Nargis A. Chowdhury1, John Robertson2, Ahmed A. Jumaily1, Maximiano V. Ramos3
1Institute of Biomedical Technologies, Auckland University of Technology, Auckland, New Zealand
2School of Applied Sciences, Auckland University of Technology, Auckland, New Zealand
3School of Engineeringc, Auckland University of Technology, Auckland, New Zealand
Correspondence to: Nargis A. Chowdhury, Institute of Biomedical Technologies, Auckland University of Technology, Auckland, New Zealand.
Email: |  |
Copyright © 2014 Scientific & Academic Publishing. All Rights Reserved.
Abstract
This study investigates the use of regenerated cellulose/functionalized carbon nanofiber matrices (RC/FCNF) for controlled subcutaneous drug delivery. These drug impregnated matrices are designed for treatments where drug release is controlled by the application of a low applied voltage to the device. Functionalized carbon nanofibers were used as an additive to increase the electrical conductivity which improves the functionality of the system. Sulfosalicylic acid (SSA) is a highly water-soluble compound with similar chemical functionality to number anti-inflammatory and anti-microbial drugs. SSA loaded RC/FCNF samples were prepared by coating functionalized carbon nanofibers dispersed into regenerated cellulose with polypyrrole. The rates of release of SSA into a pH 5.5 acetate buffer solution at 37°C under applied electric fields and with various polypyrrole coatings were studied. The matrices were shown to have zero order drug release kinetics. The mechanism of drug release from polypyrrole coated matrices under an applied electric field includes expansion of the conductive polymer chain and ionic forces between the polymer and the SSA.
Keywords:
Controlled drug delivery, Diffusion coefficient, Electro-chemical drug delivery, Emergency care, Subcutaneous implant
Cite this paper: Nargis A. Chowdhury, John Robertson, Ahmed A. Jumaily, Maximiano V. Ramos, Controlled Release of Sulfosalicylic Acid from Regenerated Cellulose / Functionalized Carbon Nanofibers / Polypyrrole Matrices, International Journal of Materials and Chemistry, Vol. 4 No. 5, 2014, pp. 101-108. doi: 10.5923/j.ijmc.20140405.01.
1. Introduction
Drugs can be introduced into are body by several routes include oral, injection, and transdermal. Oral and injection routes rely on the formulation of the drug and once administered the levels of the medication cannot be modified [1]. Significant research has been done on transdermal drug delivery systems using electro-sensitive polymers but still the polymers are not capable of delivering drugs in sufficient amount because of their low electrical conductivity [2], extremely low drug release rate from the matrices, and the low permeability of most drugs through the skin [3]. As a result, their real application as transdermal drug delivery systems is limited. The existing drug delivery devices based on micro-electromechanical-systems still have problems with low delivery rates and high power consumption [4]. Several controlled drug delivery devices have been investigated for the treatment of chronic diseases. The drug release mechanism from these devices is based on diffusion and a complete release is achieved within 10-12 hours [5]. Current implantable drug delivery devices are not capable of delivering drugs for immediate treatment of illnesses in emergency care situations. The challenge lies in the development of implantable devices for emergency treatments that require initial high release of drug delivery followed by a constant drug release with time. In this study, we developed matrices consisting of regenerated cellulose/functionalized carbon nanofibers / polypyrrole as transdermal implants for the control of angina and muscle pain. The matrices are capable of an initial high release of drug delivery followed by a constant drug release with time. The main advantages of using carbon nanofibers are that the material can be manufactured in large scale, they are smaller in size than multiwalled carbon nanotubes, and incorporating the nanofibers into polymers is easier. In addition, the known toxicity of the functionalized carbon nanofibers is significantly lower than the toxicity of functionalized carbon nanotubes [6]. In this study 5-sulfo-salicylic acid was used as a model drug compound because of its functionality which has structural elements of a number of anti-inflammatory and antimicrobial drugs. For convenience, in this study, this is referred to as the drug.
2. Matrices Synthesis
2.1. Materials
Cotton linter (DPW 4580), lithium chloride, dimethylacetamide (DMAC) (Anhydrous 99.8%), 2-propanol, and carbon nanofibers were purchased from Sigma Aldrich, USA. 5-sulfosalicylic acid (Fluka) was used as a model drug. Pyrrole, 5-sulfo-salicylic acid dihydrate (SSA), and ferric chloride hexahydrate (Fluka), acetone (Fluka, AR grade), methanol (Fluka, AR grade) were used without further purification except pyrrole.
2.2. Functionalization of Carbon Nanofibers
Functionalized carbon nanofibers (FCNFs) were synthesized by the following procedure. 240 mg carbon nanofibers were added to a mixture of 100 mL HNO3 [2.4 M] and 100 mL H2SO4 [7.6 M] and sonicated for 10 min. The mixture was then refluxed for 3 h at 100°C. The fibers were collected on a 0.2 µm membrane filter; the acid treated nanofibers were washed with deionized water until pH ~ 7 and dried under vacuum for 12 h at 60°C [7].
2.3. Preparation of Functionalized Carbon Nanofibers Dispersed Regenerated Cellulose (RC) Films
0.21 g FCNFs were dispersed into 10 mL dimethylacetamide and added to 20 mL RC solution prepared according to the method of McCormick et al. [8]. To prepare each film, the solution was ultrasonically dispersed at 125 W for 4 hour then 3.5 g of the RC/FCNF mixture was poured into a 10 cm glass petri dish, the solvent was evaporated and the films were then air dried.
2.4. Loading of SSA into the Matrices
The drug analogue (SSA) was loaded into the matrices in two different ways in order to compare the release rate from the matrices. In the first method, SSA was loaded by incubating the matrices with the drug. The drug loading process is as follows. The matrices were immersed in 3% (w/v) aqueous solution of 5-sulfosalicylic acid dihydrate (SSA) for 3 days at 37°C to swell to an equilibrium volume. The fully swollen matrices removed from the solution were blotted with filter paper to remove the surface water. The matrices were then dried in air at room temperature. The amount of drug loaded was determined from the weight difference of matrices before and after drug loading.In the second method, SSA was loaded into the matrices by chemical linkage during coating of the matrices with polypyrrole. The method of synthesis is as follows. RC/FCNF matrices were coated with polypyrrole (PPy) by the adsorption induced chemical polymerization using FeCl3/5-sulfosalicylic acid at 2:1 ratio as oxidant/dopant in aqueous solution. 5-sulfosalicylic acid dihydrate acts as a model anion drug as well as a dopant. 2.0 g (0.03 mol) freshly distilled pyrrole monomer and 3.8 g (0.015 mol) SSA dehydrate were dissolved in 50 mL distilled water. The mixture was stirred vigorously for 20 min at 0℃ in an ice bath. 4.9 g (0.03 mol) FeCl3 dissolved in 50 mL distilled water was slowly added to the mixture at a rate of 5 mL/min and the temperature was maintained at <4℃. RC/FCNF matrices were then immersed in 50 mL of the mixture in a glass petri dish and were left for 24 h in a refrigerator at 4℃. Polypyrrole was formed slowly, and deposited onto the surface of the matrices. Finally, the polypyrrole coated matrices (RC/FCNF/PPySSA) were washed with water, methanol and acetone and vacuum dried for 24 h.
2.5. Drug Release Experiments
2.5.1. Preparation of Acetate Buffer Solution
150 g sodium acetate was dissolved in 1 L of distilled water. Glacial acetic acid (approximately 15 mL) was then added slowly with stirring until a pH of 5.5 was reached [9].
2.5.2. Actual Drug Content
The actual amounts of drug in the RC/FCNF/SSA and RC/FCNF/PPySSA were determined by dissolving 1.1 X 1.0 cm pieces of matrix in 2 mL of dimethylsulfoxide (DMSO). 5 mL of this solution was added to 3.0 mL of the acetate buffer solution and the concentration of SSA determined with a UV-Visible spectrophotometer at 298 nm.
2.5.3. Diffusion Studies under Electric Field
Diffusion studies in in vitro were carried out using the set-up shown in Figure 1. A drug-loaded matrix attached to the cathode (negative) electrode was placed in 5 mL acetate buffer solution of pH 5.5 at 37°C. Electric potentials (0 and 3 V) were applied across the cathode and another carbon anode. The drug was diffused through the matrix into the buffer solution. The total diffusion period was 5 h. A sample of 1 mL of the buffer solution was withdrawn for analysis and an equal amount of fresh buffer solution was added to the cell. 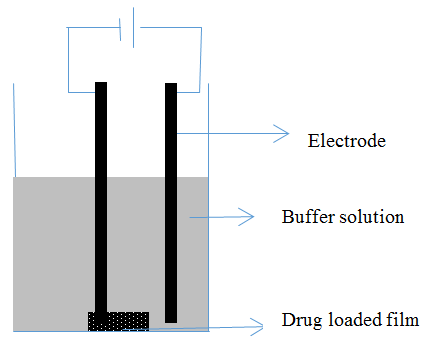 | Figure 1. Experimental set-up used to study electro-responsive drug delivery from implants |
2.5.4. Spectrophotometric Analysis of Model Drug
The maximum absorption spectra of the model drug were recorded using a Shimadzu UV-2101PC scanning spectrophotometer. The absorption at a
of 298 nm was used to determine the amount of drug released from the predetermined calibration curve.
3. Results and Discussion
3.1. Matrices Characterization
Physical, electrical, chemical properties and morphology of the matrices were determined by measuring the degree of swelling, conductivity, infrared absorption spectra, and by comparison scanning electron microscope (SEM) images.The degree of swelling was measured according to the method of Jiang and Quan [10]. The degree of swelling was defined as the water content of the matrices. Percentage degree of swelling was calculated by the following equation: | (1) |
Where, M = weight of the RC/FCNF sample after submersion in the acetate buffer solution, and Md = weight of the sample after removing the solvent. RC/FCNF matrices showed degree of swelling of 159%. The result indicates the porous structure of the matrices. In the drug-loaded matrices, drug diffuses through the gel layer formed by the swelling of the matrices by water. Although matrix swelling favours drug release, it causes strong resistance to drug diffusion through the gel layer. As a result, the amount of drug released decreases with time [11]. In this study, to eliminate this problem, the matrices were coated with polypyrrole doped with drug.Figure 2 shows that polypyrrole is successfully introduced onto the surface of the RC/FCNF matrices.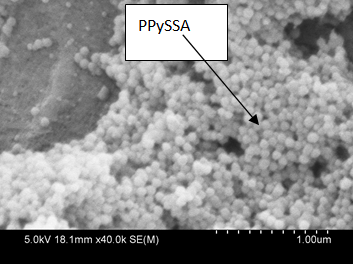 | Figure 2. SEM image of PPySSA coated RC/FCNF matrix |
Electrical conductivity (σs) of the RC/FCNF/PPySSA matrices was calculated by the following equation: | (2) |
Where, l = length, w = width, d = thickness, and ∆I /∆V = the slope of the current versus voltage curve.The electrical conductivity of the RC/FCNF/PPySSA matrices with the dimension of 2.3 cm X 1.0 cm X 60-65 µm film is 0.54 S/cm. The much higher electrical conductivity compared to the pristine cellulose (8.22 X 10-8 S/cm at 50% relative humidity) [12] is due to the presence of FCNFs. In addition, low polymerization temperature (<4°C) that leads to higher polymerization degree and hence to better electrical conductivity [12]. Figure 3 shows the typical current-voltage sweep curve. The conductivity of the matrix essentially follows Ohm‘s law.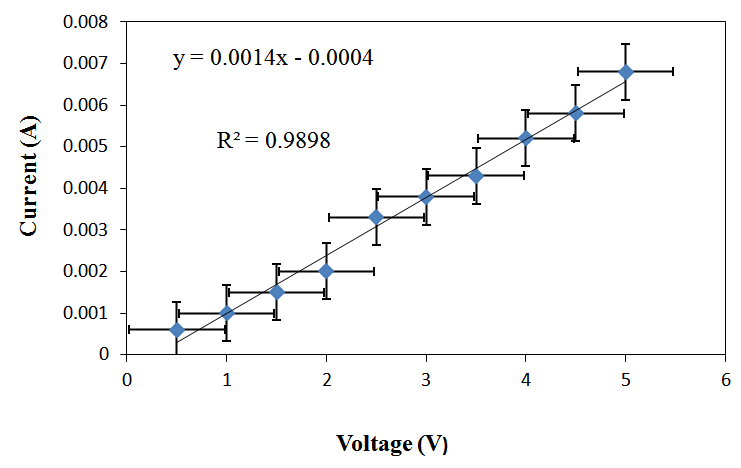 | Figure 3. Current vs. Voltage Curve for RC/FCNF /PPySSA matrix film, y = Current, x = Voltage, R2 = Correlation coefficients |
The absorption infrared spectra of RC/FCNF, RC/FCNF/PPySSA matrices are shown in Figure 4. For RC/FCNF/PPySSA matrices, five-membered PPy ring-stretching and conjugated C–N stretching vibration bands are observed at 1545 and 1429 cm-1, respectively [13]. C- H in-plane vibration is observed at 1310 cm-1. In addition, the peaks at 2915 and 2848 cm-1 are associated with five membered ring C–H stretching [14]. These spectral features indicate that the PPy layer has been successfully introduced onto the surface of the RC/FCNF matrices. In addition, the sulfonate group (R-SO3-) stretching is observed at 1016 cm-1 and the –OH stretching peak has a slight shift between 3000 and 3600 cm-1 because of the H-bonding between the sulfonate groups of sulfosalicylic acid and the amine group of pyrrole unit of PPy.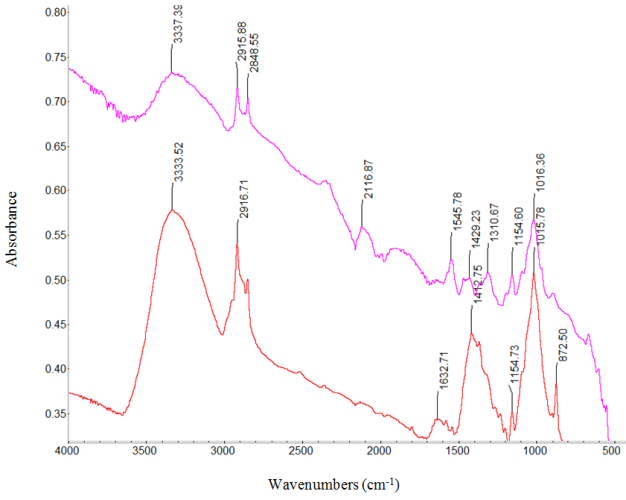 | Figure 4. Absorption infrared spectra of: RC/FCNF/PPySSA matrix film (purple line) and RC/FCNF matrix film (red line) |
3.2. Kinetics of Drug Release Profile
Initially, the actual amounts of drug within the matrices were measured. The amounts of drug present in the RC/FCNF/SSA and RC/FCNF/PPySSA matrices are about 96 ± 1.0 and 90 ± 1.0% of the theoretical amount respectively. The in-vitro release data from RC/FCNF/SSA (3%) and RC/FCNF/PPySSA matrices under an electric field were fitted to various kinetic models such as zero order, first order, and Higuchi release in order to determine the kinetics of drug release. High correlation value was obtained for the zero-order drug release (figure 5 - figure 7). The values of exponent n were obtained with the help of Korsmeyer-Peppas model. The value of the exponent n determines the type of drug release mechanism. Each data point in the plots (figure 5 - figure 9) is an average value from two samples. The drug release rate from the RC/FCNF/SSA (3%) matrices decreases with time at 0 Volt (Figure 5). This is due to the swelling of the matrices. Although matrix swelling increases the surface area of the system, which favors drug release, the resistance to drug diffusion through the gel layer becomes stronger. Consequently, the amount of drug released decreases with time.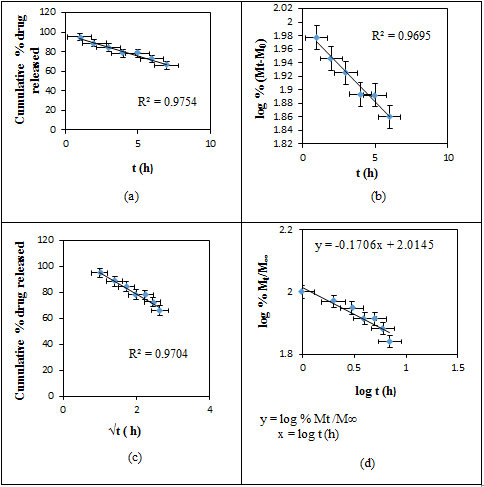 | Figure 5. Plots of (a) Zero, (b) First, (c) Higuchi, and (d) Korsmeyer-Peppas Release Kinetics of SSA from RC/FCNF/SSA (3%) matrices at E = 0 V, pH 5.5, 37°C, R2 = Correlation coefficients |
The drug release rate was increased by applying electric potential. The RC/FCNF/SSA (3%) matrices under electric field follow zero-order kinetics as the correlation coefficient (R2 value) was higher for zero order release (Figure 6).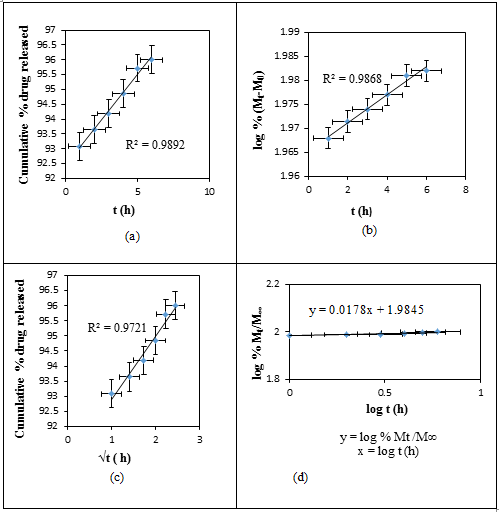 | Figure 6. Plots of (a) Zero, (b) First, (c) Higuchi, and (d) Korsmeyer- Peppas Release Kinetics of SSA from RC/FCNF/SSA(3%) matrices at E = 3V, pH 5.5, 37°C, R2 = Correlation coefficients |
Simultaneous swelling and diffusion through matrices may provide an almost constant release rate. In this study, to provide controlled drug release, RC/FCNF matrices were also coated with polypyrrole doped with the drug. Under and electric field these RC/FCNF/PPySSA matrices also follow the model for zero-order drug release (Figure 7).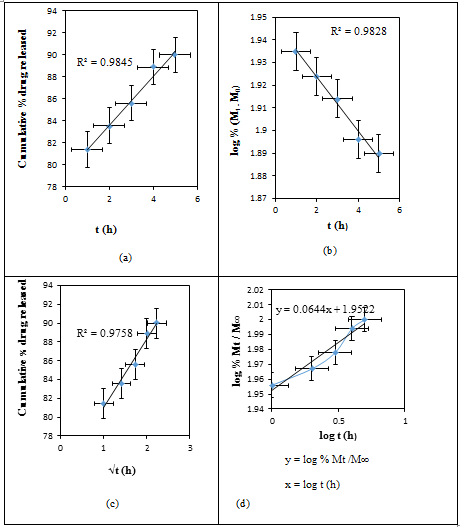 | Figure 7. Plots of (a) Zero, (b) First, (c) Higuchi, and (d) Korsmeyer- Peppas Release Kinetics of SSA from RC/FCNF/PPySSA matrices at E = 3V, pH 5.5, 37°C, R2 = Correlation coefficients |
The release mechanism of drug from the RC/FCNF/ PPySSA matrices includes expansion of polypyrrole chain and the electrostatic force between electron and the drug. As the external electric field is applied, the PPy is reduced. As a result, PPy chains are expanded, a free space in the PPy matrix is generated and the ionic drug migrates out under the applied electric field. Consequently, it increases the amount and the release rate of drug.Figure 8 shows that conductive polypyrrole has great impact on the release rate of SSA (drug) from the RC/FCNF/PPySSA matrices. The diffusion of SSA from sample 1 (RC/FCNF/PPySSA) is less than that of the sample 2 (RC/FCNF/SSA) initially due to the ionic interaction between the anionic drug and polypyrrole. In RC/FCNF/PPYSSA matrices, drug molecules are chemically bonded with polypyrrole. Here polypyrrole bind and release drug in response to the applied field. Polypyrrole has two redox states. Drug ions bound in one redox state are released from the other under electric field. The attached electrodes act to switch the redox states. For sample 2, negatively charged drug molecules migrate from the matrices at the cathode (negative) electrode. These results suggest that the drug release rate can be controlled by coating the RC/FCNF matrices with polypyrrole (sample 1) when compared to the RC/FCNF/SSA (sample 2), which shows fast release.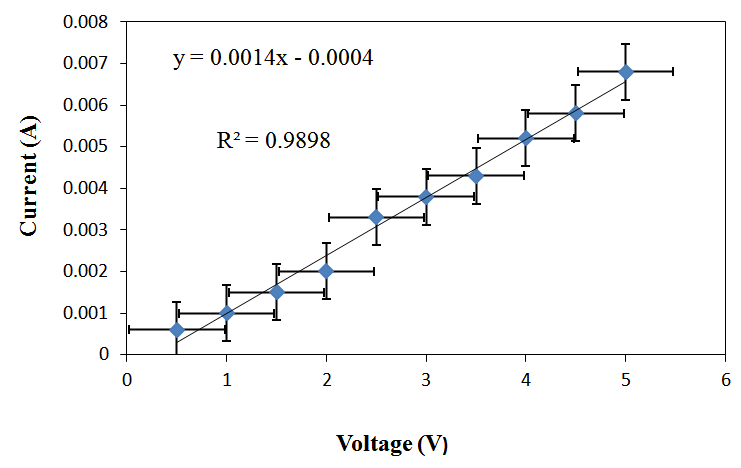 | Figure 8. Plots of cumulative % drug released vs t (h) for RC/FCNF/PPySSA film (Sample 1), and RC/FCNF/SSA film (Sample 2), E = 3V, pH 5.5, 37°C |
In this study, the rate of drug released is increased by increasing the applied electric field (Figure 9). For RC/FCNF/SSA matrices, electrophoresis of charged drugs towards oppositely charged electrodes causes drugs to be released [9]. The amounts of drug released are effectively increased with the increase of the applied electric field due to the conductivity of the carbon additives. At voltages more than 3, matrices have deswelled to a certain extent, as a result, their resistance to the passage of charge increases as the content of free water decreases. Consequently, charge passing through the matrices is proportionally smaller [15]. Therefore, 3 V has been found to be the optimum voltage for increasing the rate of drug delivery.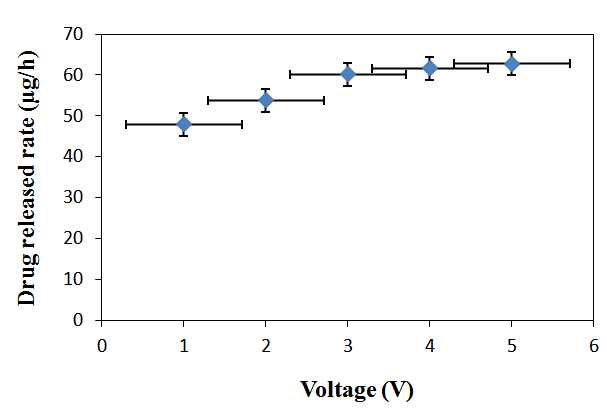 | Figure 9. Plot of drug release rate vs. voltage from RC/FCNF/SSA matrices, E = 1-5 V, pH 5.5, 37°C |
The diffusion coefficients of sulfosalicylic acid from the matrices are calculated according to the following equation [16]: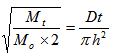 | (3) |
Where, Mt is the amount of drug released at time, t; Mo is the initial drug concentration in the matrices; D is the diffusion coefficient of the drug; and h is the thickness of the matrices. The diffusion coefficient of the drug from RC/FCNF/SSA matrices is higher (4.2 X 10-8 cm2 s -1) than that of the RC/FCNF/PPYSSA (1.4 X 10-8 cm2 s-1) matrices. Polypyrrole can hold quantities of drug within its nanoporous coatings, while at the same time regulating drug transport.
4. Conclusions
The comparison of the release mechanism of sulfosalicylic acid from the RC/FCNF/SSA and RC/FCNF/PPYSSA matrices in the passive release characteristic (E = 0 V), and under electric field was investigated in this study. At E = 0 V, for RC/FCNF/SSA matrices, the rate of drug released was decreased with time due to the matrix swelling. Therefore, in this study, the rate of drug released was controlled by varying the electric field strength. The rate of drug release was a function of the applied electric potential due to the conductivity of the carbon additives. Further, the drug release rate was found to be easily controlled by coating the RC/FCNF matrices with polypyrrole.
ACKNOWLEDGEMENTS
The research was financially supported by the Institute of Biomedical Technologies, and technically supported by the School of Applied Sciences, Auckland University of Technology, Auckland, New Zealand. The authors are grateful to Senior Research Officer Patrick Conor from faculty of Design & Creative Technologies for helping in taking SEM images.
References
[1] | Xiaoping Zhan, G.T., Sijing Chen, Zhenmin Mao., A new copolymer membrane controlling clonidine linear release in a transdermal drug delivery system. Int J Pharm, 2006. Vol. 322(No. 1-2): p. p.1-5. |
[2] | Thacharodi D, R.K., Rate-controlling biopolymer membranes as transdermal delivery systems for nifedipine: development and in vitro evaluations. Biomaterials, 1996. Vol. 17(No. 13): p. p. 1307-11. |
[3] | Li W, N.D., Rasmussen HT, Patel K, Shah T., Sample preparation optimization for assay of active pharmaceutical ingredients in a transdermal drug delivery system using experimental designs. J Pharm Biomed Anal, 2005. Vol. 37(No. 3): p. p. 493-98. |
[4] | Elman NM, H.D.H., Cima MJ, An implantable MEMS drug delivery device for rapid delivery in ambulatory emergency care. Biomed Microdevices, 2009. Vol. 11(No. 3): p. p. 625-631. |
[5] | Chaturvedi S, K.P., Agrawal V. K., Polysaccharides and gums as reservoir for a sustained delivery of tramadol hydrochloride by hydrodynamically balanced systems. International Journal of Pharmacy and Pharmaceutical Sciences, 2013. Vol. 6(No. 1). |
[6] | Arnaud Magrez , S.K., Valérie Salicio, Nathalie Pasquier , Jin Won Seo, Marco Celio, Stefan Catsicas , Beat Schwaller, László Forró, Cellular toxicity of carbon-based nanomaterials. Nano Lett, 2006. Vol. 6: p. p. 1121-1125. |
[7] | Sungryul Yun, J.K., Characteristics and performance of functionalized MWNT blended cellulose electro-active paper actuator. Synthetic Metals 2008. Vol. 158: p. p. 521–526. |
[8] | Charles L. McCormick , P.A.C., Brewer H. Hutchinson Jr. , Solution studies of cellulose in lithium chloride and N,N-dimethylacetamide. Macromolecules, 1985. Vol. 18(No. 12): p. p. 2394–2401. |
[9] | Kanokporn Juntanon, S.N., Ratana Rujiravanit, Anuvat Sirivat., Electrically controlled release of sulfosalicylic acid from crosslinked poly(vinyl alcohol) hydrogel. International Journal of Pharmaceutics 2008. Vol. 356: p. p. 1-11. |
[10] | Jiang Wu, Q.Y., Gas permeability of a novel cellulose membrane. Journal of Membrane Science., 2002. Vol. 204(No. 1-2): p. p.185-194. |
[11] | Cristina Maderuelo , A.Z., José M. Lanao., Critical factors in the release of drugs from sustained release hydrophilic matrices. Journal of Controlled Release, 2011. Vol. 154: p. p. 2-19. |
[12] | Suresha K Mahadeva, J.K., Enhanced electrical properties of regenerated cellulose by polypyrrole and ionic liquid nanocoating. Journal of Nanoengineering and Nanosystems, 2011. Vol. 225(No. 1): p. p. 33-39. |
[13] | Robert B. Rosner , M.F.R., Solid-state polymerization polypyrrole within a Langmuir-Blodgett film of ferric stearate. Chem. Mater, 1994. Vol. 6: p. p. 581–586. |
[14] | Naoki Toshima, O.I., Catalytic synthesis of conductive polypyrrole using iron (III) catalyst and molecular oxygen. Synth. Met., 1996. Vol. 79: p. p. 165–172. |
[15] | Murdan, S., Electro-responsive drug delivery from hydrogels. Journal of controlled release, 2003. Vol. 92: p. p. 1-17. |
[16] | Ferrero C, M.D., Doelker E. , Towards elucidation of the drug release mechanism from compressed hydrophilic matrices made of cellulose ethers. II. Evaluation of a possible swelling-controlled drug release mechanism using dimensionless analysis. J Control Release. , 2010. Vol. 141(No. 2): p. p. 223-33. |