Turgunov Jalaluddin Rahmonali Son 1, Naraliyeva Nasibakhan Mamanovna 2
1Andijan Institute of Agriculture and Agrotechnologies, Uzbekistan
2Doctor of Biological Sciences, Andijan State University, Uzbekistan
Copyright © 2024 The Author(s). Published by Scientific & Academic Publishing.
This work is licensed under the Creative Commons Attribution International License (CC BY).
http://creativecommons.org/licenses/by/4.0/

Abstract
In recent years, studies have been conducted on the effects of drought on specific characteristics of Momordica charantia L. gene expression and metabolic changes in Momordica charantia L. seedlings in response to low temperature stress. However, despite the fact that abiotic stresses such as drought, temperature extremes, salinity, and heavy metals can reduce plant growth and productivity by more than 50%, the presence of tolerance genes in the genome of M. charantia not enough studies have been conducted. For this reason, our research is aimed at studying the presence of 12 different tolerance genes in the medicinal plant M. charantia acclimatized in Uzbekistan. Selected genes namely CSP3, CYP707A, GST, PYL4, BCH, POD1, RBOH, CAT, GR, PP2C78, PP2C8 and SOS2 stress tolerance including cold tolerance, drought tolerance, salinity tolerance, pathogens and cadmium known to impart various properties related to resistance to heavy metals such as. Understanding the presence of these stress tolerance genes is essential to elucidate the genetic adaptation of M. charantia to withstand multiple abiotic stresses.
Keywords:
Momordica charantia, Abscisic acid, Setiltrimetil ammonium bromid, NanoDrop spektrofotometri
Cite this paper: Turgunov Jalaluddin Rahmonali Son , Naraliyeva Nasibakhan Mamanovna , Determination of Bitter Melon (Momordica Charantia L.) Genes Resistant to Different Stress Factors, International Journal of Virology and Molecular Biology, Vol. 13 No. 2, 2024, pp. 32-35. doi: 10.5923/j.ijvmb.20241302.03.
1. Introduction
Momordica charantia L belongs to the Cucurbitaceae family and is a popular and widespread vegetable in Southeast Asia due to its edible and medicinal properties. This plant contains a lot of minerals, vitamins C and E, etc. [1]. It is also used to modulate blood glucose and treat diabetes in our daily life [2]. M. charantia contains important phenolic compounds that contribute to its antioxidant, antibacterial, and anti-inflammatory properties [3], and is used against gastric diseases, hypertension, obesity, and cancer [4].Due to the adverse effects of the environment, the growth and productivity of plants decreases. The sensitivity of plants to drought is a complex process that depends on various physiological and biochemical aspects. Prolonged drought stress damages photosynthetic processes, particularly chlorophyll pigments [5]. To combat this adverse abiotic stress, plants employ strategies such as producing abscisic acid (ABA) and accumulating suitable molecules such as s ugars and proline. They also develop enzymatic and non-enzymatic antioxidant systems to protect cells from oxidative damage [6].Low temperature is the main abiotic factor limiting plant germination, growth and spread [7,8]. When exposed to low temperature stress, plants usually develop various physiological and biochemical changes and modulate gene expression to achieve cold adaptation, for example, antioxidant enzymes produce antioxidants and osmotic solutions [9,10].Cadmium (Cd) is widely recognized as one of the most harmful heavy metals in soil. Acute toxicity, water solubility, non-degradability and extremely dangerous pollutant for living organisms. Studies have shown that Cd is more readily absorbed by many plant species than other heavy metals. Excessive accumulation of Cd2 + in plant tissues can cause serious phytotoxicity and have various negative effects on plant growth and development. These effects include destroying the chlorophyll structure of leaves, stopping photosynthesis and respiration, disrupting the absorption and translocation of mineral nutrients, accumulation of reactive oxygen species (ROS), suppressing protein synthesis and enzymatic activity, growth attenuation stops root growth [11].The main antioxidant enzymes that play a crucial role in scavenging reactive oxygen species (ROS): CAT (Catalase), SOD (Superoxide dismutase), PPO (Polyphenol oxidase), GPOD (Guaiacol peroxidase) and APOX (Ascorbate peroxidase) [12].
2. Material and Methods
Plant Materials: Momordica charantia L is a plant belonging to the Cucurbitaceae family. Freshly grown plant samples were used. Samples were taken from undamaged leaves. Molecular research methods were used in the research. CTAB (cetyltrimethylammonium bromide) method is carried out in the following order.Stage 1. We measure 30 mg of plant leaf tissue on a scale, grind it in a mortar and add 200 µl (600 µl - 1000 µl) of STAB solution kept in a water bath and mix well.Stage 2. We put 0.5 ml of the homogenate into epindorffs, and then put it in a thermostat at 65°C for 90 minutes with occasional shaking (inverted).Stage 3. 600 µl of 24:1 mixture of chloroform(CHCl3)/ isoamyl(C5H12O) alcohol is added. Mix the homogenate for 5 sec. we catch in the vortex. 10 min. We put it in a centrifuge at a speed of 12000. In this, 3 different layers are formed, and DNA occupies the topmost layer.Step 4. Using a pipette, transfer 400 µl of the supernatant to a 1.5 ml Epindorf.Step 5. Repeat steps 3-4.Step 6. Add 40 µl of cold 3M sodium acetate (pH 5.2) and 400 µl of chilled isopropyl alcohol and shake several times. In this case, the DNA begins to sink down.Step 7. The supernatant is incubated in a refrigerator at -20°C for 3 hours (or left overnight).Step 8. The supernatant is removed from the refrigerator and centrifuged at 14,000 rpm for 10 min. DNA precipitates white. The part of the supernatant up to the DNA is removed.Step 9. To wash the DNA, add cold 70% ethanol in a volume of 900 µl and put it in a centrifuge for 15 min at a speed of 14,000. In this case, the DNA remains as a precipitate.Step 10. Ethanol is removed. 37°C for 20 min. is left open. DNA does not need to be overstretched.Step 11. Separated DNA is dissolved in 100 µl of TE buffer (pH=8), slightly heated and the concentration in the nanodrop is checked.
3. Result
In recent years, the effects of drought on the characteristics of Momordica charantia L cultivars [13], researches were conducted on gene expression and metabolic changes of Momordica charantia L. seedlings in response to low temperature stress [14]. However, despite the fact that abiotic stresses such as drought, sudden temperature changes, salinity, and heavy metals can reduce plant growth and productivity by more than 50% [15,16], The presence of tolerance genes in the genome of M. charantia has not been sufficiently studied.For this reason, our research is aimed at studying the presence of 12 different tolerance genes in the medicinal plant M. charantia acclimatized in Uzbekistan. Selected genes namely CSP3, CYP707A, GST, PYL4, BCH, POD1, RBOH, CAT, GR, PP2C78, PP2C8 and SOS2 stress tolerance including cold tolerance, drought tolerance, salinity tolerance, pathogens and cadmium known to impart various properties related to resistance to heavy metals such as . Understanding the presence of these stress tolerance genes is essential to elucidate the genetic adaptation of M. charantia to withstand multiple abiotic stresses. Through DNA extraction and PCR amplification methods using specific genetic markers, we aimed to determine the presence of these tolerance genes in plant samples from Uzbekistan. The identification of these stress-related genes demonstrates the genetic adaptation of M. charantia to withstand salinity stress and multiple abiotic stresses common in its native environment. In addition, it provides valuable insights to support the acclimatization and cultivation practices of this economically important medicinal species and to exploit the medicinal properties of the plant.
4. Discussion
Further characterization of genes expressing these stress tolerances will contribute to a comprehensive understanding of the genetic basis of stress tolerance in M. charantia. Our research may help develop strategies for sustainable cultivation and use of this medicinal plant, increasing its economic value and improving its medicinal properties. DNA was extracted from fresh leaves of M. charantia acclimatized in Andijan, Uzbekistan. Ensuring the reliability of experimental data, only undamaged and disease-free leaves were selected for further analysis. DNA was isolated from 20 mg of young leaf tissue using a modified CTAB (cetyltrimethylammonium bromide) method. This extraction process involves homogenizing the samples into a fine powder. The resulting powder was subjected to a series of steps including CTAB extraction, incubation, chloroform/isoamyl alcohol extraction, DNA precipitation and purification. The quality and quantity of the obtained DNA was evaluated using a NanoDrop spectrophotometer, and the suitability of the samples for further analysis was ensured (Fig. 1).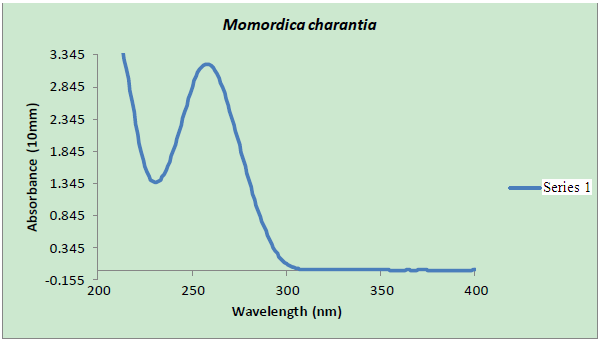 | Figure 1. The result of testing the quality and quantity of DNA extracted using the NanoDrop spectrophotometer. Concentration) 157.80 ng/ul, A260/A280) 2.289, A260/A230) 2.345 |
Polymerase chain reaction (PCR) was performed to target and amplify 12 stress tolerance genes (Table 1) in the extracted DNA. PCR reaction mixtures consisting of sample DNA, PCR buffer, MgCl2, dNTPs, specific forward and reverse primers, and Taq DNA polymerase were prepared. The resulting PCR products were analyzed by gel electrophoresis, a 1.5% agarose gel was prepared and loaded with PCR products. Electrophoresis was performed to separate DNA fragments according to their size, and the presence and size of DNA fragments were determined using UV light. This data was analyzed to determine the genetic adaptation of M. charantia to abiotic stresses by assessing the presence of stress tolerance genes (Figure 2).Table 1. Molecular markers expressing genes responsible for response to various Stresses 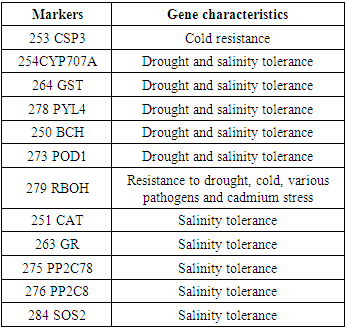 |
| |
|
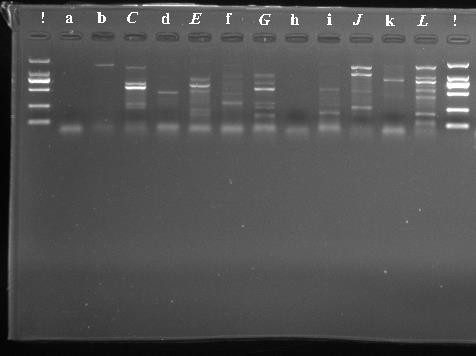 | Figure 2. The result of detection of the presence and size of DNA fragments using UV light.) Ladder, A) 250 BCH, B) 251 CAT, C) 253 CSP3, D) 254CYP707A, E) 263 GR, F) 264 GST, G) 273 POD1, H) 275 PP2C78, I) 276 PP2C8, J) 278 PYL4, K) 284 SOS2, L) 279 RBOH |
Clear bands were observed for 5 of the 12 markers analyzed – CSP3, GR, POD1, PYL4, RBOH (Figure 2) – indicating their presence in M. charantia samples. No separate bands were detected for the remaining 7 markers. The presence of bands for CSP3, GR, POD1, PYL4, and RBOH markers indicates that the analyzed M. charantia samples possess these genetic traits associated with stress tolerance. CSP3 increases cold tolerance by stabilizing proteins and membranes. GR and POD1 play important roles in scavenging ROS during drought and salinity stresses [17]. PYL4 and RBOH are involved in abscisic acid-dependent stress signaling and response pathways [18]. Our study provides the first evidence that M. charantia can be uniquely tolerant to abiotic stresses such as cold, drought, and salinity. However, further investigation of marker function and assessment of stress tolerance under controlled conditions is also necessary. The absence of bands for the other 7 markers may be due to primer mismatch or the absence of corresponding markers in this M. charantia plant.253 The presence of the CSP3 marker indicates that M. charantia has genetic adaptation for cold tolerance. Cold stress is an important abiotic stress that can adversely affect plant growth and development. The presence of this sign indicates that M. charantia has mechanisms to withstand and adapt to low temperatures. These genetic adaptations may include activation of cold-sensitive genes, changes in membrane composition to maintain membrane fluidity, and accumulation of sugars and proteins.The presence of the 263 GR marker indicates that M. charantia exhibits characteristics related to salinity tolerance. Salinity stress caused by high salt concentrations in the soil can inhibit plant growth and productivity. The presence of this trait suggests that M. charantia has genetic adaptations that allow it to thrive in saline environments.273 The presence of the POD1 marker indicates that M. charantia has genetic adaptations to both drought and salinity. Drought stress and salinity stress, characterized by water deficit, often occur together in many environments, and plants with combined tolerance mechanisms are more likely to survive. The presence of this trait indicates that M. charantia has genetic traits that allow it to tolerate both water deficit and high salinity conditions. These traits may include efficient water use strategies such as stomatal regulation and enhanced water retention, as well as activation of stress-responsive genes involved in osmotic adaptation and antioxidant defense.Similarly, the presence of 278 PYL4 markers suggests that M. charantia has genetic adaptation to both drought and salinity. This indicates that the plant has genetic mechanisms to withstand water shortage and high salt concentration in the environment. These mechanisms may include the activation of stress –responsive genes involved in regulation of the stomatal diaphragm, maintenance of water potential, and synthesis of osmoprotectants and detoxification of reactive oxygen species.In addition, the presence of 279 RBOH markers indicates that M. charantia exhibits genetic adaptations for resistance to multiple stresses. This marker indicates the presence of genetic traits related to drought tolerance, cold tolerance, resistance to various pathogens and cadmium stress. Drought stress can be mitigated by mechanisms such as increasing water use efficiency, altering root architecture, and activating stress-responsive genes involved in water conservation and osmotic adjustment. Features of cold tolerance may include expression of cold-responsive genes, accumulation of antifreeze proteins, and changes in lipid composition to maintain membrane integrity. Resistance to pathogens can be ensured by activation of defense-related genes, synthesis of antimicrobial compounds and modulation of plant immune system. Finally, resistance to cadmium stress may involve activation of detoxification pathways, up -regulation of genes related to heavy metal detoxification.
5. Conclusions
Overall, the presence of these specific markers in gel electrophoresis results provides strong evidence for genetic adaptations and stress tolerance mechanisms present in M. charantia. These findings shed light on the plant's ability to thrive under harsh environmental conditions and may have important implications for increasing crop resilience and improving the sustainable cultivation of medicinal plants in adverse environments. Further study of these genetic traits and their underlying mechanisms will ensure the creation of stress -resistant crop varieties and the preservation of valuable plant species. This study shows for the first time that 253 CSP3, 263 GR, 273 POD1, 278 PYL4 and 279 RBOH specific genetic markers associated with abiotic stress tolerance are present in M. charantia using gel electrophoresis. Identification of these characteristics will provide an opportunity to develop stress-resistant varieties of M. charantia through marker-assisted breeding for sustainable cultivation under adverse conditions. Future work will include marker validation and assessment of stress response.
References
[1] | Dhillon NPS, Lin C-C, Sun Z, Hanson PM, Ledesma DR, Habicht SD, et al. Varietal and harvesting stage variation in the content of carotenoids, ascorbic acid and tocopherols in the fruit of bitter gourd (Momordica charantia L.). Plant Genetic Resources-Characterization and Utilization. 2017; 15(3): 248– 59. https://doi.org/10.1017/s147926211500057x WOS:000402150000006. |
[2] | Saini R, Rai PK, Bara BM, Sahu P, Anjer T, Kumar R. Effect of different seed priming treatments and its duration on seedling characters of Bitter gourd (Momordica charantia L.). J Pharmacogn Phytochem. 2017; 6(5): 848–50. |
[3] | Singh A, Gupta A, Srivastava S, Choudhury B, Jain S, Bajpai A. Assessment its Antioxidant, Antimicrobial and Anti-Inflammatory Potential of Momordica Charantia. Biomed Pharmacol J. 2023; 16(2): 899–906. Available at http://doi.org/10.13005/bpj/2672. |
[4] | Jia S, Shen M, Zhang F, Xie J. Recent Advances in Momordica charantia: Functional Components and Biological Activities. IJMS. 2017; 18(12): 2555. Available at: http://doi.org/10.3390/ijms18122555. |
[5] | Nayyar H, Gupta D. Differential sensitivity of C3 and C4 plants to water deficit stress: association with oxidative stress and antioxidants. Environmental and Experimental Botany, 2006: 58(1-3): 106-13. |
[6] | Mittler R. Oxidative stress, antioxidants and stress tolerance. Trends in plant science, 2002: 7(9): 405-10. |
[7] | Wani MA, Jan N, Qazi HA, Andrabi KI, John R. Cold stress induces biochemical changes, fatty acid profile, antioxidant system and gene expression in Capsella bursa pastoris L. Acta Physiologiae Plantarum. 2018; 40(9). https://doi.org/10.1007/s11738-018-2747-z WOS:000443060500003. |
[8] | Peng M, Chang Y, Chu G, Wang M. Low-temperature tolerance and transcriptome analyses during seed germination of Anabasis aphylla. Journal of Plant Interactions. 2019; 14(1): 254–64. https://doi.org/10.1080/17429145.2019.1616840 WOS:000468969900001. |
[9] | Barrero-Gil J, Huertas R, Luis Rambla J, Granell A, Salinas J. Tomato plants increase their tolerance to low temperature in a chilling acclimation process entailing comprehensive transcriptional and metabolic adjustments. Plant Cell and Environment. 2016; 39(10): 2303–18. https://doi.org/10.1111/pce.12799 WOS:000385846100015. PMID: 27411783. |
[10] | Li S, Yang Y, Zhang Q, Liu N, Xu Q, Hu L. Differential physiological and metabolic response to low temperature in two zoysiagrass genotypes native to high and low latitude. Plos One. 2018; 13(6): e0198885. https://doi.org/10.1371/journal.pone.0198885 WOS:000434786600062. PMID: 29889884. |
[11] | Song X, Yue X, Chen W, Jiang H, Han Y and Li X (2019) Detection of Cadmium Risk to the Photosynthetic Performance of Hybrid Pennisetum. Front. Plant Sci. 10:798. doi: 10.3389/fpls.2019.00798. |
[12] | Sgherri CL, Maffei M, Navari-Izzo F. Antioxidative enzymes in wheat subjected to increasing water deficit and rewatering. Journal of Plant Physiology, 2000:157(3): 273-9. |
[13] | Jayaraj, M. S., and S. Suhara Beevy (2021) "Impact of drought on the characteristics attributes in the varieties of Momordica Charantia L." International Journal of Botany Studies; Volume 6, Issue 3, 2021, Page No. 125-131. |
[14] | Niu Y, Liu Z, He H, Han X, Qi Z, Yang Y (2020) Gene expression and metabolic changes of Momordica charantia L. seedlings in response to low temperature stress. PLoS ONE 15(5): e0233130. https://doi.org/10.1371/journal.pone.0233130. |
[15] | Khan, M. I. R., Fatma, M., Per, T. S., Anjum, N. A., & Khan, N. A. (2015). Salicylic acid-induced abiotic stress tolerance and underlying mechanisms in plants. Frontiers in plant science, 6, 135066. |
[16] | Minhas, P. S., Rane, J., Pasala, R. K. (2017). “Abiotic stresses in agriculture: an overview,” in Abiotic stress management for resilient agriculture (Singapore: Springer), 3–8. |