Mousaab M. Nahas
Electrical and Computer Engineering Department, Faculty of Engineering, University of Jeddah, Jeddah, Saudi Arabia
Correspondence to: Mousaab M. Nahas, Electrical and Computer Engineering Department, Faculty of Engineering, University of Jeddah, Jeddah, Saudi Arabia.
Email: |  |
Copyright © 2016 Scientific & Academic Publishing. All Rights Reserved.
This work is licensed under the Creative Commons Attribution International License (CC BY).
http://creativecommons.org/licenses/by/4.0/

Abstract
This paper investigates possible channeling approaches to optimize the passive indoor DAS network structure, containing both outside and inside plants. The paper shows that the optimal structure for DAS network is achieved by deploying an SMF-based all-optical connection all the way from the base station (BTS) to the distributed remote antenna units located inside the building. Vertical micro-ducted fibers are proposed for the outside plant connection around the buildings such that the deployment work and cost are significantly minimized. For internal connection, the paper demonstrates different fiber topologies and shows that the best design uses star network where each antenna unit is fully independent and has large communication bandwidth. We believe that the system proposed in this research can help telecom operators to improve their radio coverage planning especially in downtown areas where a huge number of indoor mobile handsets are simultaneously operated.
Keywords:
Distributed Antenna System (DAS), Micro-Ducted Fiber, Radio over Fiber (RoF)
Cite this paper: Mousaab M. Nahas, Optimization of Passive Indoor DAS Network Planning Using SMF-Based All-Optical Channeling, International Journal of Networks and Communications, Vol. 6 No. 1, 2016, pp. 8-12. doi: 10.5923/j.ijnc.20160601.02.
1. Introduction
Radio over fiber (RoF) based distributed antenna systems (DAS) have been widely implemented in wireless communications to cover dead spots [1]. In particular, DAS have been able to enhance coverage in areas of weak or diminished coverage, high usage and where traditional cell sites or mini-cell sites cannot be used for aesthetic or zoning reasons. In DAS, antenna modules are geographically distributed instead of being centralized at the same location. Each distributed antenna unit is connected to a central unit via dedicated fiber-optics [2]. However, there are two main applications of DAS: outdoor and indoor DAS. Outdoor DAS are typically deployed in dense urban areas, residential neighborhoods and across parklands, while indoor DAS are deployed in large buildings (such as hospitals, malls, airports, etc.), tunnels, basements and underground car parks and metro stations. In fact, indoor DAS (also called in-building DAS) have recently received great interest because up to 80% of wireless traffic originates from indoor users where people spend most of their time [3]. Moreover, these indoor users are becoming increasingly dependent on their mobile handsets especially in the presence of smart phones over which people now do most of their activities. This of course requires an increased coverage and capacity that is normally shared with other indoor and outdoor users in the same cell. Unfortunately, the mobile communication frequencies do not easily pass through the building obstacles thus the indoor service cannot be satisfactory. Figure 1 shows the regular indoor situation, where the wireless coverage is at risk and may be totally lost in the lowest building levels. 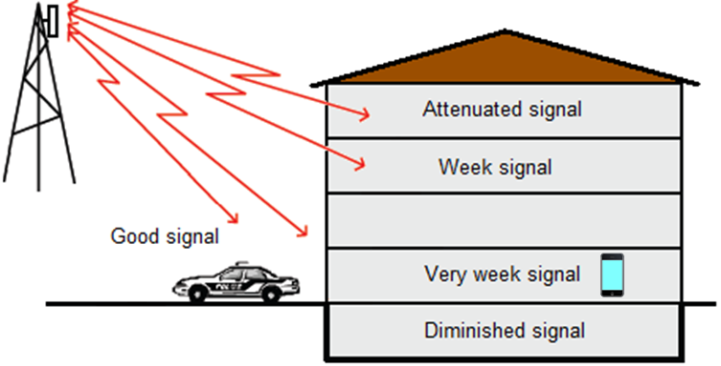 | Figure 1. Indoor coverage situation |
In practice, the transmission of RF signals from cell sites to indoor portable handsets requires a power that is 20 dB stronger than that for the outdoor communications in order to penetrate into or out from a building. In addition, the building penetration loss increases downward at a rate of ~2 dB per floor and the signal isolation between floors is 20 dB on average [4]. This would imply that the indoor coverage provided by outdoor stations [5-6] is not satisfactory due to severe signal attenuations caused by the building walls as stated above. Therefore, good indoor coverage is only provided by indoor DAS where antennas are internally distributed throughout the building, thus high-quality uniform coverage is attained. Ideally, fiber-optics is used as a connecting infrastructure for such antenna network due to its low loss, light weight, high bandwidth, and high immunity to electromagnetic interference [7].The planning of the indoor DAS network must be optimized, which involves finding the number of deployed antennas, their optimal locations and the power transmitted from each antenna. The optimal configuration should eventually minimize the deployment cost and maximize the wireless coverage while minimizing power leakage outside a building. Such optimized planning is already demonstrated [3], [8] where the transmitted power is not divided equally among the antennas but optimized for the antennas according to their locations. However, this planning has only involved optimization of the active part of the inside plant (ISP) of the DAS network. Therefore, it is essential to extend the network planning to contain the outside plant (OSP), which mainly includes the BTS-to-DAS connectivity, and the in-building fiber-optic network topology. This paper is designated to optimizing the whole passive indoor DAS network structure containing both OSP and ISP parts.
2. Indoor DAS Network Components
Indoor DAS network consists of: remote antenna units (RAUs), central wireless equipment and fiber-optics. The RAU is shown in Figure 2. It is a simple, compact, efficient and low-power antenna unit. Multiple RAUs are distributed throughout the building for full coverage. The user is basically connected via the nearest RAU. Each RAU has an antenna connected to a transceiver at which wireless signal is converted to/from optical signal. Typically, RAUs are mounted on walls or ceiling and can be hidden. Thus they provide maximum benefit to the indoor community with minimum changes to its appearance. 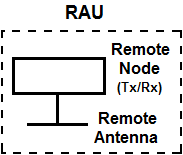 | Figure 2. Remote antenna unit (RAU) |
The central wireless equipment is commonly called a hub or optical master unit (OMU). It is a distribution/collection point for the signals transported to/from RAUs. It may be located within the building or miles away, and most often connected to a base station. Typically, each hub can support up to 36 RAUs. The fiber-optics is used to connect between the RAU and the hub due to the attributes mentioned in the introduction above. A fiber-pair or a single bidirectional fiber can serve multiple RAUs or can be dedicated for single RAU. In fact, bidirectional fibers are more economic than fiber-pairs but the latter are preferred in many cases because forward and backward signals can be totally separated.
3. Indoor DAS Network Planning
3.1. Outside Plant (OSP) Planning
OSP network planning mainly concerns the ways of linking the indoor DAS to the nearest cell tower. It literally involves the passive connection between the BTS and the hub. Such linking can have different approaches as discussed below.
3.1.1. Off-Air Channeling
In this conventional approach, external antenna is used for off-air (wireless RF) connection between the cell tower and the hub which is preferred to be located inside the building or around the edge [9]. This system is shown in Figure 3. It has already been presented in most of the DAS projects, and that is why it is referred to as conventional. Beyond the hub, indoor fiber cables are used to connect between the hub and the RAUs (fiber details are discussed later in the ISP planning). The main drawback of this structure is that the entire indoor DAS largely depends on the quality of the off-air connectivity which may face several impairments especially with buildings that are considerably far from the cell site. This would lead to developing better structure for distant buildings as presented in the next section.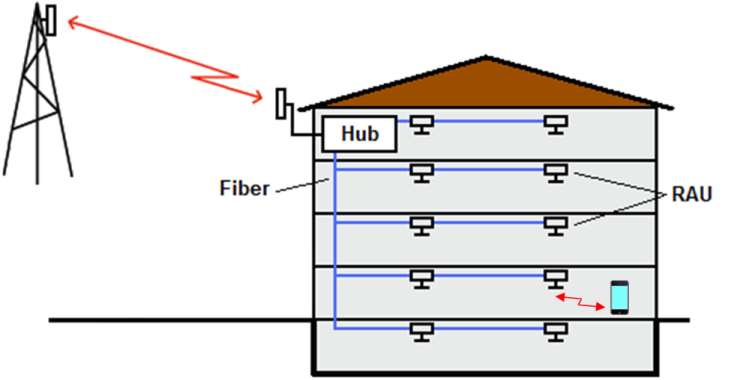 | Figure 3. Off-air channeling approach |
3.1.2. All-Optical Channeling
In this novel approach, the hub is directly connected to the base station thus it is located near the cell tower as shown in Figure 4. The fiber-optics is thus used to connect all the way between the remote hub and the distributed indoor RAUs. Therefore this structure can be referred to as all-optical approach since the cell site is linked with the indoor RAUs all-optically and no off-air connection is involved. This enables avoiding any possible degradation caused by wireless channeling hence more reliable communication throughout the whole DAS network. In practice, such all-optical channel consists of two sections: outdoor and indoor fiber-optics. The outdoor is related to the OSP part while indoor is related to ISP that is discussed in the following section. Typically, single-mode fiber (SMF) cables are used in the OSP network due to the fact that they are more reliable for distances ≥2 km. In addition, fiber-pairs are recommended to be used here rather than single bidirectional fibers due to the advantage mentioned before. In reality, the outdoor connection can make use of the existing fiber infrastructure (if any) and no necessity to deploy outdoor fibers from scratch. This would allow saving a lot of money in the OSP network planning. Otherwise, vertical micro-ducted fiber is recommended to be used where the installation time and cost are significantly minimized [10]. 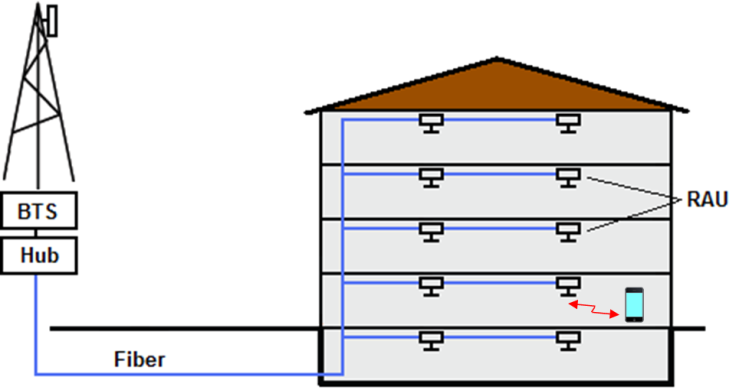 | Figure 4. All-optical channeling approach |
3.2. Inside Plant (ISP) Planning
ISP network planning mainly concerns the possible options for the indoor passive fiber network structure. It literally involves studying the optimal internal fiber-optics topology. In principle, multimode fiber (MMF) cables are recommended to be used in buildings since they are originally designed for short-haul communications. However, the all-optical approach we present here proposes that the whole optical channel including outdoor and indoor cables uses SMF due to that this fiber has become cheap and has much better bandwidth-distance product in comparison with the MMF. This makes the whole network consistent thus no additional losses arise due to coupling issues. This also reduces the complexity of the network hence cost. Moreover, the system can be upgraded easily in the future where the fiber capacity along the entire channel can cope with any potential expansion. The following part presents different options for the indoor fiber topology. Again, fiber-pairs are used with all topologies rather than bidirectional fibers.
3.2.1. Bus Connectivity
This approach uses bus topology as shown in Figure 5. A single fiber-pair is installed to serve the whole building, using optical splitters/couplers at each floor. This system is the cheapest among all topologies but the least redundant, where RAUs in all floors rely on one feeding pair. Also, all RAUs share the bandwidth of the feeding fiber.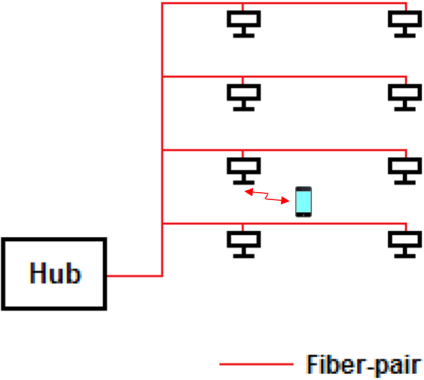 | Figure 5. ISP fiber network using bus topology |
3.2.2. Ring Connectivity
The ring topology can be shown in Figure 6. A fiber-pair ring serves the whole building. This system is also cheap but more redundant than the previous one, where each half of the ring can be a backup path for the other half in case of a break. However, the RAUs are still dependent on main feeders and share the capacity. 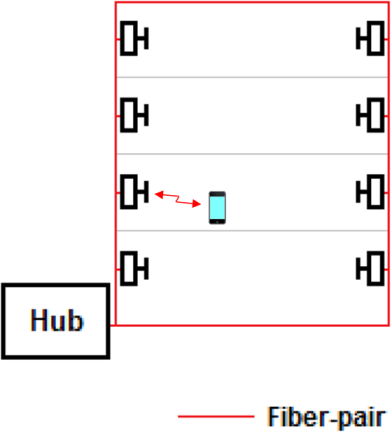 | Figure 6. ISP fiber network using ring topology |
3.2.3. Star Connectivity
This option uses star topology as shown in Figure 7. Single fiber-pair is installed and dedicated for each RAU. Although this system is costly, it is typical for our all-optical network as it is highly redundant thus huge expansion is possible. Also, each RAU is fully independent and can utilize the maximum bandwidth all the way from the hub. 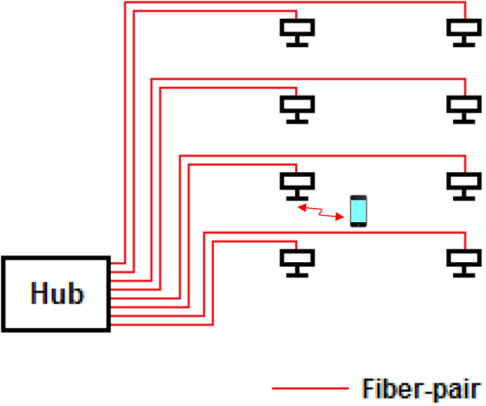 | Figure 7. ISP fiber network using star topology |
3.2.4. Mixed Topology
This is another option where it uses a mixture of star and bus topologies as in Figure 8. Single fiber-pair serves multiple RAU on each floor rather than a dedicated pair for each RAU. Apparently, this system has less redundancy and independency than star approach but however it is considerably cheaper. 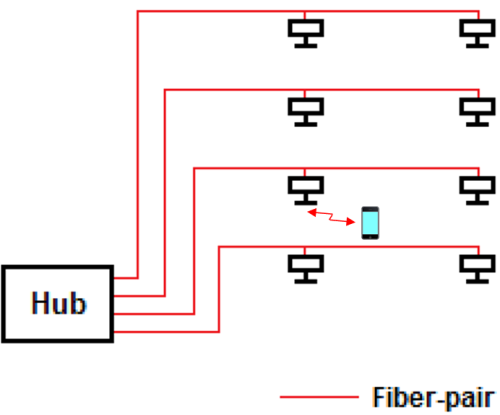 | Figure 8. ISP fiber network using hybrid star-bus topology |
For additional saving, it is also possible to use single remote node/transceiver to serve multiple antennas as shown in Figure 9. In this case, coaxial cable is used to connect the transceiver to the antennas of the same floor. This option saves some components and can also apply to the bus connectivity option presented above. 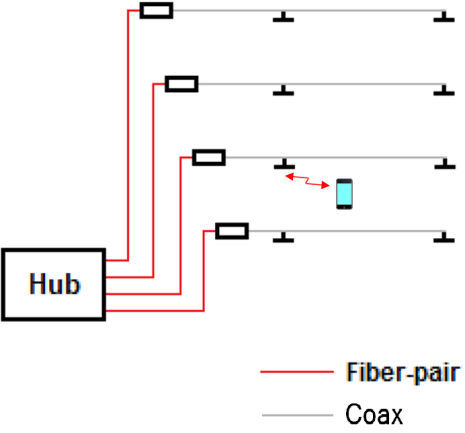 | Figure 9. ISP fiber-coax network using hybrid star-bus topology |
In summary, the star topology (shown in Figure 7) is the best option that we recommend in this particular research due to its high redundancy, independency and bandwidth. It can be considered a life-long solution.
4. Complete All-Optical DAS Structure
Having planned the OSP and ISP networks, it is possible to demonstrate the entire DAS structure that is basically a combination of the two plants. The complete structure is shown in Figure 10. Obviously, it consists of multiple direct SMF-based all-optical channels extending from the cell site down to the indoor RAUs. Since the best structure for the internal fiber network is “star” as stated before, it is crucial to use the same topology in the OSP as well. This would result in that the entire channel is consistent and can benefit from the advantages of the star such as capacity, independency etc. Even though this system is expensive, it is considered optimal in terms of performance and reliability. However, the cost of the network can be significantly reduced by using vertical micro-ducted fiber in the OSP part. In this case, the system shall use multi-channel vertical micro ducts that have multiple high-count cables (feeders) such that each cable serves single building [10]. The number of fiber cores in each feeder depends on the building size where there are cables with 72 cores and cables with 24 cores. Beyond the building edge, 1.6 mm micro cables with 2 cores (pair) are used to connect the individual RAUs inside the building. However, it is advised to install a fiber access terminal (FAT) at each building corner on which the fiber-pairs are spliced into the feeder cores. This would make the structure easy in terms of fiber accessibility, test, maintenance, repair and expansion. To further reduce the entire cost of the DAS network, it is possible to make a hybrid structure which involves both off-air and all-optical channeling. This can be implemented as shown in Figure 11, where the off-air linking is used for buildings located near the cell sites, while all-optical channels are installed for buildings that are far from the towers. This is based on that the coverage and radiation quality around the cell towers are reasonably better than those in distant areas. In other words, the advantages of having all-optical channel can be compromised for the close buildings as long as there is a huge saving in the entire project. However, it is essential at this point to emphasize that what is demonstrated above is a general planning concept on how the passive structure can be improved. This concept should fit comfortably for any indoor DAS project. Therefore, it is the engineer’s role to implement the above proposal based on given project specifications (e.g. distances, buildings size, ducts layout etc.). These specifications are different in every individual project and are usually obtained during the initial site survey. 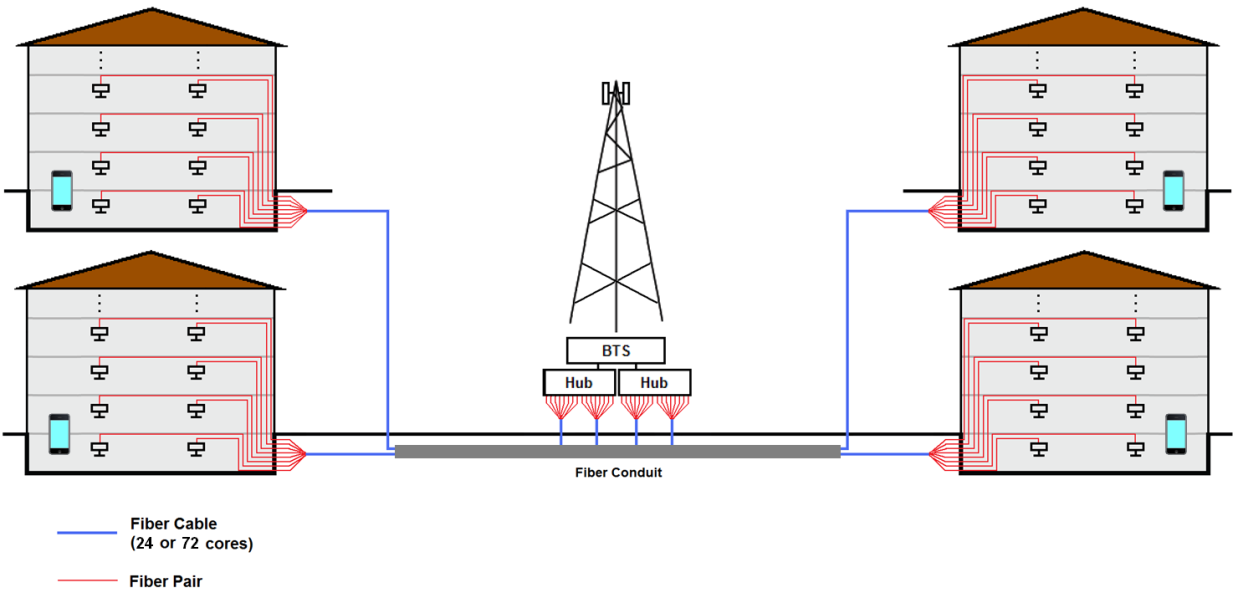 | Figure 10. Complete all-optical DAS structure |
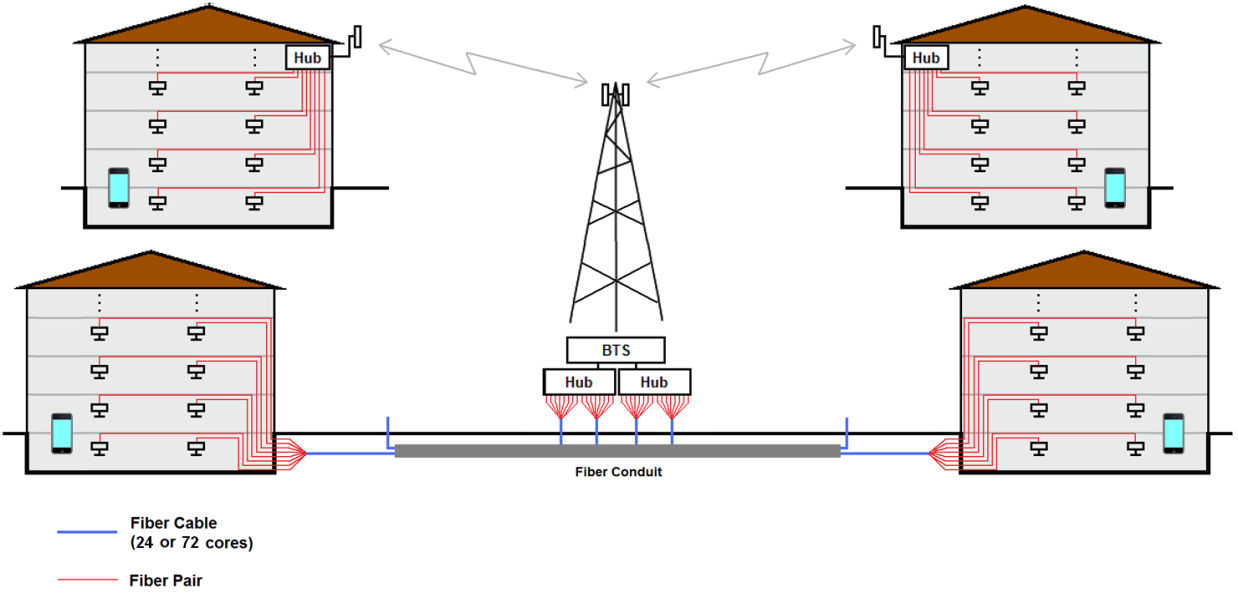 | Figure 11. Hybrid DAS system using both off-air and all-optical channeling; top represents close buildings, while bottom represents far buildings |
5. Conclusions
In this paper, we investigate different possible structures for passive indoor DAS network including both outside and inside plants. For outside plant, we conclude that the best linkage between the cell sites and indoor antenna units is achieved via SMF-based all-optical channeling. This approach is ideal for buildings located far from the BTS where vertical micro-ducted fibers are used to minimize the installation cost and effort. However, wireless RF channeling can still be used for buildings near the towers thus the overall cost of the DAS is further reduced. It is also shown that the best fiber cables distribution is attained via “star” topology where each RAU has a dedicated fiber-pair thus high independency and redundancy. As a result, the in-building coverage is significantly improved especially in far areas without requiring a complicated cell sites or mini-cell sites. This would imply that new service integration is accomplished fast where DAS equipment is easy to upgrade and vertical micro-ducted fiber network is easy to expand.
References
[1] | W. Choi, and J. Andrews, “Downlink Performance and Capacity of Distributed Antenna Systems in a Multicell Environment,” IEEE Transactions on Wireless Communications, vol. 6, no. 1, pp. 69–73, 2007. |
[2] | N. Sheng, J. Zhang, F. Zhang, and L. Tian, “Downlink Performance of Indoor Distributed Antenna Systems Based on Wideband MIMO Measurement at 5.25 GHz,” in Proc. VTC, 2011, pp. 1–5. |
[3] | R. Atawia, M. Ashour, T. El Shabrawy, and H. Hammad, “Optimized Transmitted Antenna Power Indoor Planning using Distributed Antenna Systems,” in Proc. IWCMC, 2013, pp. 993–1000. |
[4] | S. Palit, “Wireless Communications Using Distributed Antenna Networks, Part A: Design Assumptions,” in Proc. APMC, 2008, pp. 1–4. |
[5] | Q. Wu, X. Ding, and A. Chen, “A Broadband Dipole Antenna for Multiservice Indoor Distributed Antenna System (MS-IDAS),” IEEE Antennas and Wireless Propagation Letters, vol. 14, pp. 839–842, 2015. |
[6] | M. Nezam, “In-building Wireless Communication System Design,” in Proc. MIC-CCA, 2008. |
[7] | D. Cutner, J. Georges, and K. Lau, “Is a Fiber-Fed Antenna Network Optimal for In Building Wireless Signal Distribution?,” in Proc. IEEE MTT-S International Microwave Symposium Digest, 1996, vol. 3, pp. 1567–1570. |
[8] | R. Atawia, M. Ashour, T. El Shabrawy, and H. Hammad. “Indoor Distributed Antenna System Planning with Optimized Antenna Power Using Genetic Algorithm,” in Proc. VTC, 2013, pp. 1–6. |
[9] | A. Das, A. Nkansah, N. Gomes, I. Garcia, J. Batchelor, and D. Wake, “Design of Low-Cost Multimode Fiber-Fed Indoor Wireless Networks,” Transactions on Microwave Theory and Techniques, vol. 54, no. 8, pp. 3426–3432, 2006. |
[10] | M. Nahas, “Optimization of Passive FTTH Network Design Using Vertical Micro Ducting, Journal of Telecommunications, vol. 31, no. 1, pp. 19–22, 2015. |