp. 209-217, 1989. |
[22] | S. Molloi, A. Ersahin, Y.J.Qian, ‘CCD Camera for Dual-energy Digital Subtraction Angiography’, IEEE Trans. Med.Imag., vol. 14, no. 4, pp. 747-752, 1995. |