Jun Natsuki 1, Toshiaki Natsuki 1, 2, Yoshio Hashimoto 1, 3
1Institute of Carbon Science and Technology, Shinshu University, Nagano, Japan
2Faculty of Textile Science and Technology, Shinshu University, Nagano, Japan
3Faculty of Engineering, Shinshu University, Nagano, Japan
Correspondence to: Jun Natsuki , Institute of Carbon Science and Technology, Shinshu University, Nagano, Japan.
Email: |  |
Copyright © 2015 Scientific & Academic Publishing. All Rights Reserved.
Abstract
We have an interest in inkjet inks containing dispersion of nano-sized silver particles that are useful for producing electronic circuits due to the uniformity of the small silver particles dispersed in inks. In this study, a simple approach to synthesize silver nanoparticles is developed using such as benzoic acid and amines as reducing agents. The silver nanoparticles are characterized using transmission electron microscope (TEM), X-ray Photoelectron spectroscopy (XPS) and so on. The resulting silver nanoparticles by this process are easily separated from the reaction mixture because any organic solvent and external dispersing agent are not used. Uniform silver nanoparticles with a diameter less than 15 nm can be obtained easily that have high electrical conductivity.
Keywords:
Silver nanoparticles, Synthesis, Electrical conductivity, Electronic circuits
Cite this paper: Jun Natsuki , Toshiaki Natsuki , Yoshio Hashimoto , A Simple Approach to Synthesize Silver Nanoparticles and Their Electrically Conductive Property, International Journal of Materials Engineering , Vol. 5 No. 5, 2015, pp. 129-132. doi: 10.5923/j.ijme.20150505.05.
1. Introduction
In recent years, noble metal nano-materials such as gold, silver and palladium have drawn immense attention due to their potential applications as catalysts [1-4], optoelectronic nano-devices [5-6], substrates for surface-enhanced raman spectroscopy (SERS) [7], chemical/biological sensors and biomedicine materials [8-10]. Among various metals, silver nanoparticles are of particular interest because of their remarkable antimicrobial and localized surface plasmon resonance properties, which render them unique applications such as broad-spectrum antimicrobial [11, 12], optical sensor, biomarker [13-15] and so on. Among metal nanoparticles, silver nanoparticles (AgNPs) continue to be interesting in nanotechnology due to their excellent optical and electronic properties as well as their strong toxicity to a wide range of microorganism. We have an interest in inkjet inks containing dispersion of nano-sized silver particles that are useful for producing electronic circuits due to the uniformity of the small silver particles dispersed in inks. Numerous synthetic approaches have been developed for the synthesis of silver nanoparticles including chemical [16, 17], photochemical [18], and biological methods [19]. We have developed several methods to synthesize nanoparticles using Polyvinylpyrrolidone (PVP) polymer and low molecular weight compounds as dispersing agents in our previous work [20, 21]. However, the silver nanoparticles synthesized with PVP polymer need to be sintered to remove the polymer covered on particles at the temperature around 200°C. In this study, we have developed a simple approach to synthesize silver nanoparticles using benzoic acid with hydroxyl group and amines as reducing agents. The resulting nanoparticles by this procedure can be sintered at room temperature by drying the solvent within a short time.
2. Experimental
2.1. Materials
Silver nitrate (AgNO3), triethylamine (Et3N), dimethylaminoethanol (DMAE), gallic acid, dodecyl gallate and 3, 4-dihydroxybenzoic acid, which are obtained from Wako Pure Chemicals Co., Ltd. (Osaka, Japan). Deionized water was used throughout the experiments.
2.2. Synthesis of Silver Nanoparticles (AgNPs)
0.50g of AgNO3 was dissolved in deionized water of 20 ml by stirring at room temperature. An aqueous solution of 0.027 g DMAE was added to the solution, then an aqueous solution of 0.276 g gallic acid in deionized water of 30 ml was simultaneously added dropwise. The mixture was then stirred at room temperature for one hour. The color change of the solution is gradually from white to pale brown. The silver particles were separated from the solution by centrifugation (5000 rpm), washed with deionized water, and then redispersed in 10 ml deionized water. Table 1. Comparison of different amines and reducing agent in the formation of silver nanoparticles 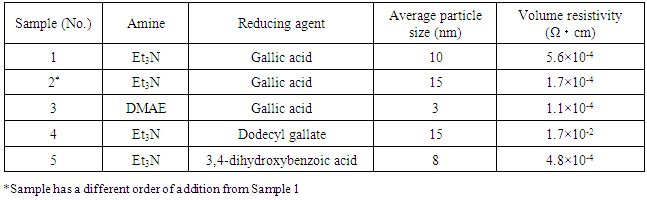 |
| |
|
The 3, 4-dihydroxybenzoic acid and dodecyl gallate were also used to prepare the nanoparticles by only varying the amounts of reactants using the same reaction procedure described above. These samples of silver nanoparticles prepared according to different dispersing or reducing agents are shown in Table 1.
2.3. Characterization
UV-Visible spectra of the silver nanoparticles were obtained with a Hitachi U-4100 UV-Vis spectrophotometer as suspensions in an optical cell. To obtain the image of Transmission electron microscopy (TEM), samples were prepared by placing a drop of the silver suspension on a carbon-coated formvar film over copper grids, and drying at room temperature. The image of the silver nanoparticles was obtained using a TEM (JEM-2100, JOEL, Japan) with an accelerating voltage of 200 kV. The size distribution of silver nanoparticles was also measured with a Zetasizer Nano Series analyzer (Malvern Instruments). X-ray spectroscopy (XPS) measurements were done with Kratos Axis-Ultra DLD, Analytical instrument. To measure volume resistivity, a suspension of silver nanoparticles was coated on a polyimide film. The samples were then dried at room temperature before testing. The volume resistivity was measured with Loresta-GP MCP-T610 resistivity meter (Mitsubishi Chemical Analytech Co., Ltd.). A four-probe method was used to measure the volume resistivity.
3. Results and Discussion
3.1. Synthesis and Characterization of Silver Nanoparticles (AgNPs)
These samples of silver nanoparticles prepared according to different dispersing or reducing agents are shown in Table 1. The Et3N, DMAE, and benzoic acid were used as reducing agents. Their results are also shown in Table 1. It is clear that the silver nanoparticles were obtained with a diameter less than 15nm and low volume resistivity.
3.2. Optical Properties of Silver Nanoparticles
UV-Vis absorption measurement and XPS analysis were used to clarify the formation and the purity of colloidal silver nanoparticles. Figure 1 shows the results of UV-Vis measurement for the synthesized silver nanoparticles. It shows optical absorption peak of silver nanoparticles due to surface plasmon resonance [22]. In Figure 2, the binding energies of Ag (3d3/2) and Ag (3d5/2) are observed at 374 eV and 368 eV, respectively. Since the reported binding energy of Ag0 (3d5/2) is 368.3 eV [23], these peaks prove the formation of silver nanoparticles. Furthermore, no impurities are observed besides small amounts of carbon and oxygen, which indicates that the reagents used have not remained. Because the chemicals used in this study are water-soluble, the final product that is insoluble in water can be separated easily from the reaction mixture. 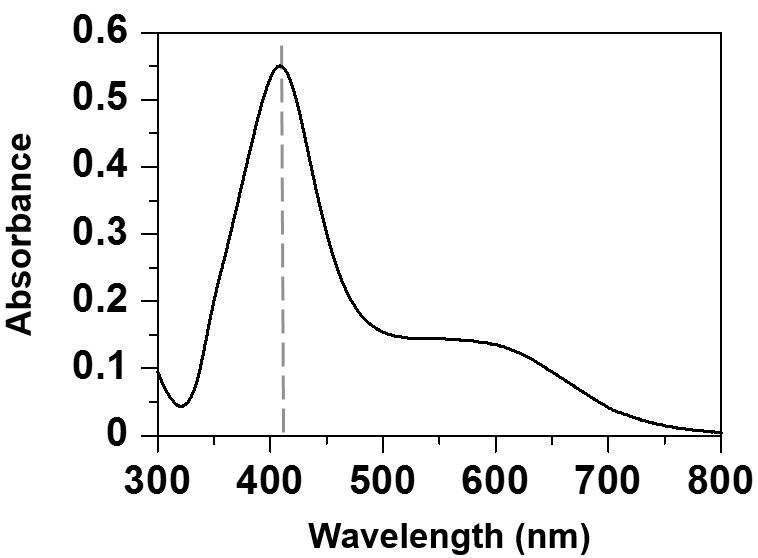 | Figure 1. UV-Vis absorption spectrum of silver nanoparticles suspension |
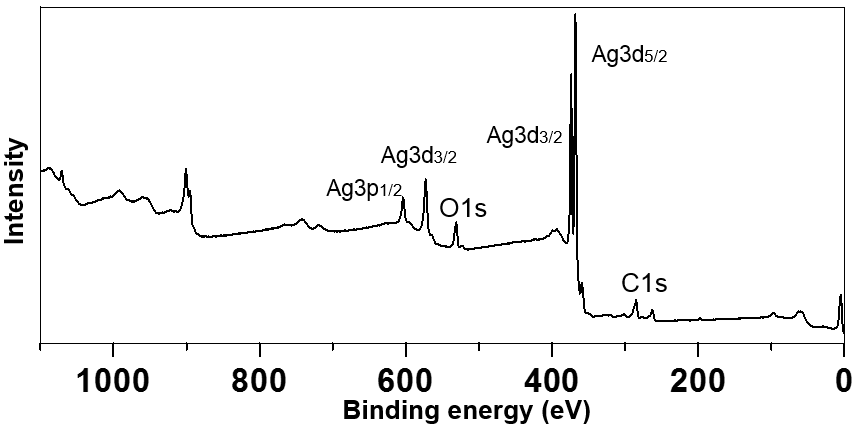 | Figure 2. XPS spectrum of silver nanoparticles |
Figure 3 shows the distribution of the diameters of silver nanoparticles for Sample 1 (see Table 1). The study provides detail knowledge about the silver nanoparticles. The distribution ranges of silver nanoparticles are approximately from 7 nm to 30 nm. The result indicates that the synthesized silver particles are a narrow distribution of diameters and the average diameter of approximately 10 nm. Furthermore, its microstructure observation by TEM is shown in Figure 4. It is observed that the silver nanoparticles are of uniform size.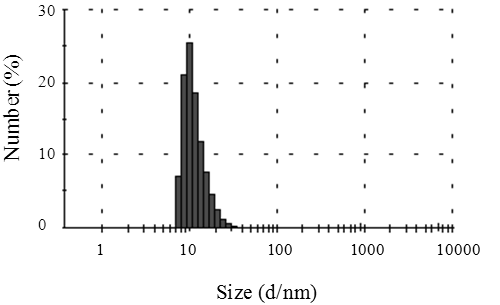 | Figure 3. Diameter distribution of silver nanoparticles |
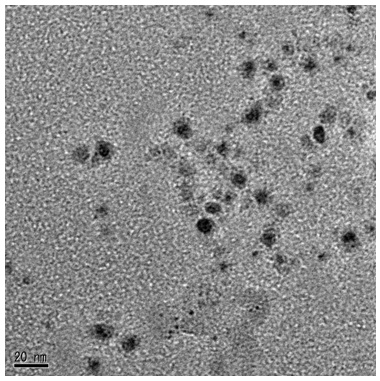 | Figure 4. TEM image of silver nanoparticles |
3.3. The Influences of Amine or Reducing Agent on the Particle Size and the Resistivity of Silver Nanoparticles (AgNPs)
Table 1 shows the influences of amine or reducing agent on the particle size and the resistivity of silver nanoparticles. The amines are mainly used as catalyst in the formation of silver nanoparticles, which can accelerate the reaction. In the present work, the two kinds of amines such as dimethylaminoethanol (DMAE) and triethylamine (Et3N) are selected as catalysts. Samples 1 and 2 have a different order of addition between the Et3N and Gallic acid. The result shows that the order of addition has a little influence on the size and the electrical conductivity of obtained silver nanoparticles. Compared with Samples 1 and 2, the sample 3 obtains the smaller silver particles with a diameter of approximately 3 nm when DMAE and Et3N are used respectively as catalyst and reducing agent. (see Table 1). On the other hand, the size of silver nanoparticles increases when the dodecyl gallate was used instead of the gallic acid (see Sample 4). The reason for this increase is that the particle dispersion decreases due to large steric hindrance of longer side-chain in dodecyl gallate.
3.4. The Electrical Conductivity of Silver Nanoparticles (AgNPs)
To measure the volume resistivity, a suspension of silver nanoparticles was coated onto a polyimide film, and then dried at room temperature. Four-probe method is used to measure the volume resistivity. As shown in Table 1, it is found that these sample films exhibit very high level of electrical conductivity only by evaporation of water at room temperature. In addition, the electrical conductivity of silver nanoparticles with small size is higher than that of silver nanoparticles with larger sizes. This may be explained that molecules of benzoic acid are so small that silver nanoparticles dispersed by the benzoic acid are very nearby each other. These sample films prepared using these nanoparticles give very high electrical conductivity. This is because that the silver nanoparticles have coalesced with each other after drying at room temperature, resulting in improved electrical conductivity. This suggests that benzoic acid with hydroxyl group plays a role in not only reducing agent but also dispersing agent in this process.
4. Conclusions
In summary, we have developed a one-step strategy for synthesizing stable silver colloidal nanoparticles with a uniform small size. This method has a lot of merits: (1) Silver nanoparticles are easily separated from the reaction mixture because any organic solvent is not used. (2) The reaction can occur at room temperature. (3) There is no requirement of other dispersing agents owing to the use of benzoic acid as reducing agent. (4) In particular, a film with high electrical conductivity can be obtained using silver nanoparticles prepared only by drying at room temperature. This is very important to fabrication of electronic circuits. It is expected that electronic circuits can be manufactured by sintering at low temperature due to using the silver nanoparticles prepared by this method.
ACKNOWLEDGMENTS
This work was supported by JSPS KAKENHI Grant Number 10432171.
References
[1] | B. Karimi, F. Mansouri, H. Vali, Green Chem. 16 (2014) pp.2587-2596. |
[2] | Z. Lin, Carbon 86 (2015) pp.301-309. |
[3] | Y. Lu, Y. Wang, W. Chen, J. Power Sources 196 (2011) pp.3033-3038. |
[4] | Y. Lu, W. Chen, J. Power Sources 197 (2012) pp.107-110. |
[5] | T. Tao, J. Geng, L. Hong, W. Huang, H. Tanaka, D. Tanaka, T. Ogawa, J. Phys. Chem. C 117 (2013) pp.25325-25333. |
[6] | S. Smitha, K. Gopchandran, N. Smijesh, R. Philip, Progr. Nat. Sci. Mater. 23 (2013) pp.36-43. |
[7] | M.P. Konrad, A.P. Doherty, S.E. Bell, Anal. Chem. 85 (2013) pp.6783-6789. |
[8] | G.A. Evtugyn, R.V. Shamagsumova, P.V. Padnya, I.I. Stoikov, I.S. Antipin, Talanta127 (2014) pp.9–17. |
[9] | F. Wang, X. Liu, C.-H. Lu, I. Willner, ACS Nano 7 (2013) pp.7278-7286. |
[10] | N. Alon, Y. Miroshnikov, N. Perkas, I. Nissan, A. Gedanken, O. Shefi, Int. J.Nanomed. 9 (2014) pp.23. |
[11] | S. Chernousova, M. Epple, Angew. Chem. Int. Ed. 52 (2013) pp.1636-1653. |
[12] | S. Poyraz, I. Cerkez, T.S. Huang, Z. Liu, L. Kang, J. Luo, X. Zhang, ACS Appl. Mater. Interfaces 6 (2014) pp.20025-20034. |
[13] | Y. Bu, S. Lee, ACS Appl. Mater. Interfaces 4 (2012) pp.3923-3931. |
[14] | P.J. Rivero, A. Urrutia, J. Goicoechea, I. Matias, F. Arregui, Sens. Actuators B 187(2013) pp.40–44. |
[15] | R.P. Singh, P. Ramarao, Toxicol. Lett. (2012) pp.249-259. |
[16] | K.D. Bhatte, K.M. Deshmukh, Y.P. Patil, D.N. Sawant, S.-I. Fujita, M. Arai, B.M.Bhanage, Particuology 10 (2012) pp.140-143. |
[17] | M. Haneda, A. Towata, Catal. Today B 242 (2015) pp.351-356. |
[18] | T. Radu, D. Benea, R. Ciceo-Lucacel, L. Barbu-Tudoran, S. Simon, J. Phys. Chem.C 116 (2012) pp,17975-17979. |
[19] | P. Nalawade, P. Mukherjee, S. Kapoor, Spectrochim. Acta A 129 (2014) 121-124. |
[20] | J. Natsuki and T. Abe, J. Colloid. Interface Sci. 359 (2011) pp.19-23. |
[21] | J. Natsuki, T. Natsuki and T. Abe, J. Nanopart Res. 15 (2013) pp.1483-1-8. |
[22] | K.S. Chou, Y.S. Lai, Mater. Chem. Phys. 83 (2004) pp.82-88. |
[23] | S. He, J. Yao, S. Xie, H. Gao, S. Pang, J. Phys. D. 2001, 34, pp.3425-3429. |