N. M. El-Gohary1, Mohsen A. M. Kassem2, F. E. Abed El-Samie3, M. M. Fouada1
1Department of Electonics and Communication, Faculty of Engineering, Zagazig University, Egypt
2Department of Communication Technology, Faculty of Industrial Education, Helwan University, Egypt
3Department of Electonics and Communication, Faculty of Electonics Engineering, Menofia University, Egypt
Correspondence to: Mohsen A. M. Kassem, Department of Communication Technology, Faculty of Industrial Education, Helwan University, Egypt.
Email: |  |
Copyright © 2016 Scientific & Academic Publishing. All Rights Reserved.
This work is licensed under the Creative Commons Attribution International License (CC BY).
http://creativecommons.org/licenses/by/4.0/

Abstract
In the course of recent years, there has been expanding confirmation on broadening the services available on wired public telecommunications networks to mobile/wireless telecommunications users. At present, only low-bit-rate data services in addition to voice services are available to mobile users. However, the demand for Wireless Broadband Multimedia Communication Systems (WBMCS) is expected to increase within both the public and special sectors. The wired networks are cannot support extensions to wireless mobile networks, where it misses the configuration flexibility and the infrastructure needs. In fact, the mobile radio channels are more polluted than wired data-transmission channels, hence the high Quality of services (QoS) tools are required in wired networks cannot be obtained in wireless networks. The object of the third and the fourth generation of mobile networks are to extend users with high data rates, and to spread a wider range of services, such as voice communications, videophones, and high-speed Internet access. A common challenge in designing a wireless system is to control and decrease the effects of the wireless channel, having multiple transmission paths and being time varying. Orthogonal Frequency Division Multiplexing (OFDM) is a wideband modulation scheme that is designed to overcome the problems of multi-path channels. In this paper, we examine the wireless OFDM system utilizing Raptor codes. Raptor code is the new developing rate less code which has indicated wonderful performance over a mixture of channels. There is an insistence on the interleaving depth of OFDM-based system because of delay and maximum packet size. This non-perfect interleaving influences the most extreme achievable assorted qualities from the channel. We explored the impact of connection between's cloudiness pieces, which identifies with the constrained interleaving imaginable between carrier in an OFDM system using Raptor code. The computer simulation experiments are carried out to evaluate the proposed technique and measure the degree of the Raptor code acceptance. There are also, many metrics are utilized based on the diverse modulation techniques and based on Raptor codes, also, for BER computation.
Keywords:
Raptor codes, Orthogonal Frequency Division Multiplexing (OFDM), Quality of services (QoS), Bit error rate (BER)
Cite this paper: N. M. El-Gohary, Mohsen A. M. Kassem, F. E. Abed El-Samie, M. M. Fouada, Performance Enhancement of Raptor Codes Employment in OFDM-System, International Journal of Networks and Communications, Vol. 6 No. 6, 2016, pp. 103-110. doi: 10.5923/j.ijnc.20160606.01.
1. Introduction
In advanced wireless communication system, the Forward Error Correcting (FEC) codes are utilized for productive transmission of information in hard situations, it plays an essential role in controlling the bad effect of the wireless channels. Accomplishing a less bit error rate (BER) has been the significant undertaking in the field of error control coding; Source codes [1-3] are an idea of forward error correcting codes. The main acknowledgment of Fountain codes was Luby Transform (LT) codes [4]. Raptor codes, which are a class of Fountain codes and the expansion of LT codes with direct time encoding and decoding, tend to give preferred execution over the low density parity check (LDPC) codes on burst error channels [2, 7]. Raptor code [8] has the properties of direct time, straight time decoding, and near perfect code execution under any channel misfortune conditions. The execution of the wireless OFDM communication system is examination utilizing Raptor codes. The time-shifting elements of wireless correspondence channels negatively influence the system execution. Raptor code is the new rising rate less code which has indicated astounding execution over a variety of channels. There is an imperative on the interleaving profundity of OFDM-based system because of deferral and most extreme packet size. This non-perfect interleaving influences the most extreme achievable differing qualities from the channel. Whatever remains of the paper is composed as takes after: In Section II, essential of Orthogonal Frequency Division Multiplexing (OFDM) system outline necessities is presented. In section III, Fountain Codes, Luby Transform code and Raptor Codes are clarified. Section IV, the proposed system model of the OFDM system is presented. In Section V, simulation results will be discussed with the assistance of the graphical representation characteristic plan over AWGN and Rayleigh Fading Channels. Section VI Discuss the throughput and analysis with Raptor consideration. Section VII, conclusions will be advanced.
2. OFDM
OFDM is of great concern by researchers and research laboratories all over the world. It has already been accepted for the new wireless local area network standards IEEE 802.11a, High Performance LAN type 2 (HIPERLAN/2) and Mobile Multimedia Access communication (MMAC) Systems. Also, it is expected to be used for wireless broadband Multimedia communications. OFDM Basically splits a high-rate data stream into a set of low-rate sub-streams that are transmitted simultaneously over a number of sub-carriers. Thereby, the bandwidth of the sub-carriers become small compared with the coherence channel bandwidth as in Fig 1.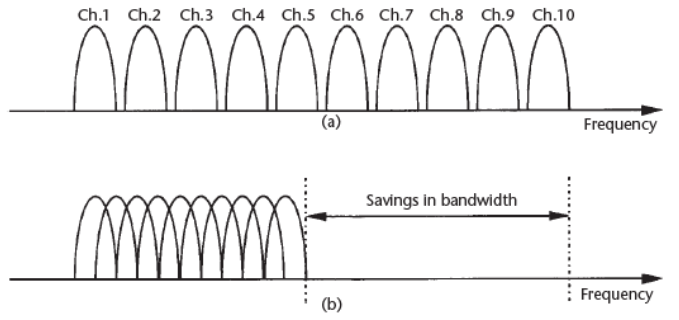 | Figure 1. Concept of the OFDM signal: (a) conventional multicarrier technique, and (b) Orthogonal multicarrier modulation technique |
Each sub-channel could be considered as a flat channel if the number of sub-channels are high enough. Increasing the number of parallel transmission channels reduces the data rate that each individual sub-carrier must convey, and that lengthens the symbol period. Because the symbol duration is increased for the low-rate parallel sub-carriers, the relative amount of dispersion in time caused by the multi-path delay spread decreases [2]. Fig 2 (a) shows the spectrum of the individual data of the subchannel. The OFDM signal, multiplexed in the individual spectra with a frequency spacing in (b) is equal to the transmission speed of each subcarrier, is shown in Fig 2 (b). The figure shows that at the center frequency of each subcarrier, there is no crosstalk from other channels.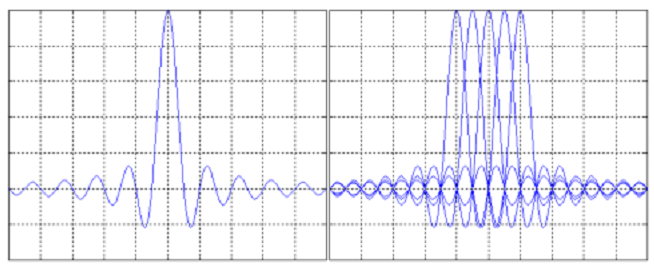 | Figure 2. Spectra of (a) an OFDM subchannel and (b) and OFDM signal |
Fig 3 shows the block diagram of a typical OFDM system. First, the input data symbols are mapped to the data symbols. Then, the symbols are serial to- parallel (S/P) converted and processed by the IFFT. The cyclic prefix is added and finally, the signal samples pass through the digital-to-analog (D/A) converter to obtain the continuous-time OFDM signal.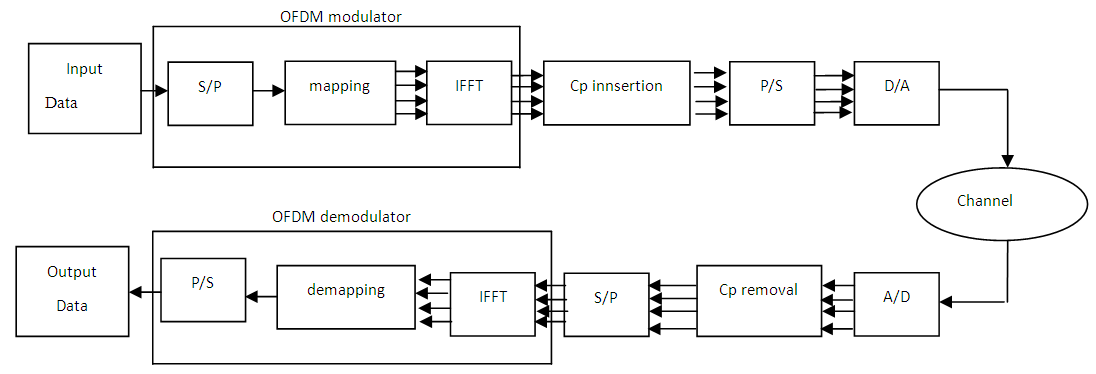 | Figure 3. OFDM Transmitter and Receiver |
3. Fountain Codes
Fountain Codes are a new class of codes designed and ideally suited for reliable transmission of data over an erasure channel with unknown erasure probability [4, 11]. A fountain code has properties similar to a water Fountain which can be thought as an infinite supply of water drops. Anyone who wants to collect the water drops holds a bucket under the fountain. When enough water is collected, the bucket is removed. Similarly with a digital source, a client gets encoded packets from one or more servers and packages once enough are obtained, the client can reconstruct the original file, which packets are obtained should not matter. They are rateless in the sense that for a given message, the encoder can produce a potentially infinite number of output symbols. Output symbols can be bits or more general bit sequences [13].A. Luby Transform CodingWe present Luby Transform (LT) codes, the first rateless cancellation codes that are extremely effective as the information length develops. LT codes, the first class of practical fountain codes, were initially designed for the Binary Erasure Channel (BEC). Although LT codes are famous for simplicity, good performance and optimality, they do not support fast encoding or decoding for large dimension. LT codes have been proposed by Michael Luby [5] to decrease the encoding and decoding complexity of random linear fountain code while keeping up the little overhead with a good choice of the degree distribution, i.e. the distributions of the edges in the Tanner graph, LT codes can come discretionarily near channel capacity with certain decoder reliability and logarithmically expanding encoding and decoding costs. To reduce the complexity with a specific end goal to lessen the multifaceted nature significantly more, the reliability of the decoder is reduced. In this way, we would have a diminished degree distribution coming about direct time encoding and decoding complexity. However, the decoder can't decode all the input data with the lower degree distribution for the same overhead imperative. In this way, using an erasure correcting pre-code would then correct the erasures emerging from the weakened decoder. Similar to an LDPC code, Raptor Codes give wonderful encoding and decoding speeds while giving close ideal execution to the BEC if the pre-code is a linear time block code [6, 12].B. Raptor Codes Raptor codes have been very streamlined and are being utilized as a part of business frameworks of Digital Fountain [6]. Raptor codes are an expansion of the other piece of LT codes joined with an arrangement of pre-coding. The configuration and degree appropriation pre-coding is the heart of Raptor codes. Rather, the media information is ensured utilizing the application layer FEC with Raptor codes.To efficiently build the transmission's dependability, an application layer FEC code can be utilized [9, 10]. A graphical presentation of a Raptor code is given in Fig 4.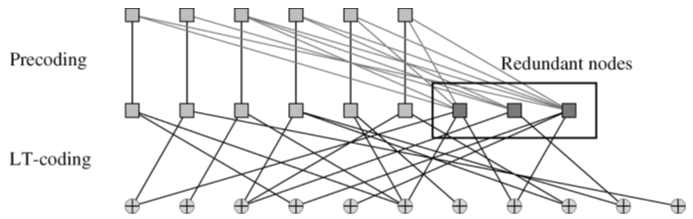 | Figure 4. Raptor codes: the input symbols are appended by redundant symbols (black squares) in the case of a systematic pre-code. An appropriate LT-code is used to generate output symbols from the pre-coded input symbols |
A Raptor code has an undeniable encoding calculation as takes after a given K input symbol; an encoding algorithm for C is used to generate a codeword C in corresponding to the input symbols. Then an encoding algorithm for the LT-code with distribution ῼ(x) is used to generate the output symbols. Raptor code is flexible error correction technique for erasure channel, it can be presented efficient trade-off between the protection degree and the bandwidth expansion. These features of the Raptor code cannot be found in the other classical error correction techniques such as Reed-Solomon codes. A DF Raptor code with k input symbols that produces n output symbols using symbols of size S bytes is designated Raptor (n,k,S). A DF Raptor code is a fountain code, capable of producing an unlimited sequence of encoded symbols (n →∞) from a block of k fixed-length source symbols. Such codes are “rateless,” allowing the actual number of encoded symbols and thus the code rate to be determined as needed to conflict the current level of network packet loss. The source symbols used for a DF Raptor code can be completely data packets or some fraction of a packet. In general, a DF Raptor code can be constructed with any combination of the number of source symbols k and symbol size S such that the total amount of data to be protected as a block is equal k ×S, subject only to the bonds that (S must ≤ the maximum packet size) so that the loss of a packet always affects a whole number of symbols. For either encoding or decoding, the number of operations of a Raptor code grows linearly with the size of the source block (i.e., processing complexity grows with the data), allowing the choice of code parameters (number of output symbols n, number of source symbols k, and symbol size S) to be relatively unaffected by processing requirements. From a source block of k source symbols, a DF Raptor code, as a fountain code, can produce any number of output symbols. Each output symbol is determined by the bit-wise XOR of a number of source symbols according to the DF Raptor algorithm, and each output symbol is thus the same size as a source symbol. The generated output symbols are then ordered in packets as necessary for transmission over the network. At the receiver, a DF Raptor decoder is able to recover the source block from any set of k′ received output symbols, where k′ is slightly greater than k, the number of source symbols in the source block. With a DF Raptor erasure code, k′> k received symbols must be used to recover the k source symbols, implying that: The maximum loss that the transmission of N output symbols can endure | (1) |
 | (2) |
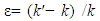 | (3) |
Where: ε represents the reception overhead associated with a DF Raptor code; ε> 0. So the reception overhead depends on the value of k and the desired probability that the source block can be fully recovered from the received output symbols. For good Fountain codes the value of n is very close to k, and the decoding time is close to linear in k, the reception overhead ε is typical < 1%.
4. Proposed System Model
Fountain codes are designed for communication over Erasure Channels, which means that the encoded packet is either received error free or not received at all. However, the wireless channels are not erased, but fading and noisy channels. In practical systems, fountain codes are used in combination with other error correction algorithms to convert the noisy channels into erasure channels, often Low-Density Parity-Check (LDPC) codes. LDPC codes together with Cyclic Redundancy Check (CRC) are employed to convert the channel, a (CRC) is calculated and appended to each transport block. The CRC allows for receiver-side detection of residual errors in the decoded transport block. If the CRC decoder fails, the receiver also assumes that the whole packet has been lost.Our FEC encoding scheme is performed in the following order: K source packets are encoded by fountain codes first to each fountain-encoded packet, a CRC is first added and the packet is encoded by a 6-shift convolution code. So the source data are first encoded jointly over all the sub-carriers by fountain codes, and then encoded separately over one sub-carrier by LDPC plus CRC codes, the proposed system is given in Fig 5. At the receiver, each fountain-encoded packet is first 8-shift convolution decoded when the SNR is equal to or higher than the threshold.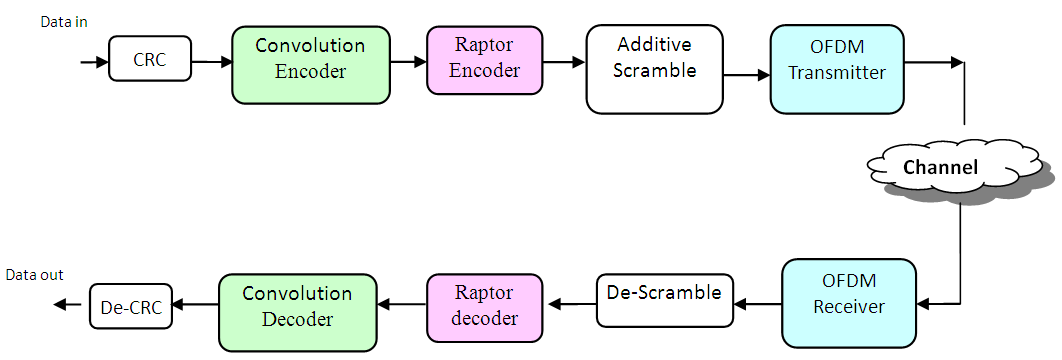 | Figure 5. The proposed model of OFDM-system |
The received packet is discarded if its energy is below the threshold. If the convolution decoding fails, the received packet is discarded as well. If the convolution decoding succeeds, the CRC is used to identify the undetected error from LDPC codes. Once the receiver has collected N surviving fountain-encoded packets, it starts to recover the source data.The proposed system model offers a good trade off of reduced complexity with some performance losses at high UE speeds. This model can be used to further reduce the operational complexity and also the power consumption. This system allows an extremely flexible code design that can be tailored to achieve very high throughput due to the inherent parallelism of the decoding algorithm while maintaining good error-correcting performance and low complexity.
5. Simulation Results
We evaluate the performance of our adaptive protocols in dynamic channel environments over adaptive white Gaussian noise (AWGN) channel and adaptive modulation techniques. The bit error rate and the noise power Vs SNR are plotted for SNR varying from 0 dB to 15 dB. It is found that as SNR increases the bit error rate performance improves. The performance of the system has been depicted in the below Fig 6.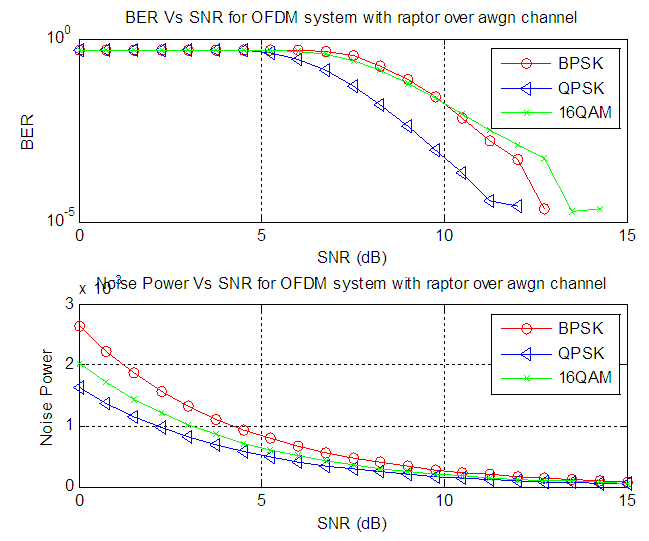 | Figure 6. OFDM system with Raptor Codes on AWGN Channel |
As illustrated in Fig 7, In case of Rayleigh is fading occurring on AWGN of OFDM system with Raptor code, signal to noise ratio (SNR) achieves very good and close Bit Error Rate (BER) error performances, consumed relatively low signal power suitable for many applications with low complexity design for different values of Doppler shift as an example in (a) FD=0, (b) FD=100, (c) FD=150, (d) FD=250 and adaptive modulation techniques BPSK,QPSK and QAM.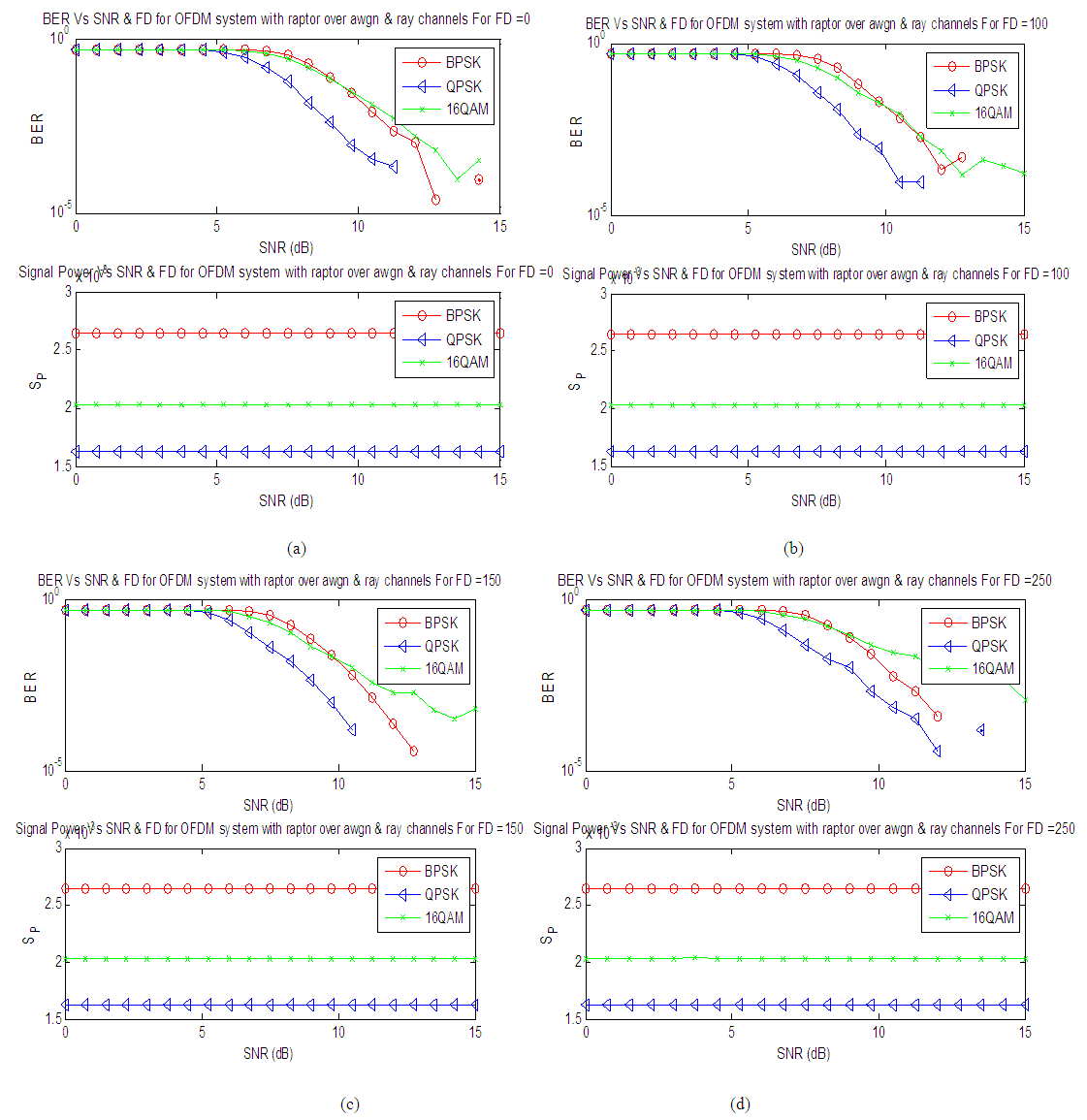 | Figure 7. OFDM system with Raptor Codes on AWGN and Rayleigh Channel for different Doppler shift and adaptive modulation techniques |
Fig 8, shows the variation of the throughput with the signal to noise ratio and the Doppler shift for the proposed OFDM system with Raptor codes FOR adaptive modulation techniques. The throughput approximately tends toward 100%.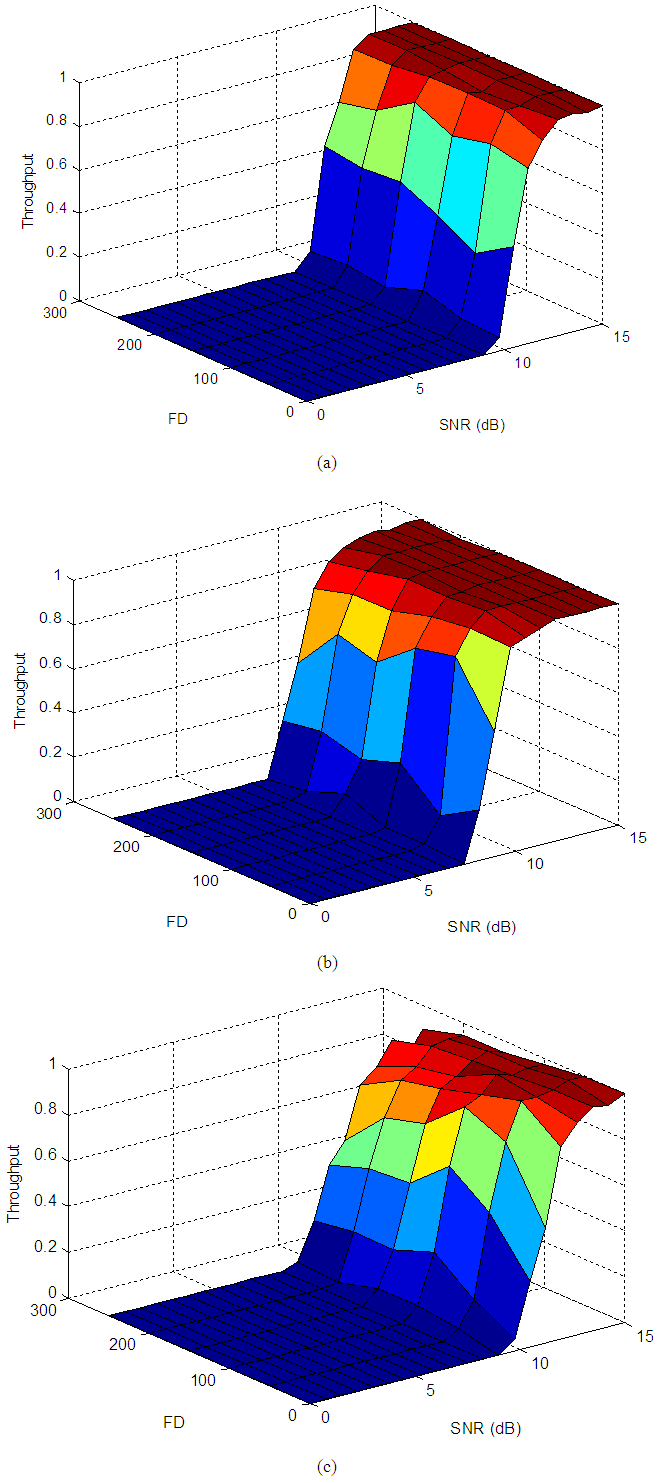 | Figure 8. Throughput of OFDM system with Raptor Codes on AWGN and Rayleigh Channel for adaptive modulation techniques (a) BPSK, (b) QPSK and (c) QAM |
6. Throughput Analysis with Raptor Consideration
In this section, the throughput analyzing is presented with the utilizing of the Raptor code is considered also. The previous section presents the computer simulation experiments and its results. These experiments are carried out using the Zero forcing equalizer, it evaluated the proposed technique over the OFDM system with the modulation technique variation. The throughput can be considered one of a vital metric for measuring the communications system performance. It is defined as the amount of correcting data success to be received at a time interval. So, based on this definition, the throughput mathematical equation can be expressed as follows:-This section presents an analysis for the standard and proposed packet formats. Also, the effect of the proposed scheme on the system performance is studied. The throughput performance is affected by the Packet Error Probability (PEP) which is related to the packet size as follows.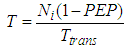 | (4) |
The throughput can be defined as the amount of data transferred from the source to the destination or processed in a specific amount of time. It is also defined as the average number of information bits per second that are transmitted, successfully. It can be expressed as follows: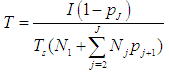 | (5) |
Where
is the symbol period, I is th amount of transmitted data by bits, and
is the probability of decoding failure in transmissions 1 through
. That is,
Where the BLER denotes the block error rate achieved after the
transmission.The average packet delay, δ, is the expected time that elapses from the moment the packet is first transmitted over the channel to the moment the packet is successfully decoded. It is given by: 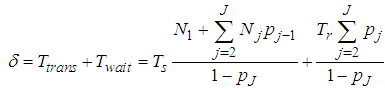 | (6) |
Where,
is the average cumulative time between transmissions of a packet until successful decoding, and
is the time interval between transmissions. Reducing the number of retransmissions can increase throughput. Also, increasing the number of retransmission leads to higher packet delays.Based on the fountain code basics, the transmitted packets won't be accepted to retransmit. It will be transmitted number of times, let Q times. So, the throughput amount can be calculated by I/(Q.Ttrans).
7. Conclusions
In this paper, we analysis the performance of wireless OFDM system using Raptor codes. Raptor code is the new emerging rate less code which has shown amazing performance over a variety of channels. We investigated the effect of AWGN and Rayleigh channels on the OFDM system based on Raptor codes. The simulation result based on different modulation technique using Raptor codes for BER calculation. The modulation techniques used are BPSK, QPSK and QAM. The fountain codes as a ruthless coding scheme find its application in a broadcasting environment, especially when the channel introduces erasures. The effectiveness of the fountain coding scheme improves as the number of transmitting packets increases as the overhead reduced with an increase in the number of transmitting packets. The simulation allows an extremely flexible code design to achieve very high throughput.
References
[1] | H. Harada and R. Prasad, “Simulation and Software Radio for Mobile Communications,” Published by Artech House, 31 Mar. 2002, Universal Personal Communications library. |
[2] | R.V. Nee & R. Prasad, “OFDM for Wireless Multimedia Communications”. Artech House Publishers, 2004. |
[3] | R.G. Gallager, “Low Density Parity Check Codes", Cambridge: MA: MIT Press, 1963. |
[4] | D.J.C. Mackay, “Fountain Codes", IEE Proc.-Commun. Vol. 152, No.6, December 2005. |
[5] | M. Luby, “LT-Codes", Proceedings of the 43rd Annual IEEE Symposium on the Foundations of Computer Science, pp. 271-280, 2002. |
[6] | Amin Shokrollahi, “Raptor Codes", IEEE Transaction on Information Theory, Vol. 52, N0. 6, June 2006. |
[7] | M. Rahman, S. Dash, F. Fitzek, OFDM based WLAN systems, Technical Report, Aalborg University, Denmark, February 2005. |
[8] | Michael Luby, Mark Watson, Tiago Gasiba, Thomas Stock hammer and Wen Xu, “Raptor Codes for Reliable Download Delivery in Wireless Broadcast Systems”, Proceedings of IEEE CCNC 2006. |
[9] | K. Singh, J. Khurana, Sh. B. Mastnath Engineering College, Rohtak, “Performance Analysis of Wireless OFDM System Using Raptor Codes with different Modulation", July 2014. |
[10] | A. U. Pandya, S. D Trapasiya, S. S. China “Performance Analysis of Al-Fec Raptor Code over 3GPP Embms Network” International Journal of Research in Engineering and Technology, Apr-2013. |
[11] | Dr. Michael B. Pursley, Committee Chair “Adaptive Transmission Protocols for Wireless Communication Systems with Fountain Coding", December 2013. |
[12] | W. Huang, H. Li, and J. Dill "Digital Fountain Codes System Model and Performance over AWGN and Rayleigh Fading Channels" USA. |
[13] | W. Yao, L. Chen, H. Li, and H. Xu, “Research on fountain codes in deep space communication,” Congress on Image and Signal Processing, Vol. 2, 27-30 May 2008, pp. 219-224. |