S. Sadhukhan 1, S. Biswas 1, M. Ghosh 1, K. Biswas 2
1Metallurgy and Materials Engineering, Bengal Engineering and Science University, Shibpur, Howrah, 711 103, India
2Metallurgical and Materials Engineering, Indian Institute of Technology, Kharagpur, P.O., 721 302, India
Correspondence to: M. Ghosh , Metallurgy and Materials Engineering, Bengal Engineering and Science University, Shibpur, Howrah, 711 103, India.
Email: |  |
Copyright © 2012 Scientific & Academic Publishing. All Rights Reserved.
Abstract
Al-Zn-Mg based alloys without and with Sc were prepared by remelting the base alloy without and with Al-Sc master alloy in a pot furnace followed by casting in metal mould. Fe and Si often remain as impurities in Al-Zn-Mg alloys. By using optical, scanning electron and transmission electron microscopy, the presence of intermetallics and the hardening effects of micro-alloyed Sc on the microstructure of super-high-strength Al-4.5Zn-2Mg alloys were observed and structure-property relationship was studied. The microstructures of the alloys were investigated for different heat treatment conditions (natural and artificial ageing). Scandium added alloys were harder compared to base alloys in the naturally aged condition by virtue of presence of large number of fine Al3Sc precipitates generated at the time of casting and could not be dissolved during subsequent homogenization treatment. These particles are highly effective in refining the microstructures, retarding recrystallisation, pinning dislocations and low angle grain boundaries. These Al3Sc precipitate act as potent nucleating site for hard η΄ precipitate at the time of artificial ageing and hence suppresses GP zone formation as noticed from the differential scanning calorimetry study. However, the morphology of AlFeSi intermetallics present in cast Al-Zn-Mg alloys was not altered in presence of Sc.
Keywords:
Al-Zn-Mg, Scandium, Ageing, hardness, Precipitates, Intermetallics
Cite this paper:
S. Sadhukhan , S. Biswas , M. Ghosh , K. Biswas , "Al-Fe-Si Intermetallics in Sc-added Cast Al-4.5Zn-2Mg Alloy", International Journal of Metallurgical Engineering, Vol. 1 No. 4, 2012, pp. 44-47. doi: 10.5923/j.ijmee.20120104.01.
1. Introduction
AA7xxx series comprising of Zn as main alloying element along with Mg and Cu in Al-base are widely used in the aircraft industry[1,2] due to high strength to density ratio and good hot workability. Aluminum-zinc-magnesium alloys have the ability to undergo natural ageing resulting higher strength. The strengthening process can further be accelerated by artificial aging. These materials can reach their peak strength through proper T6 aging treatment[3].In spite of high cost, addition of scandium in Al-matrix is done as it has a unique precipitation hardening behavior where it forms a high melting eutectic, very fine precipitates[4], retards the recrystallization[5], forms a coherent equilibrium. precipitate[6] and improves the weldability[7,8]. The strengthening effects of Al3Sc precipitates as well as hardening due to grain refinement were reported[9]. In 7xxx series also, these effects can be exploited to improve the fatigue resistance by grain refinement as well as formation of smaller and more distributed precipitates and improving fracture toughness considerably. Literature[10] also suggests that Al3Sc precipitates influence kinetics of precipitation in age hardenable alloys by acting as preferential nucleation sites hence affecting the size and morphology of the precipitates. They also act as recrystallization inhibitor and homogeneously distributed all over the matrix.There have been many studies of precipitation hardening in these alloys[11,12]; however, the small precipitate size makes determination of the crystallographic and chemical characteristics difficult[13], hence some ambiguity remains over these details and the precipitation sequence. In the present investigation, an effort has been made to study the effects of Sc addition on precipitation characteristics and hardening effect along with the investigation on presence of other precipitates in cast Al-Zn-Mg alloys with the help of DSC, SEM and TEM.
2. Experimental
Al-Zn-Mg base alloys (BA) were prepared by remelting the alloy in laboratory scale from industrially produced ingot. 0.3 wt.% Scandium was added by addition of Al-2 wt.% Sc master alloy in the molten base sample before pouring molten alloy in to the metal mould. To study the kinetics of natural ageing (NA), both BA and Sc added alloys were homogenised at 475℃ for 48 h followed by air cooled. Surface roughness was removed by machining and subsequently solutionized at 475℃ for 1 h and water quenched before naturally aged for 10 days. In order to have a better understanding about the effect of Sc on precipitation sequence and kinetics, both BA and Sc added alloys were artificially aged. Following the same treatment as performed before NA, the alloys were artificially aged at 160℃ for 1 h and water quenched. Age hardening response of the alloys was recorded by Vickers hardness tests using 10 kg load. Samples were polished in cloth followed by etching in modified Keller’s reagent. They were initially characterized by optical microscopy (OM) using Zeiss Optical Microscope. SEM (JEOL-JSM 4000) was used to understand the grain structure and precipitate distribution. The composition of the precipitates was measured by EDX on the fracture surface. Both alloys were investigated by thermal analysis using differential scanning calorimetry (DSC- Mettler T400) following standard methods[13] in order to compare the major transformations that occur during heating. To identify the effect of Sc on morphology and size of precipitates, TEM investigation was done on artificially aged BA and Sc added alloy. Slices of 200-250 µm thick were sectioned from both alloys (base and Sc-modified one) using an Isomet low speed precision cutting saw (Buehler Inc., IL, USA). These slices were further thinned by mechanical polishing on a 600 mesh silicon carbide paper until the thickness was around 80 µm. After cleaning the specimens with acetone and methanol circular disc-shaped samples with 3 mm diameter were punched using a disc punch (Gatan, USA). These discs were subsequently thinned on a dimple grinder (Gatan, USA) using diamond suspension to reduce their thickness to 20 µm. Subsequently, argon ion milling was carried out on a precision ion polishing system (Model: 691, Gatan, USA), by operating the argon ion guns with energies up to 5 KeV at a low angle of about 3-4°. The electron transparent specimens were then examined on a transmission electron microscope (Model: JEM 2100, JEOL, Japan) operated at an acceleration voltage of 200 kV. Table 1 shows the chemical composition of the investigated alloys.Table 1. Chemical composition of the studied alloys (wt%) |
| Alloy | Zn | Mg | Mn | Fe | Si | Ti | Zr | Sc | Al | BA | 4.5 | 2.0 | 0.3 | 0.2 | 0.2 | 0.009 | 0.08 | 0.0 | Bal | Sc added | 4.8 | 1.8 | 0.3 | 0.19 | 0.2 | 0.011 | 0.07 | 0.3 | Bal |
|
|
3. Results and Discussions
3.1. Comparison of Natural Ageing
Fig. 1 depicts the natural ageing response for the BA and Sc added alloys. Sc added alloy possesses higher hardness values compared to the BA irrespective of ageing time. The hardness values were recorded immediately after solutionizing and quenching and continued for 10 days at 24 hours interval. The hardness values of the two alloys increase with ageing time till they saturate after 125 hours of natural ageing and show a plateau. Because the rate of increase of hardness with ageing time are clearly very similar for both alloys, occurrence of higher hardness profile for Sc added alloy is principally due to higher value obtained immediately after quenching. Therefore, it can be said that NA response for both alloys are similar. The difference at the beginning is probably due to difference in precipitate nature influenced by presence of Sc. 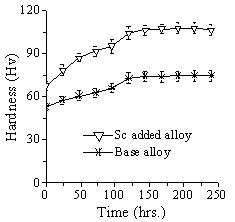 | Figure 1. Natural ageing response of the BA and Sc added alloy |
3.2. Optical and Scanning Electron Microscopy
In order to reveal the morphology of dispersoids and precipitates, optical and scanning electron microscopy was performed. The microstructures of the alloys are shown in fig. 2. Both optical and scanning electron micrographs for the BA (fig. 2a, fig. 2c and fig. 2d) revealed presence of precipitates on the grain boundary as well as in the matrix. However, dispersoids are also present in random. Presence of large amount of precipitates was observed for the Sc added alloys on the grain boundaries after NA for 240 h (fig. 2b).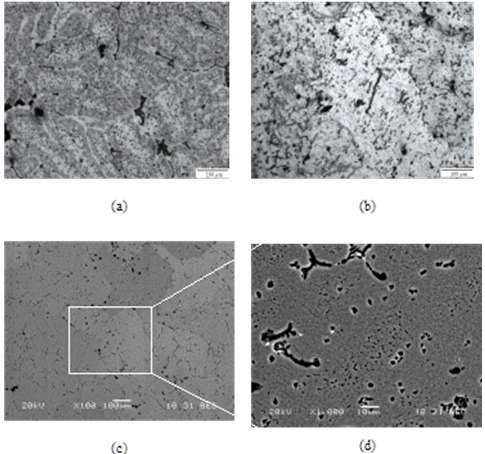 | Figure 2. (a) light micrograph of BA after NA for 10 days (b) light micrograph of Sc added alloy after NA for 10 days (c) and (d) SEM image of BA as after NA of 10 days |
3.3. EDX Results
The EDX study of the BA in naturally aged condition reveals the constituents of the coarser precipitate as shown in fig. 3. These precipitates contain considerable amount of Al, Fe and Si. Similar conclusions regarding the chemistry of the large precipitates for Sc added samples also holds true. Fe in presence of Si in Al base alloys sometimes produces this type of precipitates[15].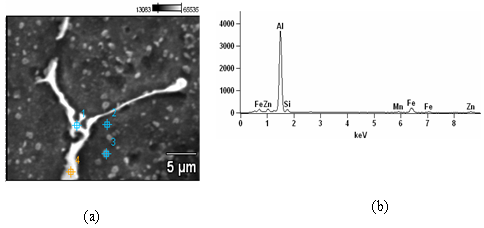 | Figure 3. (a) SEM of the BA after NA showing a large precipitate (b) EDX analysis at point 4 |
3.4. TEM results
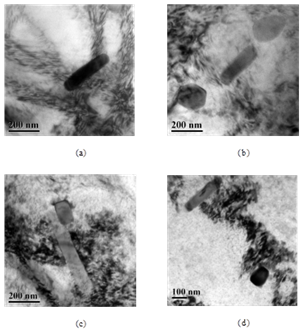 | Figure 4. TEM images (bright field) after artificial ageing (a) and (b) for BA (c) and (d) for Sc added alloy |
Fig. 4 represents the TEM micrographs of the artificially aged samples for both alloys. The microstructure of BA shows (Fig. 4a) dispersoids of different morphologies formed during casting and did not dissolve even after homogenization. These elongated rod and spherical shaped dispersoids are AlFeSi intermetallics which has been confirmed from the EDX analysis presented in fig. 3. These intermetallics show specific habit and act as local stress raiser and accordingly in figure 4a, the strain field around these particles are clearly visible. The alloy matrix is considerably strained and the strain field is distributed more or less randomly. Necessary modification during melting is needed to avoid this type of precipitate formation which affects the mechanical properties of the alloy. Although no change is observed in the morphology of AlFeSi intermetallics due to addition of Sc, but larger number of very fine precipitates in the range of 4 to 6 nm are visible in the Sc added alloy.
3.5. DSC Results
Carrying the knowledge of influence of Sc addition DSC study was performed to investigate the kinetics of precipitation and the results are documented in fig. 5 and fig. 6.and DSC traces for BA and Sc added alloys showing major transformations during heating. The presence of pronounced endotherms is the indication of dissolution of various phase mixtures present in the microstructures. In the DSC curve for BA (fig. 5) the presence of first endotherm indicates the formation of GP zones. At around 180℃, formation of semi coherent η´ precipitates start by dissolving GP zone. However, such characteristics are not observed in Sc added alloys, which can be safely extrapolated to the fact that Sc increases precipitation kinetics thus suppressing GP zone formation. Davydov[12] discussed the anti-recrystallized effectiveness of Sc in Al alloys and attributed it to high density of fine Al3Sc dispersoids formed during decomposition of solid solution homogeneously at 655℃ as stable spherical particles fully coherent with the matrix. The stable coherent Al3Sc precipitates suppress the formation of metastable coherent GP zones in Al-Zn-Mg alloys (Fig. 6]. The curve profile around 250℃ is strikingly similar, which is because of dissolution of η´ precipitates start in both at similar temperatures.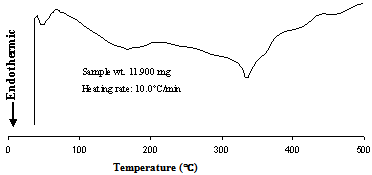 | Figure 5. DSC traces showing major transformations during heating of BA from as-solutionized condition |
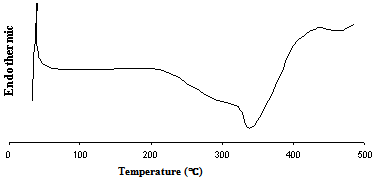 | Figure 6. DSC traces showing major transformations during heating of Sc added alloy from as-solutionised condition |
4. Conclusions
Although both BA and Sc added alloy show similar NA response Sc addition show higher profile due to initial hardness obtained immediately after quenching.Well distributed finer Al3Sc precipitates gives better hardness in the Sc added Al-Zn-Mg alloy in naturally aged condition.Although, Sc modifies the size and number of precipitates, but cannot alter the morphology of Al-Fe-Si intermetallics.Necessary modification during melting should be done to avoid formation of large hard Al-Fe-Si intermetallics, which create strain field around it. The strain field thus created may act as stress raisers in the cast products and adversely affect the properties. , DSC study clearly reveals the suppression of GP zone formation in Al-Zn-Mg alloy with addition of Sc.
ACKNOWLEDGEMENTS
The authors acknowledge University Grant Commission, Government of India, for funding the project.
References
[1] | Kima J. H, Kima J. H., Yeom J. T., Lee D. G., Su Gun Lim, Nho Kwang Park, Journal of Materials Processing Technology, 187–188, pp. 4161-4167, 2007>>> |
[2] | Polmear I.J., Materials Forum, 28, pp. 1-14, 2004 |
[3] | Mukhopadhyay A. K. : Trans. IIM, 62, 2, pp.113-122,2009 |
[4] | Krag M.E., Werbar A, Dunand D.C. and Seidman D.N. : Acta Materialia, 58, pp.134-145, 2010 |
[5] | Hiroki Adachi, Yusuke Yammoto, Hidetaka Nakanishi, Tetsuo Aida, Manabu Imaoka and Jun Kusui: Trans Indian Institute of Metals, 62, 2, pp.159-162, 2009 |
[6] | Nakai M., Eto T., Materials Science and Engineering, A285, pp.62-68, 2000 |
[7] | Wloka J., Virtanen S. : Acta Materialia, 55,pp.6666-6672, 2007 |
[8] | Selvi Dev S, A. Archibald Stuart, R.C. Ravi Dev Kumaar, B.S. Murty, K. Prasad Rao, Materials Science and Engineering A, 467, 1-2, pp.132-138, 2007 |
[9] | Wu Y L., Froes F.H., Alvarez A., Li C G., Materials and Design, 18, 4, pp.211-215, 1997 |
[10] | Baba Y., Trans Japan Inst. Metals, 1976, 6, pp.224-229, 1976 |
[11] | Senkov O.N., S.V. Senkova and M.R. Shagiev, Metallurgical and Materials Transactions A, 39, 5, pp.1034-1053, 2008 |
[12] | Jostein Røyset, Nils Ryum, Diego Bettella, Alessio Tocco, Zhihong Jia, Jan Ketil Solberg, Oddvin Reiso, Materials Science and Engineering: A, 483-484, pp.175-178, 2008 |
[13] | Davydov V.G., Rostova T.D., Zakharov V.V., Filatov Yu. A., Yelagin V.I., Materials Science and Engineering A, 280, pp.30-36, 2000 |
[14] | Mukhopadhyay A.K., Yang Q.B. and Singh S.R., Acta Metall.Mater., 42, pp.3083-3091, 1994 |
[15] | Ringer S.P. and Hono K., Materials Characterization, 44,pp.101-131, 2000 |