Haruna Isah 1, Danladi Eli 2, Gyuk P. M. 3
1Department of Physics, Bayero University, Kano, Nigeria
2Department of Physics, Nigerian Defence Academy, Kaduna, Nigeria
3Department of Physics, Kaduna State University, Kaduna, Nigeria
Correspondence to: Danladi Eli , Department of Physics, Nigerian Defence Academy, Kaduna, Nigeria.
Email: |  |
Copyright © 2015 Scientific & Academic Publishing. All Rights Reserved.
This work is licensed under the Creative Commons Attribution International License (CC BY).
http://creativecommons.org/licenses/by/4.0/

Abstract
We developed an organic dye sensitized solar cell with TiO2 nanostructures integrated into the photoanode. The sensitizer in this research paper was extracted from flame tree flowers (delonix regia) and pawpaw (carica papaya) leaves respectively. The flame flower was separated from its stalk. Each of the samples was crushed separately via the use of a porcelain mortar and pestle. The samples, each, was filtered and stored in test tubes. The dye is chemically connected to a porous layer of a wide band-gap semiconductor. The DSSC employing the nanocrystalline TiO2 as photoanode shows a short circuit current density of 0.01849 mA/cm2, open circuit voltage of 0.525 V, fill factor of 0.520 and a power conversion efficiency of about 0.0051%.
Keywords:
Organic dye, Sensitizer, Nanocrystalline TiO2, Electron injection
Cite this paper: Haruna Isah , Danladi Eli , Gyuk P. M. , Development of Organic Dye Sensitized Solar Cell Incorporated with TiO2 Nanostructures with Low Conversion Efficiency for Exploring Solar Energy Concepts, International Journal of Optoelectronic Engineering, Vol. 5 No. 1, 2015, pp. 16-19. doi: 10.5923/j.ijoe.20150501.03.
1. Introduction
Even before the industrial revolutions human life quality is greatly affected by the availability of energy. The escalated and savage consumption of conventional sources of energy are leading to forecasted energy and environmental crises. Renewable energy sources such as solar energy are considered as a feasible alternative because “More energy from sunlight strikes Earth in 1 hour than all of the energy consumed by humans in an entire year.” [1]. Facilitating means to harvest a fraction of the solar energy reaching the Earth may solve many problems associated with both the energy and global environment [2]. Therefore, intensive research activities have resulted in attention-grabbing to the different classes of organic based solar cells.The most well known and studied unconventional photovoltaic system is the dye- sensitized nanostructured solar cell developed by Professor Grätzel [3]. At the moment this unique photoelectrochemical solar cell based on a TiO2 nanoparticle photoelectrode sensitized with a light-harvesting metallo-organic dye, is on the verge of commercialization offering an interesting alternative for the existing silicon based solar cells as well as for the thin film solar cells. At the same time the research activity as well as the industrial interest around the technology is growing fast.Major advantages of DSSCs are the large flexibility in shape, colour, transparency, and performance also under diffuse light. DSSCs could be integrated into large varieties of products, e.g. hand bags or clothing, indoor applications, and building-integrated photovoltaics such as rollable devices for walls of buildings or windows. But many components of a DSSC remain to be optimized. We focus on the identification and usage of natural pigments as light harvesting elements in DSSCs. Natural pigments as photosensitizers in DSSCs have the potential to reach similar performances and stability as known from dyes based on metal complexes. It also has the disadvantage of lower efficiency than silicon cells and it is wet, so exposure to air degrades performance.In this work, we reported the performance of a DSSC Co-sensitized with a mixture of carica papaya leaves and delonix regia with water as extracting solvent for the dye. The performance of this cell was compared with a similar cell fabricated under the same condition but with ethanol as extracting solvent [4].Flame tree flower (Delonix regia) is a specie of flowering plant in the family of Fabaceae, subfamily Caesalpinioideae. It is noted for its fern-like leaves and flamboyant display of flowers. In many tropical parts of the world it is grown as an ornamental tree and in English it is given the name Royal Poinciana or Flamboyant as shown in Figure 1a;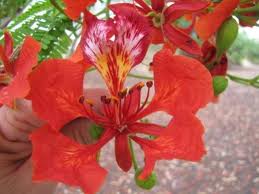 | Figure 1a. Flame tree flower |
Pawpaw is a common name for a small tropical and subtropical South American tree, and for its fruit. The papaw belongs to the family Caricaceae. It is classified as Carica papaya and is shown in Figure 1b;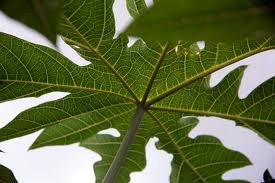 | Figure 1b. Pawpaw leaf (bottom) |
2. General Composition, Function and Parameters of the DSSCs
The cell is composed of four elements, namely, the transparent conducting and counter conducting electrodes, the nanostructured wide bandgap semiconducting layer, the dye molecules (sensitizer), and the electrolyte. The transparent conducting electrode and counter-electrode are coated with a thin conductive and transparent film (such as fluorine- doped tin dioxide (SnO2)). The TiO2 surface is stained with a dye. TiO2 nanocrystals are used rather than a continuous layer to maximize surface area for light absorption. Between the electrodes is an electrolyte. Upon absorption of photons, dye molecules are excited from the highest occupied molecular orbitals (HOMO) to the lowest unoccupied molecular orbital (LUMO) states as shown schematically in Figure 1c. This process is represented by Eq. 2. Once an electron is injected into the conduction band of the wide bandgap semiconductor nanostructured TiO2 film, the dye molecule (photosensitizer) becomes oxidized, (Equation 3). The injected electron is transported between the TiO2 nanoparticles and then extracted to a load where the work done is delivered as an electrical energy, (Equation 4). The electrons flow through the TiO2 onto the electrode, through an electric circuit, and then to the counter electrode. The electrolyte carries electrons back to the dye from the counter electrode. Electrolytes containing
redox ions is used as an electron mediator between the TiO2 photoelectrode and the coated counter electrode. Therefore, the oxidized dye molecules (photosensitizer) are regenerated by receiving electrons from the
ion redox mediator that get oxidized to
(Tri-iodide ions). This process is represented by Eq. 5. For the HOMO level to effectively accept the donated electrons from the redox mediator, the energy difference between the HOMO and redox chemical potential must be more positive [5].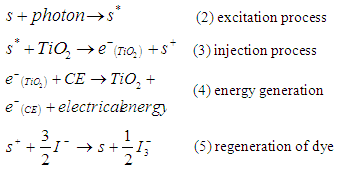
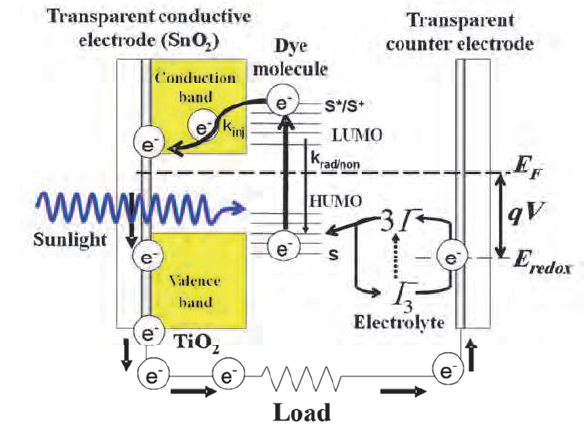 | Figure 1c. Schematic illustration of operation principle of the dye sensitized solar cell |
3. Experimental Procedure
3.1. Extraction of Dye
The flame tree flower (delonix regia) and pawpaw (carica papaya) leaves were collected in plastic rubber from flamboyant tree and pawpaw tree respectively. The flame flower was separated from its stalk. Each of the samples was crushed separately via the use of a porcelain mortar and pestle. The samples, each, were filtered and stored in test tubes. The filtrate (extract) is the dye solution for sensitization.
3.2. Dye Mixture
Pawpaw leaf (Carica Papaya) and flame tree flower (Delonix Regia) dye extracts were mixed in a ratio of 1:4 that is, (20ml of pawpaw leaf (Carica Papaya) and 80ml of flame tree flower (Delonix Regia) dye molecules).
3.3. DSSC Assembly
The fluorine doped tin oxide (FTO) electrodes were washed with laury sulphate then later rinsed with water six times in an ultrasonication bath for ten (10) minutes then finally washed in propanol. The photoanode was prepared by first depositing a blocking layer on the FTO glass, followed by the nanocrystalline TiO2 (bought from solaronix). The blocking layer was deposited from a 2.5 wt% TiO2 precursor and was applied to the FTO glass substrate by spin coating and subsequently sintered at 500°C for 45 mins. The 9 μm thick nanocrystalline TiO2 layer was deposited by screen printing. It was then sintered in air for 30 minutess at 500°C. The counter electrode was prepared by screen printing a platinum catalyst gel coating onto the FTO glass. It was then dried at 100°C and fired at 400°C for 30 mins.The sintered photo anode was sensitized by immersion in the sensitizer solution at room temperature overnight. The cell was assembled by pressing the photoanode against the platinum-coated counter electrode slightly offset to each other to enable electrical connection to the conductive side of the electrodes. Between the electrodes, a 50 μm space was retained using two layers of a thermostat hot melt sealing foil. Sealing was done by keeping the structure in a hot-pressed at 100°C for 1 minute. The liquid electrolyte constituted by 50 mmols of iodide/tri-iodide in acetonitrile was introduced by capillary action into the cell gap through a channel previously fabricated at opposite sides of the hot melt adhesive, the channel was then sealed.
4. Characterization and Measurement
The current-voltage (I-V) data was obtained using a keithley 2400 source meter under AM1.5 (100 mw/cm2) illumination and without illumination from a Newport A solar simulator, Scanning electron micrographs of the nanocrystalline TiO2 films are taken with Carl Zeis SEM, The absorption spectrum of the dye was recorded on Ava-spec-2048 spectrophotometer, The cell active area was 0.021cm2. Thickness measurement was obtained with a Dektac 150 surface profiler. X-ray microanalysis was carried out with INCA EDX analyzer.
5. Results and Discussion
The SEM micrograph shows that the TiO2 nanoparticles produced have a mean particle size of about 25nm. It also reveals that the surface is porous and has agglomeration.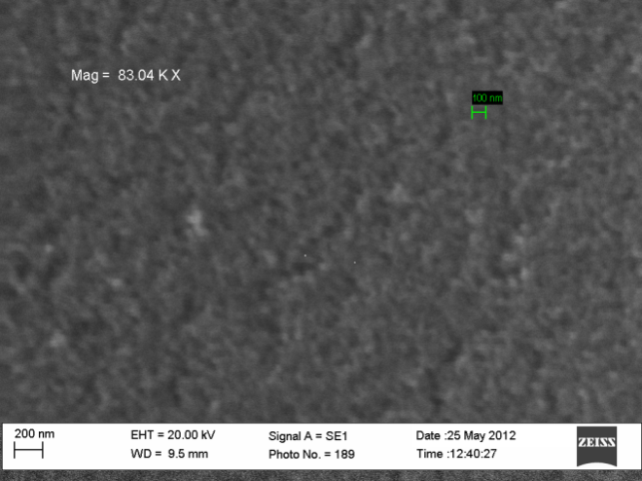 | Figure 2a. The Scanning electron microscope surface morphology of TiO2 sample |
The elements present in the TiO2 are Titania, Chlorine, Oxygen and Nitrogen. Nitrogen is present due to the blower that was used to dry the TiO2 semiconductor.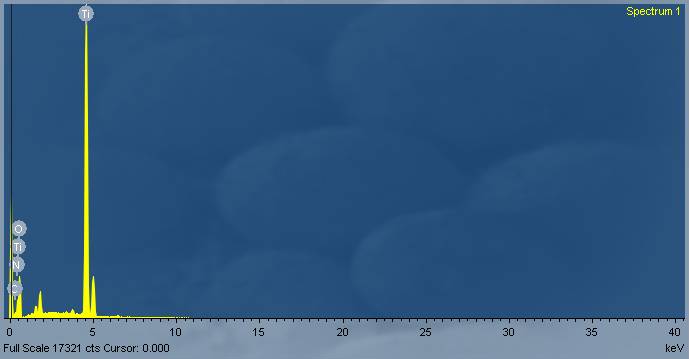 | Figure 2b. EDX Image Showing the Elements Present in the TiO2 Compound |
From Figure 2c, it was deduced that the dye absorbs photons best at a wave length peak of 370nm within the range of 350nm to 550nm.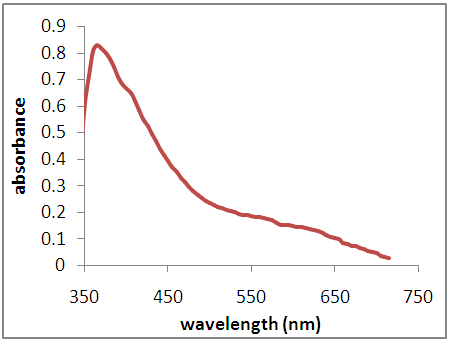 | Figure 2c. Absorption Spectra of Dye (Flame Tree Flowers and Pawpaw Leaves Dye Mixture) |
Figure 3. shows the photocurrent density–voltage (J-V) curve of the DSSC under illumination. Under illumination the J-V curve is shifted by the value of the current generated by the cell. Based on the J-V curve, the fill factor (FF) and solar cell efficiency
were determined using equations (1) and (2) respectively.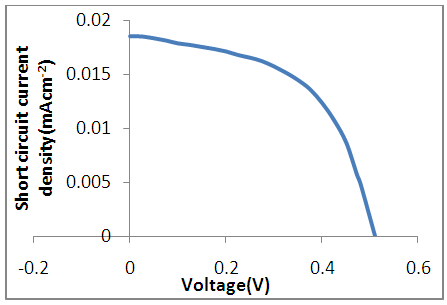 | Figure 3. Photocurrent density-voltage (J-V ) curve under illumination |
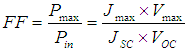 | (1) |
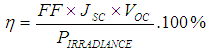 | (2) |
Where Vmax = maximum voltage (V); Jmax = maximum current (mA/cm2); Jsc = short current (mA/cm2); Voc = open circuit voltage (V) and PIRRADIANCE = light intensity (mW/cm2)Calculations of Fill Factor and Conversion EfficiencyThe dimensions of the active area of the cell was measured to be (0.03cm X 0.7cm) giving an active area of 0.021 cm2. From Fig. 3, the value of Jsc, Voc, Jmax and Vmax for the cell are 0.01849 mA/cm2, 0.5249 V, 0.0144426 mA/cm2, 0.35VFrom equ (1)
From equ (2)Efficiency (η) of the cell is therefore,
In consideration to the calculations carried out, the cell efficiency is relatively poor because the dye was extracted using water as solvent. This result could be compared with the result obtained by Kimpa et.,al [4] where 0.27% was obtained for the efficiency of mixture of flame tree flower and pawpaw leaf (with ethanol as the extracting solvent of the dye extraction).
6. Conclusions
Dye sensitized solar cell using the dye extract from flame tree flower (Delonix regia) and pawpaw leaf (Carica papaya) impregnated nanostructure TiO2 was successfully fabricated. Poor charge transfer between the dye molecule and the TiO2 due to low regeneration kinetics could be responsible for the low conversion efficiency of the cell. The dye from flame tree flower and pawpaw leaf can therefore, be made more promising efficient by the use of suitable extracting solvent [6]. The overall cell efficiency of dye sensitized solar cell is found to be proportional to the electron injection efficiency in the wide bandgap nanostructured semiconductor.
ACKNOWLEDGEMENTS
The authors are grateful to the physics advanced laboratory, Sheda Science and Technology Complex (SHESTCO), Abuja, Nigeria for the use of their research facilities.
References
[1] | Lewis, N. S. (2007). Toward Cost-Effective Solar Energy Use. Science, Vol 315, pp. 798-801. |
[2] | Nansen, R. (1995). Sun Power: The Global Soluteion for the Coming Energy Crisis s. Ocean Press, ISBN-10: 0964702118, Washington, USA. |
[3] | Gratzel, M., (2003). Dye-sensitized solar cells. Journal of Photochemistry and Photobiology C: Photochemistry Reviews 4, pp. 145–153. |
[4] | Kimpa. M.I., Momoh M., Isah K.U., Yahya H.N., and Ndamitso M.M (2012). Photoelectric Characterization of Dye-Sensitized Solar Cells using Natural Dye from Pawpaw Leaf and Flame Tree Flower as Sensitizers. Material sciences and applications, 3. 281-286. |
[5] | Hara, K. & Arakawa, H. (2003). Dye-sensitized Solar Cells, In: Handbook of Photovoltaic Science and Engineering, A. Luque and S. Hegedus, (Ed.), Chapter 15, pp. 663-700, John Wiley & Sons, Ltd, ISBN: 0-471-49196-9. |
[6] | Wongcharee, K., Meeyoo V. and Chavadej, S., (2006). Dye-sensitized solar cell using natural dyes extracted from rosella and blue pea flowers. Journal Solar Energy & Solar Cell, 91, 566 – 571. |