Omar A. Al-Hartomy1, 2, Ahmed A. Al-Ghamdi1, Said A. Farha Al Said1, 2, Nikolay Dishovsky3, Michael B. Ward4, Petrunka Malinova3, Lako Lakov5, Radostin Nikolov3
1Department of Physics, Faculty of Science, King Abdulaziz University, Jeddah 21589, Saudi Arabia
2Department of Physics, Faculty of Science, University of Tabuk, Tabuk 71491, Saudi Arabia
3Department of Polymer Engineering, University of Chemical Technology and Metallurgy, 8 Kl. Ohridski blvd., 1756 Sofia, Bulgaria
4LEMAS, Institute for Materials Research, SPEME, University of Leeds, LS2 9JT, UK
5Institute of General and Inorganic Chemistry, Bulgarian Academy of Sciences, 1113 Sofia
Correspondence to: Nikolay Dishovsky, Department of Polymer Engineering, University of Chemical Technology and Metallurgy, 8 Kl. Ohridski blvd., 1756 Sofia, Bulgaria.
Email: |  |
Copyright © 2014 Scientific & Academic Publishing. All Rights Reserved.
Abstract
In this study dual phase fillers obtained by impregnation method have been characterized by energy dispersive X-ray (EDX) spectroscopy in a scanning transmission electron microscope (STEM) or STEM-EDX. The difference between these fillers and a simple physical mixture of carbon black and silica, taken in the same proportions as in the resulting through impregnation fillers was investigated. The impregnation method used to obtain the dual phase filler allows tailoring the characteristics of the filler by altering the amount of silicon dioxide introduced. The results achieved showed that the conditions of obtaining influence the distribution and the location of the phases in the carbon silica hybrid fillers as well as their most essential characteristics including specific area, oil absorption number, iodine adsorption number, ash content and others.
Keywords:
Carbon Silica Hybrid Fillers, Impregnation, Filler Characterization, STEM-EDX Method
Cite this paper: Omar A. Al-Hartomy, Ahmed A. Al-Ghamdi, Said A. Farha Al Said, Nikolay Dishovsky, Michael B. Ward, Petrunka Malinova, Lako Lakov, Radostin Nikolov, STEM-EDX Characterization of Carbon-Silica Dual Phase Fillers Obtained via Impregnation Technology, International Journal of Materials and Chemistry, Vol. 4 No. 3, 2014, pp. 41-50. doi: 10.5923/j.ijmc.20140403.01.
1. Introduction
The crucial role that the type of the filler has in improving the exploitation properties of rubber articles is well known [1]. In the recent years aiming to improve the trade off between wear resistance and rolling resistance in tires the company Cabot Corporation has developed new types of fillers. Those include carbon black modified chemically with different functional groups and carbon-metal oxide-multiphase composites. The company has also launched at the marked a dual phase filler of the carbon-silica type (CSDPF 2000) manufactured by a co-fuming process [2-4]. With this material, the filler-filler interaction is substantially reduced due to the surface modification, and the polymer-filler interaction is enhanced by increasing the surface energy of the carbon domain of the filler thus creating chemical bonds over the silica domain via a coupling reaction between polymer chains and silanols. The effects of filler-filler and filler-elastomer interactions on rubber reinforcement are also well known [5]. The pyrolysis cum water vapour of worn out waste tires used obtaining a carbon-silica type of fillers is a method alternative to the co-fuming process, and in the same time it is useful for rubber recycling [6-8]. The paper reports on the characteristics of the obtained fillers and on the effect they produce upon elastomer composites based on various compounds, including those based on natural rubber, epoxidized natural rubber, ethylene-propylene triple copolymer, etc. [9-13]. Impregnation is one of the most widely used and popular methods for loading different phases on carriers (first of all in the production of catalysts) or for surface and texture modification of adsorbents [14]. The second phase is deposited from the solution of a so called “precursor” (most often salts or soluble complexes) by impregnation. The impregnated substances are subjected to chemical or physical influence that may be considered as thermal activation. The method of impregnation was chosen because it allows controlled disposition of the second phase (silica) on the carbon black surface. A part of it may be also retained within the pores of the carbon black particles and in the space between the particles. Generally this technique results into a better dispersion and more homogeneous particle size distribution [14]. In our previous investigations we obtained hybrid filler for rubber by deposition of a zinc oxide phase on active carbon surface using the impregnation method [15]. In another paper [16] we reported on the possibilities for applying the impregnation method for obtaining a new type of reinforcing fillers for rubbers by introduction and deposition of silica phase on the particles of low active furnace carbon black. The small specific surface and characteristics of the filler thus obtained were investigated. Its influence on the properties of styrene butadiene rubber (SBR) based composites (applicable for passenger tread compounds) was studied. It was found that the fillers mentioned above increase considerably the modulus at 100% of elongation and tensile strength in comparison to standard composites containing carbon black. The most important matters of our interest have been to establish: (i) the distribution of the phases of carbon and silica in the fillers obtained; (ii) extent they penetrate one another; (iii) how (and if) amount of silica influences this distribution; (iv) the difference between these fillers and a simple physical mixture of carbon black and silica, taken in the same proportions as in the fillers resulting from impregnation. Finding answers to these questions is the purpose of the present investigation. Doubtless, energy dispersive X-ray (EDX) spectroscopy using a scanning transmission electron microscope (STEM or STEM-EDX) is the most appropriate research approach for the purpose. The high angle annular dark field (HAADF) imaging in STEM displays the compositional contrast resulting from elements with different atomic number and their distribution. EDX allows semi-quantitave elemental analysis. Initial EDX analysis usually involves the generation of a characteristic X-ray spectrum from the specific area (spot size, line profile) entire scan area of the STEM image. When electron beam strike the material in TEM column then a characteristic X-ray radiation appears. Usually the Y-axis shows the counts (cps-counts per second) (number of photons received and processed by the detector) and the X-axis shows the energy of those X-rays. In addition EDX software can: - keep the electron beam stationary on a spot or on a series of spots and generate spectra that will provide more localized elemental information. - have the electron beam follow a line drawn on the sample image and generate a plot of the relative proportions of different elements along that line (one dimensional line scanning). - map the distribution and relative proportion (intensity) of different elements over the scanned area (two dimensional mapping). Modern software now collects entire cumulative spectra from each point, so element choices can be made post acquisition.
2. Experimental
2.1. Carbon-silica Fillers Preparation
Three types of carbon-silica fillers (marked as CSF-5, CSF-10 and CSF-20, respectively) were obtained by the impregnation procedure as follows:Industrial furnace carbon black type PM-15 (produced in Russia with characteristics close to those of carbon black ASTM type N 776) with specific area of 15 m2/g were used as a substratum in our investigation. Silicasol (containing 40% of SiO2; pH – 9; density 1. 3g/cm3) was chosen as an impregnating agent due to our idea to obtain reinforcing carbon-silica dual phase filler. This particular type of carbon black was chosen since: a) its specific surface is very low, so we wanted to find out whether it will increase upon the introduction of a second phase; b) the particles of this carbon black are very large in size (over 150 nm) and are distinguished perfectly from the much smaller particles of SiO2. That allows detecting precisely the distribution of SiO2 particles in the dual phase filler. The carbon black was modified with SiO2 via impregnation according to the thin layer chromatography method using a sprayer and constant stirring. The amount of SiO2 solution necessary for wetting the carbon black was 1.6 ml/g carbon black. The amount of silicasol was calculated in advance in order to introduce the right percentage of SiO2. The impregnation procedure was carried out at two stages. At the first stage of the synthesis a 1/10 of the calculated solution was diluted with distilled water at a 1:10 ratio. Under constant stirring this solution was sprayed over the carbon black and the sample was let stay in air at room temperature for 24 h. Then it was thermally treated at 323 - 353оK for 2 h and at 523оK for 2 h. At the second stage the rest of the solution was sprayed in an analogous manner under the same conditions. Having treated the carbon black with the solution it was again let stay in air at room temperature for 24 h. Then it was thermally treated at 323 - 353оK for 2 h, at 425оK for 4 h and at 523оK for 4 h. The dual phase fillers thus obtained comprised different amounts of SiO2, calculated theoretically as follows: CSF 5- 5 % SiO2, CSF10 - 10 % SiO2, CSF20 - 20 % SiO2. For comparison the physical mixture was prepared by mixing carbon black and silica at an 80:20 mass ratio on a ball mill for 2 h.
2.2. Methods Used to Characterize the Substratum and Carbon Silica Dual Phase Fillers
• Iodine adsorption – in correspondence to [17].• Oil absorption number – in correspondence to [18].• Specific surface area – calculated according to BET method [19].• Mesopore volume – calculated by Gurvich rule [20-22].The average diameter of the mesopores and their size distribution were determined by the method of Barett - Joyner-Halenda (BJH) [23]. The ash content of the carbon silica fillers obtained by the impregnation technology was determined according to [24]. The ash from the fillers was studied by complete silicate analyses, weight analysis, atomic absorption spectroscopy – AAS (Perkin Elmer 5000) and by inductively coupled plasma-optical emission spectroscopy - ICP-OES (“Prodigy” High Dispersion ICP-OES, Teledyne Lemas Labs). To ascertain the distribution of carbon black and silica, the hybrid products and their physical mixture were also investigated by the method of STEM (STEM, FEI Tecnai F20, FEGTEM) operating at 200 kV and equipped with an EDX Analyzer (Oxford Instruments, 80 mm2X-max SDD, running INCA software). One gram powder of each sample was used for the analyses. A small amount of it was mixed with solvent and then dropped with a pipette onto a carbon support film. The ratio of the Si to C counts was used as an estimate of the relative ranking of the amount of silica and carbon in certain regions in the filler aggregates.
3. Results and Discussion
Table 1 summarizes the main properties of the fillers obtained. Table 1. Main Properties of the Carbon-Silica Fillers Used 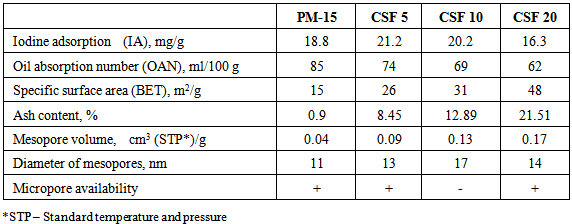 |
| |
|
The data in Table 1 show that at first, at 5% and 10% of SiO2, iodine adsorption increases, if compared to that of the initial carbon black. Then it starts to decrease with increasing amount of SiO2 in the dual filler and finally drops under its starting level. That indicates an improvement in the adsorption activity of the dual filler with regard to rubber macromolecules which results from the introduction of SiO2 (up to 10%) [25]. Contrary to the expectations that the effect observed might be also due to iodine penetration into carbon black pores, it is seen that the sample with largest pore volume has the lowest iodine adsorption value. Meanwhile the decrease of oil absorption number at higher SiO2 amounts reveals the worsened interaction between the filler particles and their proneness to form secondary structures. Noteworthy, the increase in SiO2 amount causes a considerable increase (several times) in the specific surface area of the filler particles while the increase in the mesopores volume and diameter is lesser. The filler comprising 10% of SiO2 has the largest mesopore diameter, i.e. the dependence of this parameter on SiO2 amount is not linear. The ash content increases regularly (8.45%, 12.89% and 21.51%) what is due mainly to the higher SiO2 amount in the filler. As seen from Table 2, SiO2 amount in the ash calculated experimentally is dominating and varies within the 93-96% range. Recalculated regarding the entire filler the ash content is 5.9%, 10.7% and 20.4%, respectively, which is close to the theoretical one (5%, 10% and 20%). With the increasing SiO2 amount the overlap between the experimental and theoretical values is greater. Hence, the suggested impregnation method for obtaining dual fillers can be altered and the content of the second phase is controllable what allows tailoring the other properties of the filler (specific surface area, mesopore volume and diameter, etc.). Representative HAADF images and compositional maps of the carbon silica fillers obtained by the impregnation method are shown in Figures 1-4. The bright field TEM images of the same samples are shown in Figures 5-8. Table 2. Silicate Analysis, AAS and ICP-OES (in %) of the Carbon-Silica Fillers Ashes |
| |
|
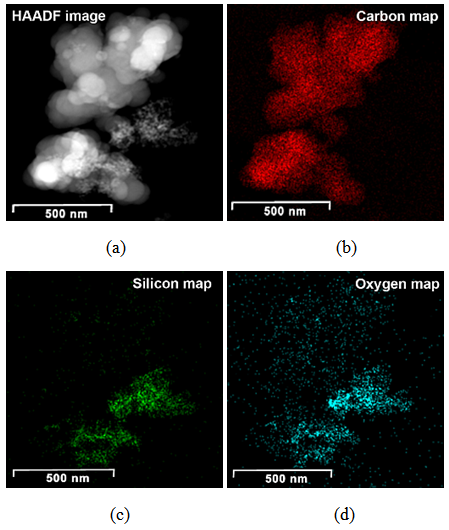 | Figure 1. HAADF image (a) and compositional maps of CSF-5 (b, c, d) |
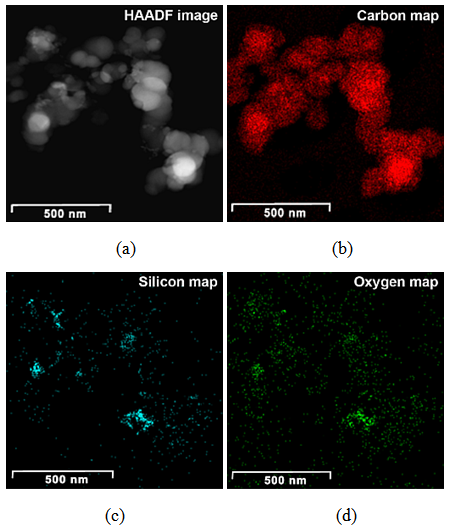 | Figure 2. HAADF image (a) and compositional maps of CSF-10 (b, c, d) |
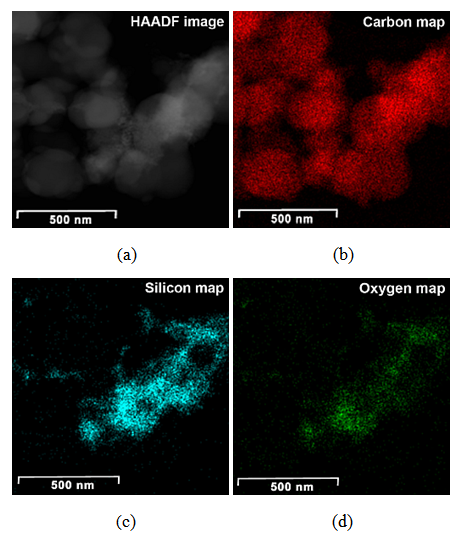 | Figure 3. HAADF image (a) and compositional maps of CSF-20 (b, c, d) |
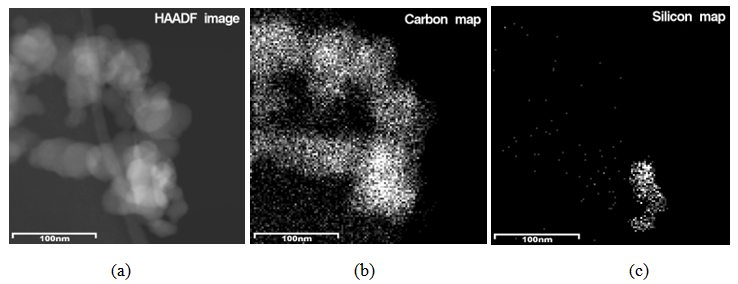 | Figure 4. HAADF image (a) and compositional maps of a physical mixture of carbon black and silica (b, c) |
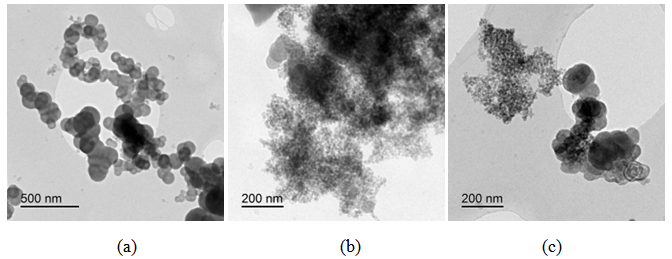 | Figure 5. Bright field TEM images of CSF-5 at different magnifications |
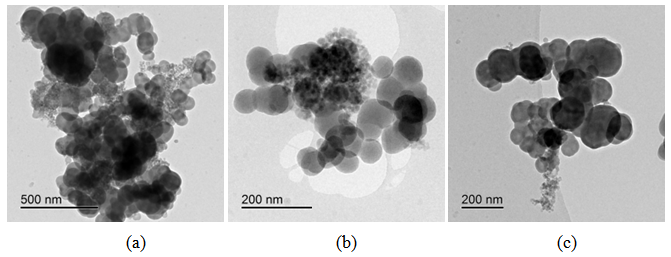 | Figure 6. Bright field TEM images of CSF-10 at different magnifications |
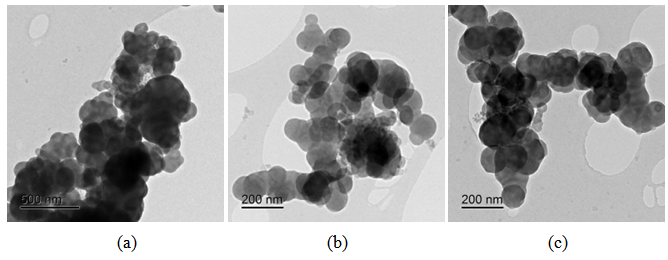 | Figure 7. Bright field TEM images of CSF-20 at different magnifications |
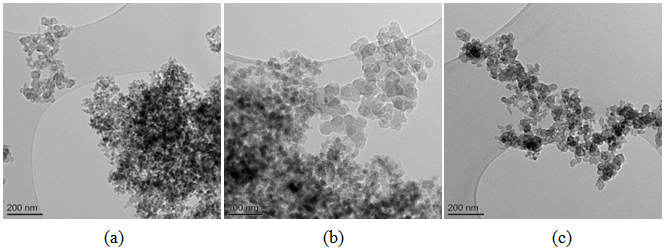 | Figure 8. Bright field TEM images of carbon black-silica mixtures prepared by ball mill at different magnifications |
Generally at first carbon and SiO2 phases in the samples prepared by the impregnation seem to be mixed much better than those in the physical mixture prepared on a ball mill. STEM-EDX examination reveals isolated silica and carbon black aggregates in the physical mixture of silica and carbon black, whereas for CSF the mixes on smaller length scales. A typical area from the physical mixture sample can be seen in Fig. 8b, with a carbon cluster on the right and SiO2 on the left. Regarding the samples obtained by impregnation, it is quite clear that both phases exist independently, i.e. there are discreet regions of amorphous SiO2 and of soot-like carbon. The only difference is to what extent and on what scale the particles are mixed. The EDX maps show distributions of the different particles. In sample CSF-5 silicon dioxide is almost entirely in the form of separate clusters which are in contact with those of carbon black. The two types of clusters exist independently and are well distinguishable on the micrographs – those of carbon black are much larger while those SiO2 are smaller. The structure of carbon black particles is graphite-like (its carbon layers are well seen – Fig. 9), strings of carbon black particles are also observed (Fig. 5a). Silicon dioxide is amorphous. Although its particles are located close to each other they are not mixed. Neither type of particles dominates in the sample CSF-10. The cluster observed have various positions. Silica clusters are located both outside and inside the carbon black clusters but it seems that more of them are outside, especially over the surface of carbon black clusters. There are almost no independent silicon dioxide clusters observed in the sample CSF-10 wherein the particles of the latter have penetrated into the carbon black clusters and the bonds between carbon and silica particles are stronger. In most cases silica particles (as clusters) are inside the carbon black clusters, i.e. they are occluded, but some silica clusters are out of the carbon black clusters and act as a coating. It seems that silica clustering is seen more often than silica coating of the carbon black clusters. As the images show, the most successful sample is CSF-10. Its phases seem to be the best mixed ones. At a lower SiO2 concentration (CSF-5) the two phases exist rather independently and are isolated. At a higher SiO2 concentration (CSF-20) most silicon dioxide particles are located inside the carbon black particle being isolated from the contact with elastomer macromolecules.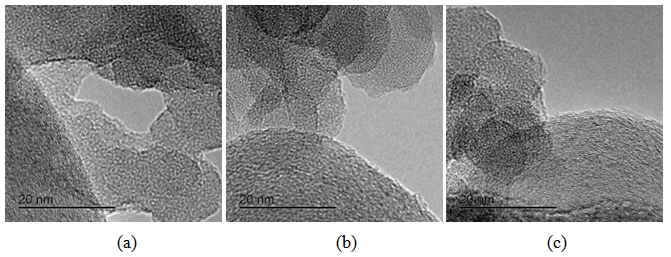 | Figure 9. Bright field TEM images of carbon black particles, showing their structure; (a) sample containing 5% SiO2; (b) sample containing 10% SiO2; (c) sample containing 20% SiO2 |
Carbon and silica clusters are at the same location all over CSF samples. In the physical mixture of carbon black and silica prepared on a ball mill SiO2 exists in isolated aggregates which are not mixed with the carbon aggregates. Clumps of SiO2 can be seen on the SiO2 map. SiO2 particles coalesce with each other but not with carbon. It is difficult to find areas in which carbon and SiO2 are co-localized. Bright field TEM images confirm the said above. The clusters are separated approximately by 15 nm. Bright particles are carbon, dark particles are SiO2.Energy dispersive spectra on STEM of carbon black-silica filler obtained by impregnation are shown in Fig. 10-12.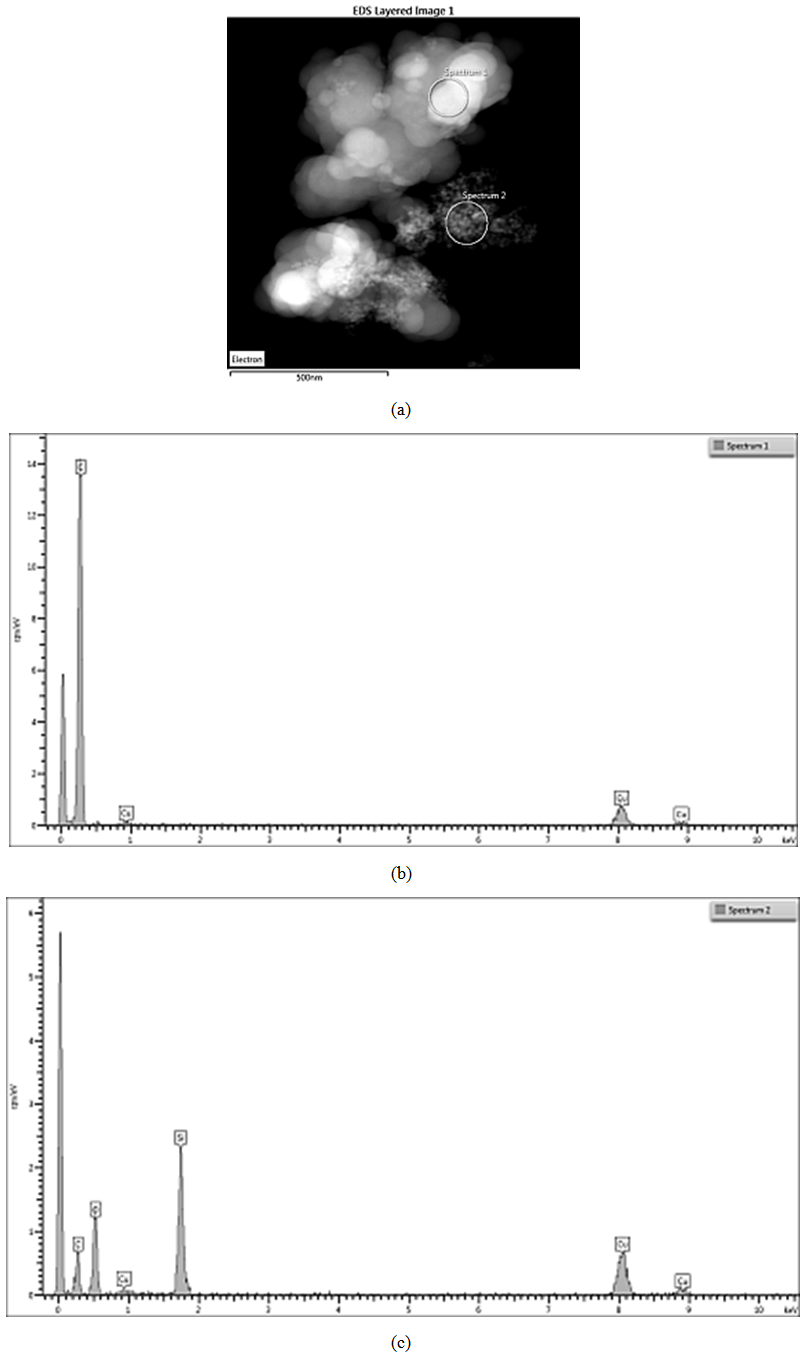 | Figure 10. EDS on STEM of CSF 5 filler: (a) spectra positions; (b) spectrum 1; (c) spectrum 2 |
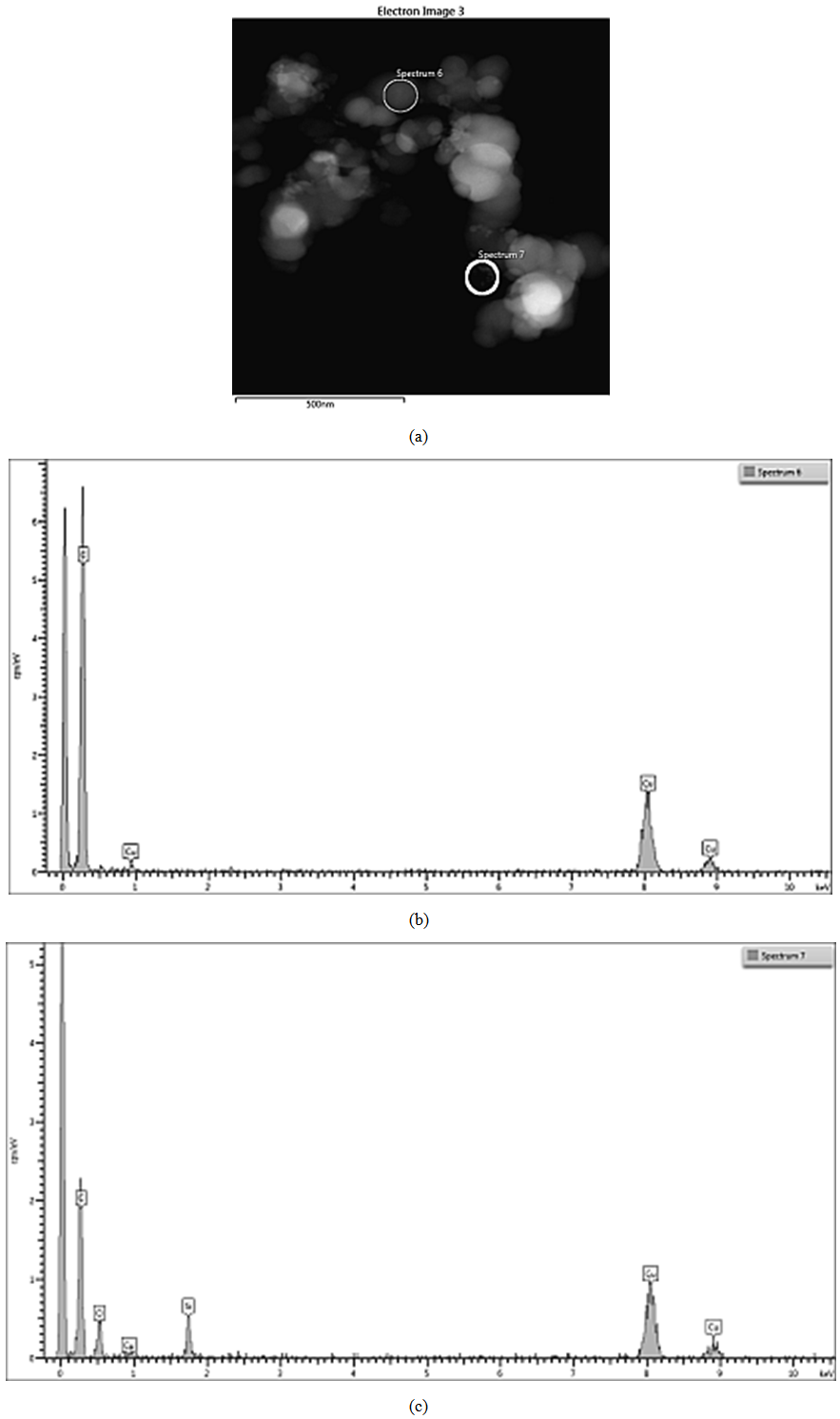 | Figure 11. EDS on STEM of CSF 10 filler: (a) spectra positions; (b) spectrum 6; (c) spectrum 7 |
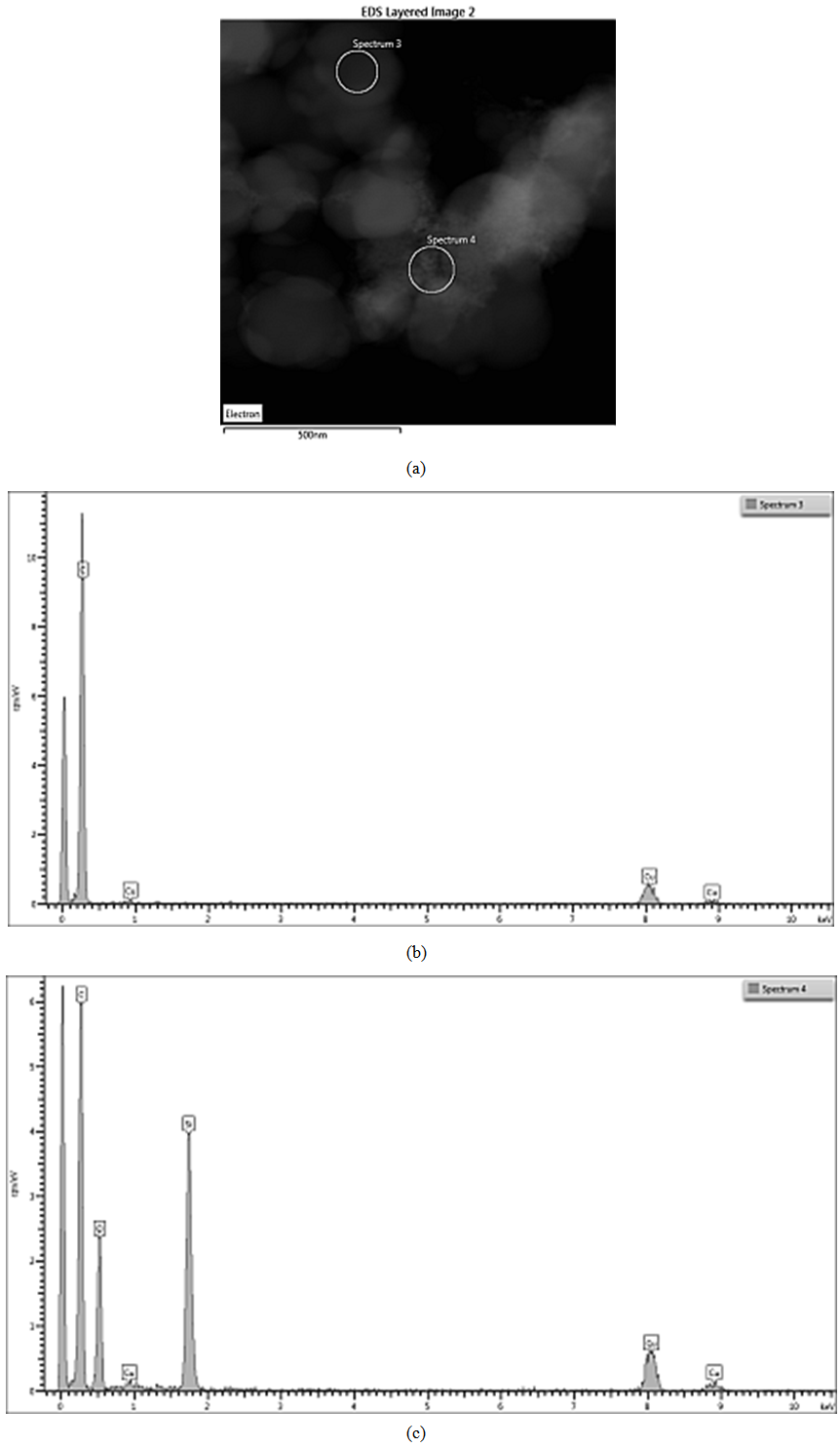 | Figure 12. EDS on STEM of CSF 20 filler: (a) spectra positions; (b) spectrum 3; (c) spectrum 4 |
ЕDX show the existence of domains containing entirely carbon (spectra 1, 3, 6) as well as domains wherein the carbon and silicon dioxide clusters co-exist. The observed trend is that the increasing SiO2 amount in the sample leads to the increase in its amount in those particular domains what is detected by the higher intensity of the peaks assigned to silicon and oxygen (spectra 2, 4 and 7). The physical mixing does not produce compounding at a deeper level, hence the spectra have peaks either for carbon or for silicon dioxide. It is also obvious that the amount of silicon dioxide influences practically all the characteristics of the hybrid filler, as seen from Tables 1 and 2. Studies by STEM-EDX also show the filler influence on the formation of silica aggregates, their size and placement amongst the formations of carbon black phase. From that point of view, we conclude that the silica concentration for obtaining of CSF-10 is more favorable because the aggregates of both phases are comparable and not well isolated. The ccharacteristics of CSF-10, shown in Tables 1 and 2 also give precedence to this sample: its specific surface area is not so high, whereas it has characteristic pores of large diameter what enables the contact between a great number of elastomer macromolecules and the filler, hence that produces the best reinforcement effect.
4. Conclusions
The impregnation method used to obtain dual phase fillers of the type carbon-silicon dioxide allows precise regulation and control over the qualities of silicon dioxide introduced.The percentage of silicon dioxide introduced in the form of silicasol determines its location and mode of dispersion along the filler. Silicasol structure may vary from separate clusters, through clusters located over the surface of carbon black aggregates, to clusters that have penetrated into the volume of carbon black particles and to occlusion of the latter. The impregnation method produces mixing of the two phases better than that achieved by their physical mixture. The impregnation method used to obtain the dual phase filler has a number of advantages, namely it allows tailoring the characteristics of the filler by altering the amount of silicon dioxide introduced. The quantities of silicon dioxide introduced significantly change the specific surface area of the fillers, their mesopores volume and diameter and to a lesser extent their iodine adsorption and oil absorption number. Based on the results it is worth performing similar analysis on other type of carbon black (N330, N220) that has a well pronounced reinforcement effect and whose particles size is commeasurable with that of silicon dioxide.
ACKNOWLEDGEMENTS
The present research is a result of an international collaboration program between University of Tabuk, Tabuk, Kingdom of Saudi Arabia and the University of Chemical Technology and Metallurgy, Sofia, Bulgaria. The authors gratefully acknowledge the financial support from the University of Tabuk.Authors wish also to thank Dr. Antonia Dimitrova, SPEME, University of Leeds, Leeds LS2 9JT, UK for her assistance in STEM-EDX analyses, as well as Prof. Ljutzkan Ljutzkanov, Institute of Chemical Engineering, Bulgarian Academy of Sciences, Sofia, Bulgaria, for the preparation of the carbon-silica fillers.
References
[1] | J.-B. Donnet, and E. Custodero, Reinforcement of Elastomers by Particulate Fillers, in: J. E. Mark, B. Erman, C. M. Roland Ed. The Science and Technology of Rubber, 4th ed., Elsevier Inc., 383-416, 2013. |
[2] | K. Mahmud, M.-J. Wang, and R.A. Francis, “Elastomeric Compound Incorporating Silicon-Treated Carbon Black” U.S. Patent 5 830 930, 1998. |
[3] | M. J. Wang, Y. Kutsovsky, P. Zhang, G. Mehos, L. J. Murphy, and K. Mahmud, “Using carbon-silica dual phase filler improve global compromise between rolling resistance, wear resistance and wet skid resistance for tires”, Kaut. Gummi Kunstst., 55, (1-2), 33-40, 2002. |
[4] | M. J. Wang, K. Mahmud, L.J. Murphy, and W.J. Patterson, “Carbon-silica dual phase filler-a new generation reinforcing agent for rubber”, Kaut. Gummi Kunstst., 51, (5), 348-360, 1998. |
[5] | J. Frohlich, W. Niedermeier, and H.-D. Luginsland, “The effect of filler-filler and filler-elastomer interaction on rubber reinforcement”, Composites: Part A, 36 449-460, 2005. |
[6] | D. Kolev, R. Ljutzkanova, and S. Abadjiev, “Method and installation for pyrolysis of tires”, Bulgarian Patent 65901 B1, 2005. |
[7] | D. Kolev, R. Ljutzkanova, and S. Abadjiev, “Method and installation for pyrolysis of tires”, European Patent EP 1879978 A1, 2008. |
[8] | A. Isayev, Recycling of Rubbers, in: The Science and Technology of Rubber, Ed. B. Erman, J. Mark, and C. Roland, 4th ed., Elsevier Inc., 753-755, 2013. |
[9] | M. Ivanov, M. Mihaylov, and L. Ljutzkanov, “Silica obtained via pyrolysis of waste "green" tyres - a perspective filler for rubber industry” Kaut. Gummi Kunstst., 63, (7-8), 303-307, 2010. |
[10] | M. Ivanov, and M. Mihaylov, “Silica obtained via pyrolysis of waste “green” tyres - a Filler for tyre tread rubber blends”, J. Elast. Plast. 43 (4), 303-316, 2011. |
[11] | O. A. Al-Hartomy, A. A. Al-Ghamdi, S. A. Farha Al Said, N. Dishovsky, M. Mihaylov, M. Ivanov, and L. Ljutzkanov, “Effect of the carbon-silica reinforcing filler obtained from the pyrolysis-cum-water vapour of waste green tyres upon the properties of natural rubber based composites”, Progress in Rubber, Plast. Recycling Tech., 2013 (submitted). |
[12] | O. A. Al-Hartomy, A. A. Al-Ghamdi, S. A Farha Al Said, N. Dishovsky, M. Mihaylov, M. Ivanov, and L. Ljutzkanov, “Effect of the solid product yielded from pyrolysis-cum-water vapor of waste green tires upon the properties composites based on epoxidated natural rubber”, J. Elast. Plast., 2013 (submitted). |
[13] | O. A. Al-Hartomy, A. A. Al-Ghamdi, S. A. Farha Al Said, N. Dishovsky, M. Mihaylov, M. Ivanov, and L. Ljutzkanov, “Influence of the carbon-silica reinforcing filler obtained via pyrolysis of waste “green” tyres on the properties of EPDM based composites”, Kaut. Gummi Kunstst., 2013 (submitted). |
[14] | H. Marsh, E. Heintz, and F. Rodrigues-Reinoso, Ed. Introduction to Carbon Technologies University of Alicante, 1997. |
[15] | P. Malinova, R. Nikolov, N. Dishovsky, and L. Lakov, “Modification of carbon-containing fillers for elastomers”, Kaut. Gummi Kunstst., 57 443-445, 2004. |
[16] | O. A. Al-Hartomy, A. A. Al-Ghamdi, S. A. Farha Al Said, N. Dishovsky, P. Malinova, and R. Nikolov, “Obtaining of carbon-silica dual phase filler by impregnation method and investigation of its influence on the properties of SBR based composites”, J. Mat. Design and Appl. 2013 (submitted). |
[17] | ISO 15651/1-91 |
[18] | Bulgarian State Standard 9665:1176. |
[19] | S. Brunauer, P. H. Emmett and E. Teller, “Adsorption of Gases in Multimolecular Layers”, J. Am. Chem. Soc., 1938, (1938) J. Am. Chem. Soc., 60, 309-319. |
[20] | L.J. Gurvich, “Physico-chemical attractive force”, Zh. Russ. Fiz-Khim. Obshchestva Chem., 47, 805- 827, 1915. |
[21] | F. Rouquerol, J. Rouquerol, and K.S. W. Sing, Adsorption by Powders and Porous Solids, Academic Press, London, UK, 1999. |
[22] | S. Lowell, J. Shields, M. A. Thomas, and M. Thommes, Characterization of Porous Solids and Powders: Surface Area, Pore Size and Density, Springer, Netherlands, 2004. |
[23] | E.P. Barrett, L.G. Joyner, and P.P. Halenda, "The determination of pore volume and area distributions in porous substances. I. Computations form nitrogen isotherms". J. Am. Chem. Soc. 73 (1) 373–380, 1951. |
[24] | ISO 1125:1999 |
[25] | J. S. Dick, Ed. Rubber Technology Hanser Publishers, Munich, 2005. |