V. P. Geetha Vani1, R. W. Miles2, K. T. Ramakrishna Reddy1
1Department of Physics, Sri Venkateswara University, Tirupati, 517502, India
2School of CEIS, Northumbria University, Newcastle, NE1 8ST, U. K
Correspondence to: K. T. Ramakrishna Reddy, Department of Physics, Sri Venkateswara University, Tirupati, 517502, India.
Email: |  |
Copyright © 2012 Scientific & Academic Publishing. All Rights Reserved.
Abstract
The ternary semiconductor, copper tin sulphide (Cu4SnS4) is considered to be a promising absorber layer in the development of thin film heterojunction solar cells due to its optimum physical properties. Further, it contained more abundant and cheaper elements that are environmentally safe to handle. In the present study, Cu4SnS4 (CTS) films were deposited by co-evaporation technique at various deposition temperatures in the range 200 – 350℃. The effect of substrate temperature (Ts) on the growth of CTS films was investigated. The structure, composition, morphology and optical properties of the films were studied using appropriate techniques. The X-ray diffraction analyses revealed that all the deposited films were polycrystalline and showed the (221) plane as the preferred orientation. The energy dispersive spectroscopy analysis indicated all the three elements, Cu, Sn and S. The grain size of the films was varied in the range 180 – 350 nm. The average optical transmittance of the films was found to be ~ 80% in the visible region and the evaluated optical band gap of the films increased from 1.70 eV to 1.93 eV with the increase of substrate temperature.
Keywords:
Cu4SnS4 Films, Co-Evaporation, Structure, Optical Properties
Cite this paper: V. P. Geetha Vani, R. W. Miles, K. T. Ramakrishna Reddy, Preparation and Properties of Cu4SnS4 Thin Films, International Journal of Optoelectronic Engineering, Vol. 3 No. 1, 2013, pp. 1-5. doi: 10.5923/j.ijoe.20130301.01.
1. Introduction
Polycrystalline thin film solar cells using CuInGaSe2 (CIGS) and CdTe as absorber materials have proved to be potential candidates for photovoltaic solar cell fabrication with the demonstrated efficiencies>15%[1, 2]. Although solar cell modules based on these materials are commercially available in the market, however, these cells are facing few serious problems that might cloud their prospects in the future. The availability of In and Ga in the case of CIGS and the toxicity of Cd in CdTe-based cells are considered to be the detractors of these technologies for large scale production of the modules with more public acceptability. Therefore, attention of researchers is diverted towards the investigation of novel and new photovoltaic materials that are freely available in nature at low cost and likely to replace these front runner materials in the future. Among the many materials that have been studied in recent years, the ternary semiconductor, copper zinc tin sulphide (CZTS) is proved to be an important alternative with the conversion efficiencies > 10%[3, 4]. But it is a quaternary material where the elements involved had a large variation in the vapor pressures. A ternary or binary compound with similar properties to CZTS will be more useful. In this context, copper tin sulphide (CTS) is considered to be one of the potential materials as an absorber layer in solar cells because of its optimum physical properties such as energy band gap and optical absorption coefficient[5, 6]. Further, it contained more abundant and cheaper elements that are environmentally safe to handle. Moreover, the investigations reported on the deposition and characterization of copper tin sulphide layers is very meager. Copper-tin-sulphide system exists in different stable forms and the prominent of them are: Cu2SnS3 with the disordered cubic structure and Cu4SnS4 that exists in orthorhombic structure[7]. The present investigation is aimed to deposit Cu4SnS4 (CTS) films by a simple co-evaporation technique using CuS and SnS as precursors. The composition, structure and optical properties of the grown films were studied in relation to the substrate temperature and the results discussed.
2. Experimental Details
Thin films of copper tin sulphide were formed on glass substrates by vacuum co-evaporation technique. A Hind Hi Vac Box Coating system was used for the deposition of the films at a vacuum better than 10-5 Torr. 5N pure SnS powder and CuS were used as the starting materials to grow the films. The materials were taken in two tantalum boats connected to two separate heating sources. The boats were covered with quartz wool in order to avoid splattering of the materials. The temperatures of both SnS and CuS sources were maintained at the predetermined values for the evaporation to occur. After getting the required evaporation rate, the two shutters over the sources were moved sideways for the deposition to take place. Ultrasonically cleaned Corning glass was used as the substrates. The layers were deposited at various substrate temperatures (Ts) that vary in the range, 200–350℃. The source-substrate distance was maintained as 20 cm. The as-grown layers were characterized to evaluate the chemical and physical properties using appropriate techniques and procedures. Philips X-ray diffractometer with Cu kα radiation (λ=1.542 A) was used to analyze the structural properties of the films such as the crystal structure, preferred orientation, crystallite size and texture coefficient. The X-ray diffraction (XRD) spectra were recorded in the 2θ range, 20-70. The chemical composition of the films was measured using Energy dispersive X-ray analyzer (Inca Penta FETx3) attached to scanning electron microscope (SEM). The surface topology and grain size of the films were investigated using Zeiss SEM. The optical properties such as the transmittance, reflectance, absorption coefficient and energy band gap were determined using Perkin Elmer UV-Vis-NIR spectrophotometer. In this study, the thickness of the films was measured using the quartz crystal thickness monitor present in the vacuum system and it was approximately 200 nm.
3. Results and Discussion
The visual observation of the as-grown CTS layers indicated that the films were reddish brown in colour, pin hole free and uniform. The scratch tape test revealed that the film were strongly adherent to the surface of the substrate. The energy dispersive X-ray analysis data revealed that all the layers grown at different substrate temperatures consisted of copper, tin and sulphur with small amount of carbon. The presence of C might be due to the oil vapours from the diffusion pump. Figure 1 shows a typical EDAX spectrum of CTS fil deposited at 350℃. The films formed at higher substrate temperatures >300℃ showed slight deficiency of S compared to other elements. This might be due to its re-evaporation because of the high vapour pressure. The EDAX analysis also indicated that the composition of various elements was nearly stoichiometric for the films formed at 300℃. CTS films deposited at other substrate temperatures have shown compositional deviation from the stoichiometric value. 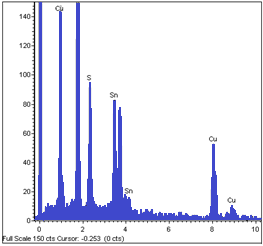 | Figure 1. EDAX spectrum of CTS film formed at 350℃ |
Figure 2 shows the X-ray diffraction (XRD) spectra of CTS films deposited at different substrate temperatures. The spectra revealed that all the films were polycrystalline and showed the (221) plane appearing at a 2θ value of 30.31o as the preferred orientation. The other orientations observed in the XRD spectra were (102), (420), (222), (502), (512) (040) (711) and (004) that correspond to the crystal planes of Cu4SnS4 phase. The different orientation observed in this work are in agreement with the data reported on electrodeposited films[8]. However, Nair et al. reported different orientations for Cu4SnS4 layers prepared by heating a thin layer of CuS on SnS deposited by chemical bath deposition[9]. It can be also seen from the figure that with increase of substrate temperature, the (221) peak intensity increased with a decrease of the fringe width at half maximum (FWHM). This indicates that the crystallinity of the films was improved with the increase of substrate temperature. The crystallite size, D of the films was evaluated using the Debye Scherer formula[10] | (1) |
where ‘β’ is the FWHM of (221) peak of CTS, ‘λ’ is the wavelength of X-rays and ‘θ’ is the corresponding diffraction angle. The evaluated crystallite size varied in the range, 18–35 nm. 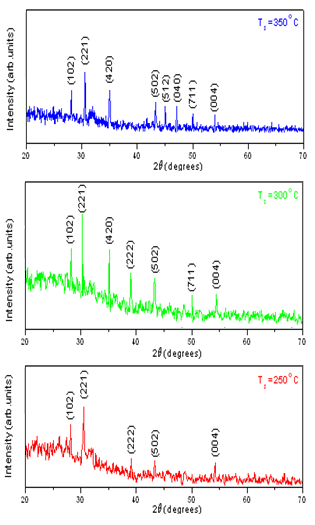 | Figure 2. X-ray diffraction spectra of CTS films grown at various temperatures |
For thin films, a wide variety of textures are possible depending on the deposition conditions used to synthesize the layers. In this study, the texture coefficient (TC) was determined using the preferred orientation from the X-ray diffraction spectra of CTS films deposited at various substrate temperatures using the Harris texture analysis[11]. The texture coefficient represents the preferential growth of certain planes compared to randomly oriented crystallites and is defined by the relation,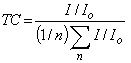 | (2) |
Here I is the measured intensity of the intense peak in the XRD spectrum, Io is the intensity for completely random sample (JCPDS) and ‘n’ is the number of reflections considered in the analysis. The preferential orientation of each sample as a whole was analysed by the standard deviation (σ) of all the texture coefficient values as compared to randomly oriented crystallites.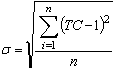 | (3) |
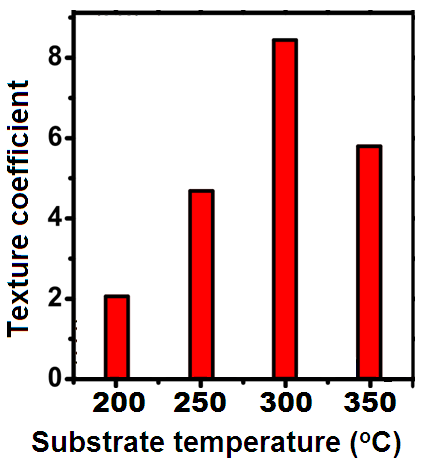 | Figure 3. Variation of texture coefficient with substrate temperature |
The σ value can be used to compare the degree of orientation of the crystallites in the samples. If TC=1, it represent the films with randomly oriented crystallites whereas the TC is higher than one for the films that are oriented preferentially in a particular direction. In order to know the final texture of the films and to determine the preferred orientation of the grains, strain energy and surface energy minimization have a significant influence on them. In the present investigation, the (221) plane is the predominant peak. Figure 4 shows the variation of texture coefficient with substrate temperature. It indicates an increase of texture coefficient with substrate temperature. Figure 4 shows the typical SEM pictures of CTS layers grown at different substrate temperatures. The pictures showed that the layers formed at 200℃ had smooth surface topography while the films formed at higher substrate temperatures revealed flake-like structure. The size of these flakes increased with the increase of substrate temperature. However, the films grown at 350℃ indicated clusters of these grains formed into bigger grains of circular shape. The evaluated grain size from the SEM analysis is higher than that value calculated using the XRD data.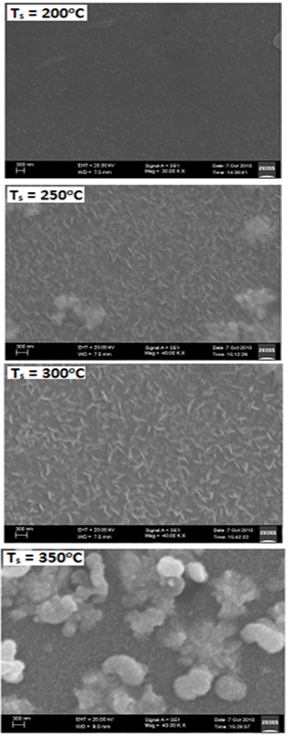 | Figure 4. SEM photographs of CTS films formed at different substrate temperatures |
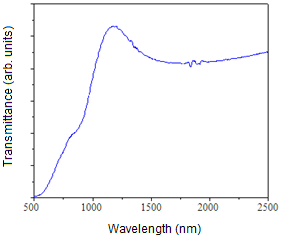 | Figure 5. Optical transmittance versus wavelength spectrum of CTS films grown at Ts=300℃ |
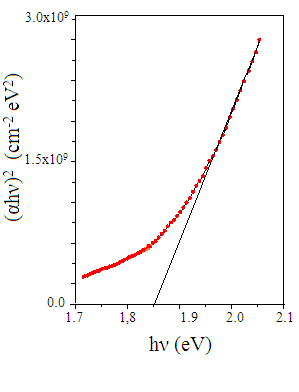 | Figure 6. (αhν)2 versus hν plot for CTS film formed at Ts=300℃ |
The optical absorption measurements are extremely important in evaluating the optical parameters of thin films such as optical transmittance, reflectance, absorption coefficient, energy band gap etc. The dependence of absorption coefficient, ‘α’ on the photon energy, ‘hν’ gives the information regarding the type of optical transition occurred between the valence band and conduction band of the sample. The optical transmittance spectra for all the films were recorded in the wavelength range, 500–2500 nm at room temperature. The films formed at lower substrate temperatures had lower optical transmittance and it increases with the increase of substrate temperature. Figure 5 shows the variation of transmittance with wavelength for a typical CTS films grown at Ts=300℃. The optical absorption coefficient in the absorption region was determined using the relation[12],  | (4) |
Here, ‘T’ is the transmittance and ‘t’ is the thickness of the film. The evaluated optical absorption coefficient, α was >104 cm-1, indicating that the films were highly absorbing. The optical energy band gap ‘Eg’ is determined using the following relation, which is valid for direct band gap semiconductors[12]. | (5) |
where α is the absorption coefficient, hυ is the photon energy. The extrapolation of the linear portion of the plot on to the energy axis gives the energy band gap of the film, which varied in the range, 1.70–1.94 eV in the investigated substrate temperature range. The linear dependence of (αhν)2 with hν plot for a typical CTS film grown at a Ts=300 ℃ in Figure 6 that showed a band gap of 1.85 eV. The optical band gap obtained in the present work is slightly higher than the value reported in literature[13].
4. Conclusions
Thin films of Cu4SnS4 have been deposited by co-evaporation method. The films were formed on Corning glass substrates at different temperatures varying from 200℃ to 350℃. The layers formed at 300℃ showed nearly stoichiometric composition. All the grown films were polycrystalline with the (211) plane as the dominant orientation and the cyrstallinity of the films increased with the increase of substrate temperature. The films formed at substrate temperatures > 250℃ had an optical transmittance > 60%. The films exhibited an absorption coefficient > 104 cm-1 and the evaluated energy band gap varied in the range, 1.70 – 1.94 eV.
ACKNOWLEDGEMENTS
One of the authors (V.P. Geetha Vani) would like to thank UGC for awarding RFSMS Fellowship. Prof. K. T. R. Reddy, Prof. R. W. Miles, Sri Venkateswara University and Northumbria University acknowledge financial support from European commission via Marie-Curie funding.
References
[1] | Philip Jackson, Dimitrios Hariskos, Erwin Lotter, Stefan Paetel, Roland Wuerz, Richard Menner, Wiltraud Wischmann and Michael Powalla, 2011, New world record efficiency for Cu(In,Ga)Se2 thin-film solar cells beyond 20%, Progress in Photovoltaics: Research and Applications, 19 (7), 894–897. |
[2] | Miles R.W., Zoppi G., Ramakrishna Reddy K.T. and Forbes I., Organic Nanostructured Coatings and Coatings for Clean Energy,Volume 3, CRC Press, New York (2010) p-1-56. |
[3] | Barkhouse D.A.R., Oki Gunawan, Tayfun Gokmen, Teodor K. Todorov, David B. Mitzi, 2012, Device characteristics of a 10.1% hydrazine-processed Cu2ZnSn(Se,S)4 solar cell, Progress in Photovoltaics: Research and Applications, 20 (1), 6-11. |
[4] | Shafaat Ahmed, Kathleen B. Reuter, Oki Gunawan, Lian Guo, Lubomyr T. Romankiw, Hariklia Deligiann, 2012, A High Efficiency Electrodeposited Cu2ZnSnS4 Solar Cell, Advanced Energy Materials, 2 (2), 253-259. |
[5] | Avellaneda D., Nair M. T. S. and Nair P. K., 2010, Cu2SnS3 and Cu4SnS4 Thin Films via Chemical Deposition for Photovoltaic Application, Journal of Electrochemical Society, 157 (6), D346-D352. |
[6] | Qinmiao Chen, ZXiaoming Dou, Yi Ni, Shuyi Cheng and Songlin Zhuang, 2012, Study and enhance the photovoltaic properties of narrow bandgap Cu2SnS3 solar cell by p-n junction interface modification, Journal of Colloid and Interface Science, 376 (1), 327-330 . |
[7] | Daqing Wu, Charles R. Knowles, Luke L. Y. Chang, 1986, Copper-tin sulphides in the systemCu-Sn-S, Mineralogical Magazine, 50 (356), 323-325. |
[8] | Anuar K.., Ho S.M., Tan W.T., Atan S., Kuang Z., Haron M.J. and Saravanan N., 2008, Effects of Bath Temperature on the Electrodeposition of Cu4SnS4 Thin Films, Journal of Applied Science Research, 4 (12), 1701-1707. |
[9] | Nair M.T.S., Lopéz-Mata C., GomezDaza O. and Nair P.K., 2003, Copper tin sulfide semiconductor thin films produced by heating SnS–CuS layers deposited from chemical bath, Semiconductor Science and Technology, 18 (8), 755-759. |
[10] | Barren B.E., X-ray Diffraction, Dover, New York, (1990) p.253. |
[11] | Moutinho H.R., Hasoon E.S., Abulfotuh F. and Kazmerski L.L., 1995, Journal of Vacuum Science and Technology A, 13 (6), 2877-2883. |
[12] | Pankov J.I., Optical Process in Semiconductors. 2nd ed. Academic Press, New York (1975). |
[13] | Anuar Kassim, Saravanan Nagalingam, Zulkefly Kuang, Atan Sharif, Tan Wee Tee, Ho Soon Min, 2010, Influence of complexing agent (Na2EDTA) on chemical bath deposited Cu4SnS4 thin films, Bulletin of Chemical Society of Ethiopia, 24 (2), 259-266. |