Luís Gustavo Costa de Castro1, Sérgio Fernando Nunes Júnior1, José Wilson de Jesus Silva2, Eduardo Norberto Codaro1, Heloisa Andréa Acciari1
1Physics and Chemistry Department, Faculty of Engineering, Guaratinguetá, Brazil
2Teresa D'Avila Integrated Faculties, FATEA, Lorena, Brazil
Correspondence to: Heloisa Andréa Acciari, Physics and Chemistry Department, Faculty of Engineering, Guaratinguetá, Brazil.
Email: |  |
Copyright © 2014 Scientific & Academic Publishing. All Rights Reserved.
Abstract
Basic metal alloys, as Ni-Cr-Mo, have been widely used in the manufacture of fixed and removable prostheses, due to their desirable physical and mechanical properties. However, these alloys undergo an oxidation in the oral environment, which causes metal ions release in the body, leading to harmful systemic effects for human health. The purpose of this study is to assess the electrochemical behavior of a Ni-Cr-Mo commercial dental alloy, in a physiological environment that simulates the aggressiveness of oral cavity (0.9% NaCl solution) with its pH varying from 2.0 to 6.0. The corrosion behavior was assessed by electrochemical measurements which are commonly applied in metals’ corrosion study, with quantitative parameters to estimate corrosion resistance. In the open circuit potential curves, it was observed a typical behavior of passive state, mainly at pH 4.0 and 6.0. On the potentiodynamic curves it was observed that the passive current density increased and the re-passivation potential decreased with diminishing pH, suggesting that the re-passivation does not occur at pH 2.0. Chronoamperometric curves, as well as EIS spectra shapes, were consistent with these analyses. The results obtained from different methods of analyses indicate that corrosion resistance can be affected by pH changes in a saline medium.
Keywords:
Ni-Cr alloys, Corrosion, Chloride Ions, Electrochemical Techniques
Cite this paper: Luís Gustavo Costa de Castro, Sérgio Fernando Nunes Júnior, José Wilson de Jesus Silva, Eduardo Norberto Codaro, Heloisa Andréa Acciari, Effect of pH on Electrochemical Behaviour of a Ni-Cr-Mo Commercial Dental Alloy in Chloride Medium, International Journal of Materials and Chemistry, Vol. 4 No. 3, 2014, pp. 69-77. doi: 10.5923/j.ijmc.20140403.04.
1. Introduction
Tooth structure loss has been an issue for a long time in dentistry due to aesthetics and functional problems that affect the health and life quality of patients. Many studies have contributed to new materials development which can restore features and the natural functions of teeth. Basic metal alloys, such as Ni-Cr-Mo, have emerged in the dental market as a practical result of this study being widely used in the manufacture of fixed and removable prostheses, particularly in developing countries, due to their desirable physical and mechanical properties, and also because they are easily processed and inexpensive [1, 2].However, these alloys undergo an oxidation in the oral environment, leading to metal ions release in the body, with systemic effects which are harmful to human health, leaving doubts as to its biocompatibility [1, 3]. Ni, for example, has an allergenic feature and Cr, when present in its chromate form, is highly toxic [1]. Therefore, new formulations of Ni-Cr-Mo alloys were evaluated.Since Ni2+ release causes allergic reactions, less noble alloying elements can also be dissolved causing diseases from a cumulative process that will be manifested only after many years wearing the prosthesis. The quickness in which an ion is released into the corrosive medium is a function of the alloy composition and the solubility of the corrosion products. Therefore, a higher ions release leads to a higher risk of undesirable reactions in the mouth. Even in metal-ceramic restorations, when a ceramic material is fused to the alloy, this problem is not discarded due to atoms inter-diffusion at the interface metal/ceramic interface reaching temperatures of about 1000℃ [4].Corrosion researchers concluded that the Ni amount released into the solution depends on a combination of factors, such as Cr and Mo contents, where these factors are responsible for a decrease in the Ni release due to a formation of Cr and Mo oxides, predominantly Cr2O3 and MoO2, by means of evidence of higher localized corrosion susceptibility in dendritic structures of Mo-free Ni-Cr alloys [5-7].In the oral cavity, these structures are exposed to a chemically adverse environment, due to a high concentration of chloride ions in the saliva composition and also to constant changes in pH and temperature. This process may be enhanced through abrasion from food intake and brushing. An aggravating factor is that, once it is placed in the mouth, it must remain subjected to mechanical stress and corrosion for a long time. In an attempt to simulate these conditions, the electrochemical behavior of these alloys has been commonly assessed in artificial saliva or in solutions with concentrations of chloride ions which are similar to that found in the chemical composition of natural saliva [5, 8-12].In this paper, it is proposed to assess the electrochemical behavior and corrosion resistance of a dental Ni-Cr-Mo alloy in a physiological environment that simulates the aggressiveness of the oral cavity (0.9% NaCl), by varying the pH from 2.0 to 6.0. Electrochemical techniques commonly applied to the study of metallic corrosion provided quantitative parameters to estimate corrosion resistance.
2. Materials and Methods
It was used a dental alloy widely commercialized with the following nominal composition (wt.%): Ni-62.01; Cr-32.65; Mo-1.02; Co-3.00; other-1.01. Prior to electrochemical measurements, the sample was mechanically polished using SiC polishing paper and finished with alumina suspension (1 µm). Thereafter, it was ultra-sonically rinsed in distilled water for 900 s. Subsequently the sample was electro-etched in 20% (wt.%) at 2.0 V for 3 s and examined using a Nikon Epiphot 200 optical microscope. For corrosion experiments, the alloy surface was polished and rinsed as previously described. In this new step, an electro-etching was not performed. Electrochemical measurements were achieved in a three electrodes cell containing an electrolyte which is similar to physiological serum (0.9% aqueous physiological solution) at different values of pH (2.0, 4.0, and 6.0). The counter electrode was a Pt foil and the reference electrode was an AgCl/Cl-. Solutions were prepared using an analytical grade reagent and were not deaerated. Experiments were carried out at room temperature (25o C).Electrochemical measurements were made with a PGSTAT302 potentiostat (Eco. Chemie B. V., Utrecht, Netherlands), that included corrosion potential, cyclic polarization (at 0.001 V s-1 from OCP until +1.0 V) and chronoamperometric curves (at +0.3 V for 450 s). Additionally, impedance measurements were carried out with a 10 mV rms perturbation in a frequency ranging from 100 kHz to 0.01 Hz, ten points per frequency decade, fitted by FRA software (Frequency Response Analyser, Eco. Chemie B. V., Utrecht, Netherlands) version 4.9. A minimum of two replicates was carried out for each type of test.
3. Results
Figure 1 shows the microstructure of the investigated dental Ni-based alloy after its electro-etching. It reveals a microstructure characterized by porous of different sizes and shapes randomly distributed in a typical dendritic arrangement (light grey) in a solid solution matrix (dark grey).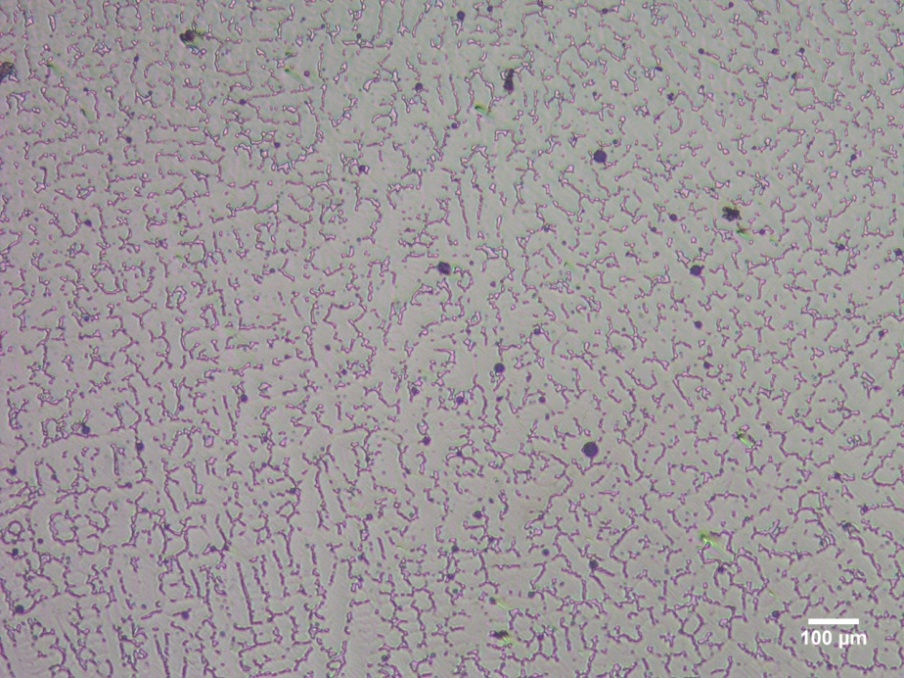 | Figure 1. Optical micrograph of Ni-Cr-Mo alloy |
In Figure 2, replicates of the OCP curves are presented. They were obtained for the dental alloy in 0.9% NaCl at different pH values. The open circuit potential assessment enables establishing a comparison of the nobility of the material in different media, so that, the higher potential value indicates a higher stability in the assessed medium. According to this figure, a typical behavior of passive state was observed at pH 4.0 and 6.0 through the ascending potential with time and the formation of a protective film, which is probably an oxide with a certain degree of hydration. In addition, the OCP values in the apparently steady state became smaller with a decrease in pH for all obtained replicates. It is assumed, hence, that the corrosive wear by the chloride ions is increased with a decrease in the pH of the medium, which may lead to partial dissolution of the passivation film at pH 2.0.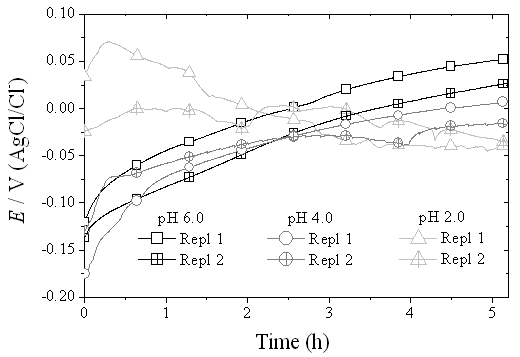 | Figure 2. OCP curves of the Ni-Cr-Mo alloy in 0.9% NaCl medium at different values of pH |
The CP curves presented in Figure 3 were obtained by polarizing the electrode at approximately +1.0 V from OCP in its apparently steady state. The cyclic polarization incorporates the same potential spectrum of the potentiodynamic scanning, moreover with the potentials of the reverse one until it returns to its original value of OCP [13]. With this broad spectrum of potential, it is possible to get additional information on the stability of the passive film. The kinetic parameters used in this analysis include the primary passivation potential, Epp, where the current decreases or becomes essentially constant within a finite range of potential; the film disruption potential, Eb; where the current increases after the primary passivation; and the width of the passive region, which is determined by the difference between the Epp and the Eb; where these amounts are commonly used to assess how effectively a passive film protects a metal against corrosion. In this sense, it was found that the corresponding values of primary passivation current, Ipp, were significantly lower at pH 6.0, when compared to those obtained at pH 4.0 or 2.0, as shown in Table 1. 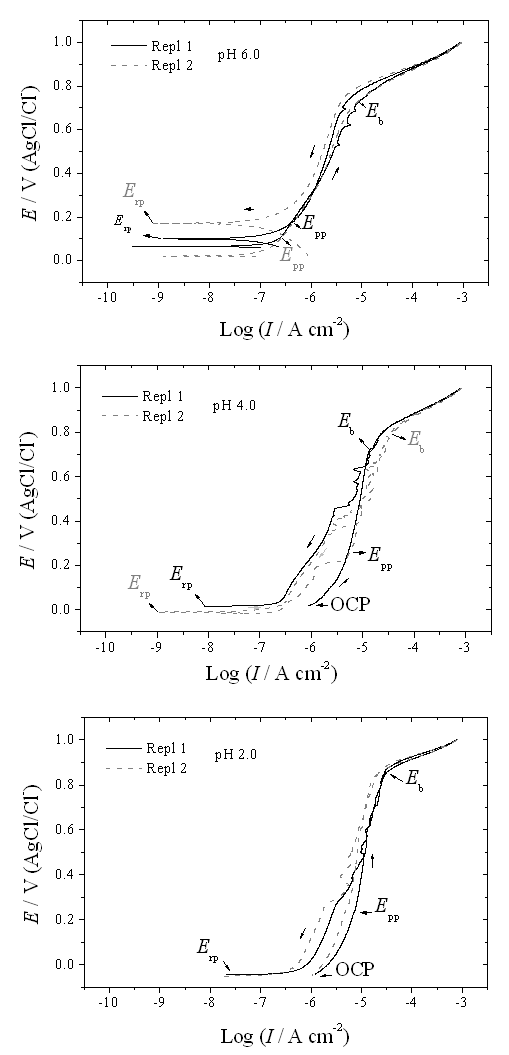 | Figure 3. CP curves of the Ni-Cr-Mo alloy in 0.9% NaCl medium at different values of pH |
Table 1. Kinetic parameters calculated from the OCP and CP curves obtained for the Ni-Cr-Mo alloy in 0.9% NaCl at different pH values 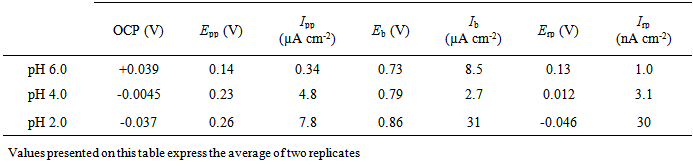 |
| |
|
The values of OCP in this table represent the average of the final 10 points, and it confirms the descending order of the potential, which can be seen in the graph of Figure 2, with a decrease in the pH. Although the Epp increases with a decrease in the pH, the Eb also increases, and thus the difference between the Eb and the Epp is not significant when compared to different experimental conditions. Furthermore, although the Eb can be clearly identified in the presented CP curves, the Epp can only be estimated by its shown pseudo-passive features that are identified by a gradual and continuous increase in the current, i.e. the current is not entirely stationary during the passive range of these curves. With this result, comparing the assessed conditions, it is verified that the differences are not noteworthy just with direct scanning records. On the other hand, the negative hysteresis, which can be observed more clearly in the replicates recorded at pH 6.0, is characteristic of a passive behavior and it occurs when the current density values of the reverse scanning are lower than those recorded in the direct scanning, which indicates, hence, how readily a passive film can regenerate itself after its transpassive limit. It was found that the hysteresis tends to become narrower and narrower with a decrease in the pH, thus denoting a greater difficulty for the passivation film to regenerate at pH 2.0. In addition, constant oscillations were observed in current density values, within a potential range between 0.4 and 0.8 V during the direct scanning. This probably indicates the lack of film stability in chloride medium, which becomes more pronounced with decreased pH. This behavior can also be interpreted by a change in the physicochemical properties of the passive film, as the potential becomes greater and greater.Another quantity used to assess the corrosive process and indicate whether the localized corrosion occurs is the potential of re-passivation, Erp. It is believed that pitting corrosion occurs when the OCP is greater than the Erp, but it does not occur when the OCP is less than or equal to the Erp [13]. In this work, the Erp was significantly greater than the OCP at pH 6.0, and slightly greater than the OCP at pH 4.0 while that, at pH 2.0, the Erp and the OCP were very similar, indicating that the corrosive wear is increased with a decrease in the pH. In other words, the difference between Erp and OCP becomes increasingly smaller with a decrease in the pH value. Although the Irp values are all in the same order of magnitude, they point out to stress this observed difference (the Irp at pH 2.0 is 10 times greater than at pH 4.0 and 30 times greater than at pH 6.0, approximately, as the results in Table 1).In the chronoamperometric curves of Figure 4, it can be observed the expected decay of the current density values recorded at +0.3 V (AgCl/Cl-), due to a decrease in the active area by the passivation film growth. This potential value is inserted within the passive region of the CP curves of Figure 3. The current densities, observed at the end of the growing period of the film, decrease in the following order: IpH2.0 > IpH4.0 > IpH6.0, which is consistent with the anodic profiles analysis of Figure 3. The rapid passivation was observed at higher values of pH (4.0 and 6.0) and, in the solution at pH 2.0, the current does not seem to reach the steady state. It is also important to highlight that it was not observed active/passive peaks that reveal the formation of new species during the film growth on this period.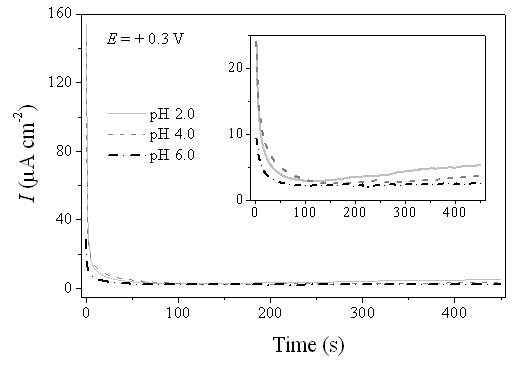 | Figure 4. Chronoamperometric curves recorded at +0.3 V (AgCl/Cl-) for the Ni-Cr-Mo alloy in 0.9% NaCl medium at different values of pH |
The EIS graphics measured in the open circuit potentials are presented in Figure 5. The complex plane graphics, Figure 5(a), display a slight decrease in the capacitive arc as the pH of the solution decreases. At intermediary and low frequencies, a straight segment was observed, which is typical of a diffusional process. This behavior suggests the occurrence of, at least, two processes in the corrosion mechanism. In the three pH values, the impedance module was in the order of 105 Ω cm2, which indicates good corrosion resistance, Figure 5(b). 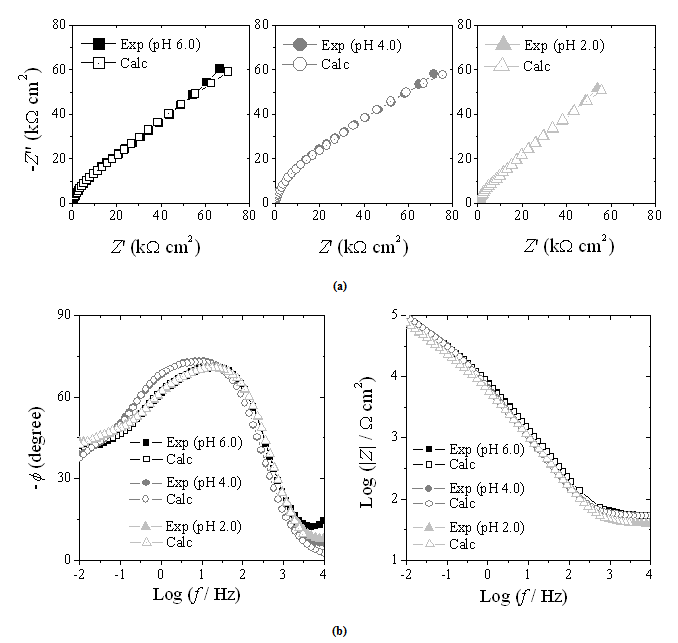 | Figure 5. EIS spectra obtained for the Ni-Cr-Mo alloy in 0.9% NaCl medium at different values of pH: (a) complex plane format; (b) Bode format |
In the same figure, the phase angle variation with the frequency showed in all cases broad peaks with maximums that are much smaller than 90º, denoting the lack of an ideally capacitive response. This behavior is explained in literature by the formation of a passive film with discontinuities of morphological order, and micro-structural defects on the surface of the Ni-Cr-Mo alloy [6]. It was also observed that this maximum becomes narrower with a decrease in the pH, indicating an increasingly different response from an ideally capacitive behavior. For this reason, it is deduced that the formed film is more discontinuous at pH 2.0, due to the alternation between the passivation and dissolution processes, which is consistent with the oscillations observed in the direct scanning of the CP curve replicates (Figure 3).At least two time constants can be observed in the spectra, modeled by the use of equivalent electrical circuits which take into account the diffusion component insertion (Warburg impedance, W) for the settings of the spectra’s settings at low frequencies [14]. Different circuits were tested to set the spectra at different pH values, Figure 6.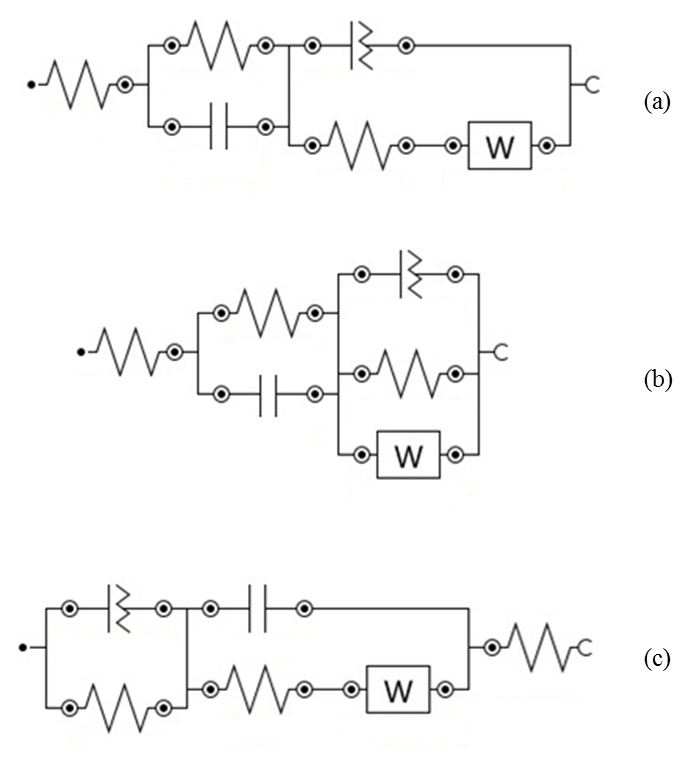 | Figure 6. Equivalent electrical circuit models used for the fitting of EIS spectra: (a) R1(R2C1)(Q1R3W); (b) R1(R2C1)(Q1[R3W]); (c) (Q1R1)(C1[R2W])R3 |
The results illustrated in Figure 5 spectra were obtained using the circuit of Figure 6(a). According to this model and comparing the three assessed conditions, it is verified that the term Q1 significantly increases with a decrease in the pH. This term represents a constant phase element (CPE) with n > 0.80, and it seems to indicate that the impedance responses could be better represented by a RC instead of a RQ combination. However, the fittings were better with the insertion of this element instead of a pure capacitance. It was observed that C1 values were significantly higher at pH 4.0 and 2.0 when compared to those obtained at pH 6.0. The other parameters did not significantly vary with a decrease in the pH, except for R2 which decreases with a decreasing pH. All these variations together may be associated to an increase in the passive film instability with pH decrease, as a consequence of a localized dissolution process making the film more porous. The equivalent electric circuit of Figure 6(b) is described in literature for the impedance response of a Ni-Cr-Mo alloy in NaCl 5.0 mol L-1 as an applied potential that corresponds to the passive range of the polarization curve in this medium [5]. Using this model, the authors consider the absence of elements representing the film/electrolyte interface, as the negligible load transfer resistance on this interface. In this case, the total system impedance presented the highest values (Table 2).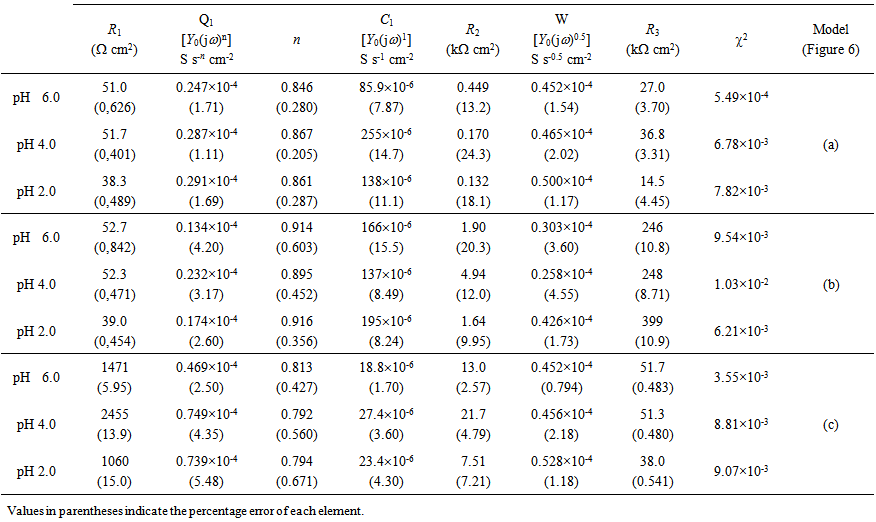 | Table 2. Fitting results of the EIS spectra obtained for the Ni-Cr-Mo alloy in 0.9% NaCl medium at different pH values |
The circuit model represented by Figure 6(c) is related in literature to the formation of a layer of more defective oxides, allowing the transfer of load on the film/electrolyte interface. The results of the adjustments made using this model were more similar to those in Figure 6(a).
4. Discussion
Although Ni-Cr-Mo alloys are widely disseminated in the dental market, very few publications are found in literature regarding corrosion studies in a chloride medium. The most recent works with OCP, CP, and EIS measures generally correlate different compositions of Ni-Cr-Mo alloys with corrosion resistance, so that works reporting the influences of variations in the composition, temperature, or pH of the saline medium do not have any precedents.Reclaru et al. [16] also point out to the lack of epidemiological studies on the correlation between Ni-Cr alloys in the mouth and allergies due to contact with Ni because its released quantity is greater than the limits imposed by the European Union, whereas these alloys were effectively banned in countries such as Sweden and Denmark because of it. Despite the imposed restrictions, the use of Ni-Cr alloys is increasing even more, which raises the need for more conclusive studies on its effect in the human organism for many years. One of the criteria assessed in these studies takes into account the association of Eb to a better behavior regarding corrosion resistance. In the comparison of different compositions of Ni-Cr-Mo alloys with those of noble metals, for example, the lowest values of Eb were recorded for the Ni-Cr-Mo, which means a greater possibility of corrosion with more Ni release [15].Basic metals alloys, used as biomaterials, are commonly covered with a thin layer of oxides, whose composition would vary according to alterations of the medium due to continuous processes of localized dissolution and passivation, even though the film is macroscopically stable. Nagai et al [17] characterized the surface covered with an oxide film of a Co-Ni-Cr-Mo alloy immersed in Hanks solution, and found oxides of all constituent metals, in addition to a large amount of OH-, especially in the outer layers of the film. The authors also found that the Cr is distributed more on the inner layer and the Ni on the outer surface of the oxides film.Zhang et al. [18] characterized the properties of the passive film on a Ni-Cr-Mo alloy. These authors obtained a double layer structure, one being inner, Cr3+ ion-enriched, and the other one external containing hydrated oxides formed by the hydrolysis of the released cations of the innermost layer. As the potential increases within the passive range during the electrode polarization, the film’s composition changes because the oxidative dissolution of the innermost layer takes place instead. Moreover, the oxidation of Cr3+ into Cr4+ on the innermost layer of the film during the transpassive range and its resulting dissolution leads to a loss of Cr2O3 on this layer, and to a destruction of the passivity.In the case of passive alloys, corrosion takes place due to variations in chemical composition or properties of oxide film when in contact with the corrosive medium. In the mouth, this process is continuous due to the abrasion of food and brushing [1]. Still with respect to the characterization of the film, no attempt to adjust EIS spectra was successful using models of simple equivalent circuit without Warburg element inclusion, referenced in this work to take into account the shortcomings of the film, which allows the transport of ionic species through the same via ionic vacancies or interstitial cations with effects on the diffusion component represented by a straight segment in the low frequency region of the spectra [5]. Based on the EIS cited studies and on the results of this study, it was found that the properties of the passive film on the Ni-Cr-Mo alloy were determinant in the corrosion resistance.
5. Conclusions
In the corrosion analysis of the Ni-Cr-Mo alloy in 0.9% NaCl, it was found a passive behavior under the assessed conditions. The passive film was interpreted in terms of a double layer structure, an inner one being more compact and outer one more porous. A diffusion process through the pores can be a way to release nickel ions release. With decreasing pH, a higher difficulty of the passive film to regenerate was observed, which can be due to changes in the morphology and structure of film in different media. The presented data clearly show that combined electrochemical techniques are very suitable for corrosion resistance assessment of dental alloys, through a good correlation between the results obtained from different methods of analysis.
ACKNOWLEDGEMENTS
Acknowledgements are to PROPe/UNESP and FUNDUNESP for financial support. The authors would like to acknowledge the English language review, realized by FDCT – Foundation for Scientific and Technological Development.
References
[1] | Lu Y, Chen W, Ke W, Wu S. Nickel-based (Ni-Cr and Ni-Cr-Be) alloys used in dental restorations may be a potential cause for immune-mediated hypersensitivity. Med Hypotheses 2009; 73: 716-717. |
[2] | Alves H, Heubner U. Aqueous corrosion of nickel and its alloys. Sheir’s Corrosion 2010; 3: 1879-1915. |
[3] | McGinley EL, Coleman DC, Moran GP, Fleming GJP. Effects of surface finishing on the biocompatibility of a nickel-chromium dental casting alloy. Dent Mater 2011; 27: 637-650. |
[4] | Liu J, Qiu XM, Sun DQ. Microstructures and mechanical properties of interface between porcelain and Ni-Cr alloy. Mater Sci Eng A Struct Mater 2008; 497: 421-425. |
[5] | Jakupi P, Zagidulin D, Noel JJ, Shoesmith DW. The impedance properties of the oxide film on the Ni-Cr-Mo alloy-22 in neutral concentrated sodium chloride solution. Electrochim Acta 2011; 56: 6251-6259. |
[6] | Jones SB, Taylor RL, Colligon JS, Johnson D. Effect of element concentration on nickel release from dental alloys using a novel ion beam method. Dent Mater 2010; 26: 249-256. |
[7] | Manaranche C, Hornberger H. A proposal for the classification of dental alloys according to their resistance to corrosion. Dent Mater 2007; 23: 1428-1437. |
[8] | Tuna SH, Pekmez NO, Keyf F, Canli F. The influence of the pure metal components of four different casting alloys on the electrochemical properties of the alloys. Dent Mater 2009; 25: 1096-1103. |
[9] | Saji VS, Choe H-C. Electrochemical behavior of Co-Cr and Ni-Cr dental cast alloys. Trans Nonferrous Metals Society of China 2009; 19: 785-790. |
[10] | Lin H-Y, Bowers B, Wolan JT, Cai Z, Bumgardner JD. Metallurgical, surface, and corrosion analysis of Ni-Cr dental casting alloys before and after porcelain firing. Dent Mater 2008; 24: 378-385. |
[11] | Wylie CM, Shelton RM, Fleming GJP, Davenport AJ. Corrosion of nickel-based dental casting alloys. Dent Mater 2007; 23: 655-776. |
[12] | Ciszewski A, Baraniak M, Urbanek-Brychczynska M. Corrosion by galvanic coupling between amalgam and different chromium-based alloys. Dent Mater, 2007; 23: 1256-1261. |
[13] | Tait WS. An introduction to electrochemical corrosion testing for practicing engineers and scientists. PairODocs Publications, 1994. |
[14] | Boukamp, B. A. Equivalent circuit software. University of Twente, Netherlands; 1989. |
[15] | Sharma M, Ramesh Kumar AV, Singh N. Electrochemical corrosion behavior of dental/implant alloys in saline medium. J Mater Sci Mater Med 2008; 19: 2647-2653. |
[16] | Reclaru L, Unger RE, Kirkpatrick CJ, Susz C, Eschler P–Y, Zuercher M–H, Antoniac I, Luthy H. Ni-Cr based dental alloys; Ni release, corrosion and biological evaluation. Mater Sci Eng C Mater Biol 2012; 32: 1452-1460. |
[17] | Nagai A, Tsutsumi Y, Suzuki Y, Katayama K, Hanawa T, Yamashita K. Characterization of air-formed surface oxide film on a Co-Ni-Cr-Mo alloy (MP35N) and its change in Hanks’ solution. Appl Surf Sci 2012; 258: 5490-5498. |
[18] | Zhang X, Zagidulin D, Shoesmith DW. Characterization of film properties on the Ni-Cr-Mo Alloy C-2000. Electrochim Acta 2013; 89: 814-822. |