Frans De Medts, TU Eindhoven
University of Technology, Eindhoven, 5600MB The Netherlands
Correspondence to: Frans De Medts, University of Technology, Eindhoven, 5600MB The Netherlands.
Email: |  |
Copyright © 2014 Scientific & Academic Publishing. All Rights Reserved.
Abstract
Georges Lemaitre was invited to London in 1931 in order to take part in a meeting of the British Association on the relation between the physical Universe and spirituality. There he proposed that the Universe expended from an initial point, which he called the ‘Primeval Atom’ and developed in a report published in Nature: ‘The beginning of the World from the Point of View of Quantum Theory’. [1] This article tries to make clear how the way in which the universe is supposed to have come into being directs (and can therefore be the deeper cause of) all quantum mechanical events. On the basis of hidden symmetries a‘smallest space-action’ (or ‘smallest limited amount of uncertainty’) is established, which provides a connection between all that is and which lays down the boundaries within which all variation is possible; unity in diversity then.
Keywords:
The Smallest Space-Action
Cite this paper: Frans De Medts, TU Eindhoven, A Phenomenological Relation for Decay Times, International Journal of Theoretical and Mathematical Physics, Vol. 4 No. 4, 2014, pp. 151-155. doi: 10.5923/j.ijtmp.20140404.02.
1. Introduction
The following is a quotation from a lecture held by Max Planck at the Berlin Academy on 29 June 1922 during a day that was organised in honour of Leibniz. Planck drew attention to a very special work of that great scientist:“Modern science, as far as it has been theoretically organised, is described in full by a system of space-time differential equations that states that every process in nature is completely determined by the events that take place in the immediate space-time surroundings of the process. This whole complex system of differential equations has now been summarised in just one proposition, the principle of the smallest action, even though the differential equations differ in detail as they are concerned with processes in mechanics, electricity, magnetism, and theory of heat. In short this principle boils down to the idea that, of all possible processes, the only processes that actually occur are those that display a minimum of total action. In modern science the principle of the smallest action plays a relatively small role. It does not fit in very well with current theories. I admit that this is of course a correct assertion; still this principle in general does not serve as the basis of the theory, but as a correct and in disposable addition, because modern theoretical science is completely focused on the principle of infinitesimal local effects, and because it views the expansion of observations to larger spaces and times as an unnecessary and uneconomical complication of the method of treatment. It is for this reason that science is inclined to consider the principle of the smallest action as a formal and coincidental curiosity instead of as a cornerstone of physical knowledge. Therefore it has been all the more surprising that Hermann von Helmholz found this principle, which was originally considered to be a thesis from mechanics by Leibniz and Maupertius, to be valid for the whole science of his time without any restriction. Recently, David Hilbert, using Hamilton’s version of the above-mentioned thesis, has verified the principle by means of Einstein’s general theory of relativity. As the circumstances become more complicated, it becomes less likely that the dominant position of such a simple law can be a mere coincidence. It is indisputable that a method is incomplete if it cannot explain a universally accepted, simple and general relation. It is only when the law that has been proven to be correct is understood in its complete meaning and relevance, and when it is integrated in the whole of the theoretical system, that our desire for comprehensiveness will be fulfilled.”
2. Discussion
Einstein, Podolsky and Rosen protected the view that the wave function does not provide a complete physical description of reality and that a higher, complete theory is possible. [2-3] Several claims in some works from the 1960s were placed on higher descriptions given in terms of hidden variables. [4-5].Very recently, Roger Colbeck and Renato Renner have presented an argument to show that ‘a system’s wave function is in one-to-one correspondence with its elements of reality’. [6]They have also recently shown that, under the assumption of free choice of measurement settings, if quantum theory is correct then it is non extendible, in the sense of being maximally informative about measurement outcomes. [7]Pusey, Barrett and Rudolph have placed the subjective interpretation of the wave function (‘a subjective state of knowledge about some underlying reality’, such as the Copenhagen interpretation), into doubt [8]. In other words, quantum events have a clear cause, namely the way in which the universe has come into being. This first quantum mechanical event in specific, which corresponds to the same mathematical pattern as all quantum events, also provides a different outlook on the concepts of complementarity and quantum mechanical entanglement. In his book ‘Einsteins Schleier’ [9] Zeilinger writes: “As early as 1935, soon after the article by Einstein, Podolsky and Rosen, Niels Bohr claimed that the entangled particles, regardless of the distance between them, should be considered as a unit or as one single system. The entangled particles do therefore not exist independently of each other.” That is, it is not because Heisenberg’s uncertainty relation can be considered as an absolute uncertainty and because, for instance, place and impulse of an atomic particle in a quantum physical event can never be known accurately at the same time as a result, that such an event could not correspond to a rudimentary element, a minimum principle or smallest action, that shows itself in the form of a hidden symmetry. In other words, ‘chance’ in the sense of ‘unpredictable’ exists, but that does not mean at all that such a chance does not have a deeper cause. For instance: the entangled particles in Zeilinger’s tests should, in my opinion, correspond to a quantised action (space-action), and this also explains why both particles behave so unusually to the observer during measuring. Nobel Prize Winner Gerard ‘t Hooft writes in a recent article entitled ‘Quantum mechanics only leads astray’ [10] that a theory of everything can only be about certainties: “If you would know of all dynamic variables in a system the exact initial situation, the final situation should only take on one form – not a distribution of probability.” He asks himself if a universal equation of movement will be completely determining for all events in the universe, or that it, as we are used to in quantum mechanical theory, will only yield distributions of probability of everything that can happen. Gerard ‘t Hooft says furthermore that according to currents views it is fundamentally unpredictable when an individual radioactive atom decays. He clarifies: “The theory only includes the chance per unit of time that it will decay (and describes very accurately how fast a macroscopic amount of radioactive material decays).”“Most of my colleagues”, as ‘t Hooft claims, “think that all of nature, including the most fundamental laws to which all phenomena can be traced back, is fundamentally quantum mechanical. My suspicion is that not nature, but our current knowledge of it, is quantum mechanical. The laws that we know, produce only distributions of probability, because we do not (yet?) know the actual laws.”According to ‘t Hooft the reality of nature consists of little cogs that are ‘billions of times’ smaller than the quantum structure we now know. “The collective conduct of these cogs”, as ‘t Hooft states, “allows for us to talk about atoms and electrons in a quantum mechanical language, but the conduct can never be separated from what happens at a much smaller scale. This could be a natural explanation of the remarkable phenomena we call ‘quantum mechanics’.”Physicist Murray Gell-Mann (in collaboration with the Santa Fe Institute) gives an account of the ‘connection of things’ in his book ‘The Quark and the Jaguar. Adventures in the Simple and the Complex’ [11]. He makes clear that a smallest action needs to be at the base of everything and connects everything with everything. He claims: “We will have to view fundamental physics from the perspective of simplicity and complexity and ask ourselves which roles are fulfilled by the unified theory of the elementary particles, the initial situation of the universe, the indefiniteness of quantum mechanics and the quirks of classical chaos when establishing patterns of regularity and arbitrariness in the universe in which complex adaptive systems have had to develop”. In the book ‘Variational Principles in Dynamics and Quantum Theory’ by Wolfgang Yourgrau and Stanley Mandelstam [12] the importance of minimum principles is pointed out, and the Fermat principle of least time, as well as the Maupertius principle of smallest action (Lorsqu’il arrive quelque changement dans la Nature, la quantité d’action, nécessaire pour ce changement, est la plus petite qu’il soit possible”) and the development of this principle by Euler and Lagrange etc., are discussed. The authors refer to the fact that Helmholtz, too, and on the basis of absolutely scientific grounds, assumed that the principle of smallest action ought to be considered as a unifying law of nature, which dominates the whole of physics. Furthermore R. Feynman takes the view that symmetry principles and conservation principles can only be interrelated if the principle of smallest action applies.
3. Claim
The underlying mathematical part of this article subscribes to the propositions of physicists Einstein, Gell-Mann and ‘t Hooft concerning the need for a deeper substructure. That is, the article goes in search of this smallest action in a concrete manner. Here, too, the starting points are the notions of symmetry and symmetry breaking. Subsequently, they will be linked up with the minimum principle in nature on a quantum physical level. As it is of paramount importance to understand the value of the frequent hidden or broken symmetries in nature, the following ‘balance concept’ is presented as a specific and central instantiation of hidden symmetry. Within relativistic mechanics (1) it is stated that for the kinetic energy of a particle that moves with a velocity v in relation to the observer, the following applies: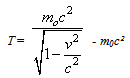 | (1) |
Fundamentele Natuurkunde - Mechanica (p.245) Alonso, M. & Finn, E. J. 1978m0 = mass at rest in relation to the observerv = velocityc = velocity of lightT = added energy in the form of movement energy to the mass at restIt is said that this outcome is very suggestive and that it indicates that the increase in kinetic energy can be viewed as an increase in mass. This idea is based on experiments that are intended to give evidence that the mass of a particle that moves with velocity v in relation to the observer is shown by:
The formula for a particle’s kinetic energy or the added energy to the mass at rest can however be described and interpreted differently: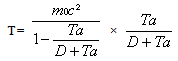 | (2) |
(2) T is completely written in function of space shrinkage or ‘shrunken space’ as a representation of energy; and impulse
can be written as
. In other words, the excess value of the impulse can just as well refer to ‘space shrinkage’ instead of to mass.When we consider the forces of the levers as weights or masses we can translate each mass into an amount of movement energy (by E=Mc²), which we can add to the opposite mass. We can calculate that that mass in this case has exactly such an amount of velocity that its matching lever arm, (in accordance with the characteristics of special relativity theory à that is, for the moving system of reference the unit for linear measurement is larger), corresponds to space shrinkage. In other words, for the moving masses the lengths of their own lever arms have become non-existent, when the distance between the edges of the lever arms within the systems of reference of these masses is measured. (Fig 1-De Medts F.)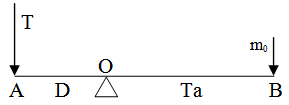 | Figure 1. |

orT × D = m0 × Tav = velocityc = velocity of lightT = added energy to the mass at rest (m0)D = ‘real’ distance from the lever arm (remaining distance for the moving system of reference)m0 = mass at restTa = space shrinkage
In this argument mB moves that fast that, when bridging the distance from A to B, OB is converted into space shrinkage. The added energy T which is needed for this, in terms of mass (mA), corresponds exactly to the mass needed to keep the balance balanced. The question that arises immediately is to what extent this reasoning has a physical meaning. It appears to have this meaning indeed when we apply the idea to the excited hydrogen atom and to all processes of decay. Quantum physics tries to explain quantum leaps of electrons and other elementary particles. However, very variable times of decay are known to exist depending on whether the electromagnetic force, the weak force, the strong force or the gravity is responsible for the decay. Whereas the decay time for Planck mass is the Planck time of 10-43 sec, for resonances an approximate time of 10-23 sec is recorded, for a lot of radioactive decay ≈ 10-12 sec, for the excited hydrogen atom 10-10 sec, for the muon 10-6 sec, for the neutron almost 15 min and for stable particles such as the proton many billions of years. Is it possible to distinguish some sort of regularity in the average values of these so very divergent times of decay? In order to provide an answer to this question, one can take the formula for the Planck constant, which can be transformed into 2,0642105 x 1026
x Planck length =
, as a starting point. For, to express the Planck mass in terms of a number of spin-action units, we can (for the unit system) multiply the Planck mass with 1m²/sec² and divide it by
.Consequentially the following phenomenological relation is proposed:
The right part of the equation is the constant and the left part represents every quantum physical event or every spin-orbit interaction of the subparticle within the decay time of the excited system. In the above equation,
s stands for the number of spin-action units per sec² of the subparticle that effects the decay of the system, vs for the subparticle’s estimated velocity, Tv S for the excited system’s time of decay, h for the Planck constant and c for the velocity of light.To balance the unit system we can multiply the second part of the equation by 1m²/sec² in this case (given the followed reasoning). In the excited hydrogen atom the electron serves as the subparticle that effects the decay: it acts as a virtual particle that gives us an idea of the nature and the intensity of the electron wave. The hydrogen atom takes up exactly the amount of photon energy needed to have the electron jump from its ground state to the excited state (so that the difference in distance from the electron orbits to the nucleus corresponds to space shrinkage). If we want special relativity to be applicable, this movement lies outside the horizon of observation. At that point the subparticle starts a spin-orbit movement equivalent to the variation principle. (The electron rotates around the proton at a high velocity until the difference in distance between the circumferences of both orbits is converted into space shrinkage). All of this happens exactly during the decay time and so within our horizon of observation. Afterwards the photon is radiated. A similar situation occurs in the radioactive decay. Here the subparticle is a quark. It can be noted that in the case of a high increase in energy of a quark to another type of quark in excited state, the life span of the unstable particle is short and all the more energy goes to the orbit movement. When we next consider the decay of the neutron, we notice that the total increased energy of the neutron in relation to the proton, is only slightly more than the value of two electrons. The life span is approximately 918 sec and we can assume that this energy as ‘space-action’ goes to the spin movement. For the rest, the effective masses of up quark and down quark are more or less the same, while their naked masses differ only by a few electrons. We also know that the ratio –2/3 for the magnetic moment for nucleons is based on the fact that it is only the spins of the quarks that contribute to the total angular momentum. For resonances the responsibility for the decay lies with the particle’s oscillating orbit, which corresponds to a spatial movement of 4π times the wavelength of the particle. Their average life is therefore very short.Table 1. Compound on the base of data from: ‘t Hooft, G. , De Bouwstenen van de Schepping (2014). Tables: p.41, 42, 148, 160 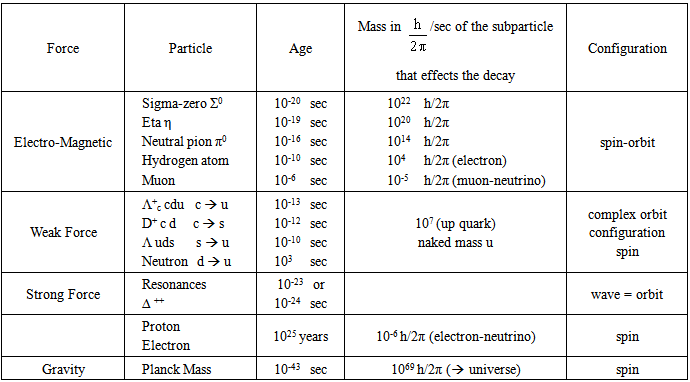 |
| |
|
The so-called stable particles on the other hand exist for a very long time and the ‘space-action’ goes entirely to the spin movement. In the proton and the electron the particle responsible for the decay can be a neutrino. For the muon the spin-orbit interaction of a muon neutrino can fulfil the ‘space-action’ exactly within the decay time.Then there are the particles that have a very short life span and that decay on the base of electromagnetic force, such as sigma-zero, eta and the neutral pion. We assume that heavy particles are responsible for the decay of these particles. Taking into consideration that the uncertainty principle has to be a fundamental characteristic of the universe in which we live and that the Planck mass occurs on such a deep level in physics, a successful unification theory needs to contain both the Planck mass and this uncertainty principle. Respecting the relation between the hierarchy principle, the fine-tuning problem and the problem of the cosmological constant, there has to be a certain principle that chooses one initial situation and therefore there has to be one model that represents our universe. The question that presents itself then is whether this is a smallest event or a minimum principle on the level of quantum physics. That is, this phenomenological relation can be considered as the ‘smallest space-action’, expressed in terms of the units energy x distance or 10-43 x 3,5179 kgm³/sec².
4. Final Conclusions
When we apply the minimum principle to the decay of the Planck mass, we come to the remarkable conclusion that decay can only take place by a mass that refers us to the energy capacity of the universe. Arguments in connection with Alan Guth’s inflation theory makes this a credible idea. During the decay of the Planck mass the energy capacity of the universe would be released as a result of the immense pressure. In inflation theory a state that can assume the role of ‘false vacuum’ is then called for. As appears from general relativity theory, large pressure can generate a field of gravity. Positive pressure causes an attracting field of gravity; negative pressure (the ‘false’ or ‘temporary’ vacuum) causes a repelling field of gravity. The repelling gravity causes the universe to expand exponentially. The energy density of the ‘false vacuum’ has the specific property of remaining constant when it expands. During inflation, pressure (or gravity) is converted into more space, without the energy density decreasing. Stephen Hawking discovered that the spectrum of density disturbances, predicted by inflation theory, has a simple form: it is scale-invariable, which essentially means that each wavelength has the same strength. It is therefore remarkable that we have considered h/2πc as the smallest quantum physical event. Every ‘smallest space-action’ occurs in the Minkowski space. The perceptible universe is situated in the space of the general relativity theory. This ‘smallest space-action’ confirms therefore once again that a modification of general relativity is necessary. It is moreover well-known that a better mathematical description, in which singularities are avoided, is needed. A possible explanation for the fact that ‘space and time’ supposedly only consist of a loose collection of points, yet should be shaped by a connection between these points, can lie in the very existence of a ‘smallest space-action’. For, to enable a description of the first moments of the universe, a quantisation of gravity and therefore of time-space is imperative. Thus, for a unification theory the importance of inflation theory needs to be strongly endorsed, and, as Planck already suggested, the evolutive process, from the creation of the universe, indeed needs to be taken into account.
References
[1] | Lemaitre, G. The Beginning of the World from the Point of View of Quantum Theory. Nature 127 (1931), n. 3210, pp. 706. |
[2] | Einstein, A., Podolsky, B. & Rosen, N. Can quantummechanical description of physical reality be considered complete? Phys. Rev. 47, 777 780 (1935). |
[3] | Harrigan, N. & Spekkens, R. W. Einstein, incompleteness, and the epistemic view of quantum states. Foundations of Physics 40, 125 157 (2010). |
[4] | Kochen, S. & Specker, E. P. The problem of hidden variables in quantum mechanics. Journal of mathematics and mechanics 17, 59 87 (1967). |
[5] | Bell, J. S. On the Einstein-Podolsky-Rosen paradox. In Speakable and unspeakable in quantum mechanics, chap 2 (Cambridge University Press, 1987). |
[6] | Colbeck, R. & Renner, R. Is a system’s wave function in one-to-one correspondence with its elements of reality? Phys. Rev. Lett. 108, 150 402 (2012). |
[7] | Colbeck, R. & Renner, R. No extension of quantum theory can have improved predictive power. Nature Communications 2, 411 (2011). |
[8] | Pusey, M. F., Barrett, J. & Rudolph, T. The quantum state cannot be interpreted statistically. e-print arXiv: 1111. 3328 (2011). |
[9] | Zeilinger, A. Einsteins Schleier. Verlag C. H. Beck, München (2003). |
[10] | ’t Hooft, G. Kwantummechanica is slechts dwaalspoor. Natuurwetenschap & Techniek, 76 nr.1 (2008). |
[11] | Gell-Mann, M. The Quark and the Jaguar. Adventures in the Simple and the Complex. Amsterdam/Antwerpen: Contact (1994). |
[12] | Yourgrau, W. & Mandelstam, S. Variational Principles in Dynamics and Quantum Theory. New York: Dover Publications, (1968). |