Abdul Al Mortuza 1, Md. Hafizur Rahman 2, Sinthia Shabnam Mou 3, Md. Julker Nain 3, Abu Bakar Md. Ismail 3
1Department of Biomedical Engineering, Gono University, Savar, Banglaesh
2Department of Physics, Pabna University of Science & Technology, Pabna, Banglaesh
3Department of Applied Physics and Electronic Engineering, Rajshahi University, Rajshahi 6205, Banglaesh
Correspondence to: Abu Bakar Md. Ismail , Department of Applied Physics and Electronic Engineering, Rajshahi University, Rajshahi 6205, Banglaesh.
Email: |  |
Copyright © 2012 Scientific & Academic Publishing. All Rights Reserved.
Abstract
Passivation of porous silicon (PS) by LaF3 by a novel chemical-bath technique has been investigated in this report. The porous silicon surface has been passivated with LaF3 by reacting LaCl3 with HF in the etching chamber at room temperature. Without removing the porous silicon from the anodizing chamber LaF3 as a result of chemical reaction between LaCl3 and HF was allowed to passivate the PS surface. Several cycles of reaction that gives the various thickness of LaF3 layer on PS have been investigated. The Scanning Electron Microscopy (SEM) on the surface of LaF3/PS/Si structure confirms the LaF3 film deposition on PS layer. The X-ray diffraction (XRD) of the deposited layer revealed a hexagonal nanocrystalline LaF3 deposition. The as-deposited thin (10-cycle) LaF3 layer contained very low concentration of lanthanum (La) and fluorine (F). The concentration of La and F started increasing with the increase in reaction cycle. But the photoluminescence (PL) of as deposited-LaF3 passivated PS was lower than that of fresh PS. Annealing the just passivated PS structure recovered the PL intensity. Experimental results show that the optimized chemical bath passivation process for the LaF3 on porous silicon could enable the porous silicon to be an important material for photonic application.
Keywords:
Porous Silicon, Passivation, Photoluminescence, Chemical Bath Deposition (CBD)
1. Introduction
Porous silicon (PS) has the potentiality to emit visible light and it is expected that this material can be effectively used to fabricate silicon-based visible light emitting devices and optical interconnections. A disadvantage of this material is the aging, that is, the slow spontaneous oxidation of PS[1]. A native oxide layer forms on the surface of the pores and the thickness of this oxide layer grows with time. Due to the aging effect, the structural and optical properties of PS show continuous change with storage time[1]. Passivation of PS is very important for the stabilization and enhancement of room-temperature photoluminescence (PL) of PS.[2]. The surface defects passivation can be achieved from various chemical adsorbates by several techniques[3-5]. In this work, an attempt has been made to grow an epitaxy of Lanthanum Fluoride (LaF3) on PS surface, as a passivation step, aiming to obtain stable and enhanced luminescence of PS layers. LaF3 is a large band-gap (about 10.3 eV)[6] material having a hexagonal crystal structure with a refractive index of1.61[7]. LaF3 thin films exhibit extremely good moisture resistance, therefore they are very useful, e.g., for protecting optical components[8]. As a passivating layer LaF3 has been grown on PS in various ways such as thermal evaporation and e-beam evaporation[9]. Thin films deposited by thermal evaporation are known to possess a columnar microstructure that strongly influences many of their properties[10]. But in this process just prepared PS needs to be transferred to the vacuum coating unit for deposition. Due to extremely high reactivity of the just prepared PS, during this time of drying and transferring the PS to the coating unit oxygen passivation occurs and that is believed to degrade the PL intensity [11, 12]. Moreover it is very difficult to passivate the silicon inside the pores by physical deposition technique. In the quest of finding a way of in-situ passition of LaF3 on the PS so that the PS needs not to be exposed to ambient air before passivation and pores are well passivated, we propose a novel technique quite similar to chemical bath deposition of LaF3. This technique is very simple and contains only one-step. In this CBD of LaF3 technique, LaCl3 is allowed to react with HF which produces the LaF3. When this reaction is done in the PS etching chamber the LaF3 crystallizes on the PS surface and passivates the surface dangling bond of the just prepared PS. This report investigates the structural and optical properties of the novel CBD-LaF3/PS system.
2. Experimental
Anodic etching was carried out on a (100) oriented phosphorous doped n-type c-Si of 1–2 Ω.cm resistivity using an electrolyte of HF (48%) and ethanol (98%) in 1:1 proportion under a constant current density of 15 mA/cm2 for 30 min at room temperature. The electrochemical anodization of Si wafer was done using a double tank cell set-up as shown in Fig-1. The wafer was cut into pieces and these pieces of Si wafer were cleaned by successively immersing in acetone, ethanol and deionized water. The electrolyte consisted of HF: C2H5OH in the ratio of 1:1 by volume. The etching was done at a current density of 15 mA/cm2. A 100W tungsten lamp was used for illumination from 15 cm distance. After 30 minute anodization, the etching solution and back contact solution was drained out keeping the samples in the etching chamber. The same double-tank chamber in the upright position is used for LaF3 deposition (Fig 2). Fresh HF was then introduced in the chamber to wash out any remaining etching solution on the chamber. After draining out the HF that used for washing, 0.2 M solution of LaCl3 and 48% HF were introduced simultaneously into the etching chamber through the “HF in” and “LaCl3 in” channels to do the chemical reaction.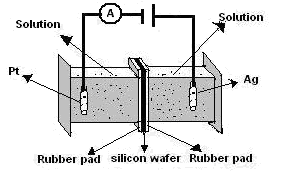 | Figure 1. Schematic drawing of a double-tank chamber for PS fabrication |
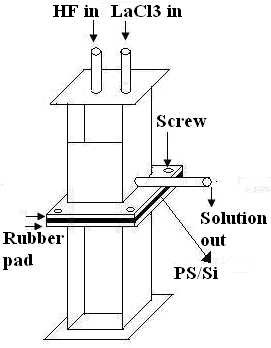 | Figure 2. Double-tank chamber in upright position for chemical bath deposition of LaF3 |
The chemical reaction that produces LaF3 is pretty simple, at room temperature, the addition of hydrofluoric acid to an aqueous solution of lanthanum chloride precipitates out lanthanum fluoride, LaF3[13]. The formation of white precipitate (LaF3) confirmed the mechanism of film formation.LaCl3 + 3HF → LaF3 + 3HClThe solution inside the etching chamber was stirred for 10 seconds and resulting LaF3 crystals were allowed to passivtate the PS layer for 4 min. After each cycle of reaction the solution was drained out through the “Solution out” channel and a new solution was introduced into the chamber for the next deposition cycle. In this case, the deposition results from a chemical reaction in solution, which may involve the surface silicon atoms, and in this case, we will speak of chemical grafting of the surface, and why the reaction is limited to the formation of one monolayer[14]. The whole process was repeated to obtain the various thickness of LaF3 on to PS. After completing the required cycle the wafer was removed from the chamber, rinsed with de-ionized water and dried in air at room temperature. In the first case,
3. Results and Discussion
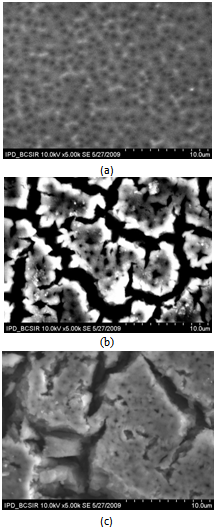 | Figure 3. SEM image of the top surface of (a) 10 (b) 20 and (c) 30 reaction cycles-grown LaF3/PS/Si structure |
It is interesting now to examine the growth of the LaF3 in terms of morphology. As shown in Fig 3 the surface morphology and chemical composition of LaF3 surface in the LaF3/PS/Si heterostructure were examined by SEM and EDX analysis. Surface of LaF3 for 10 deposition-cycles is shown in Fig. 3(a). Here the pores of the porous silicon are still visible indicating a very thin LaF3 layer on PS, which is also evident from the corresponding EDX spectra as shown in Fig 4. 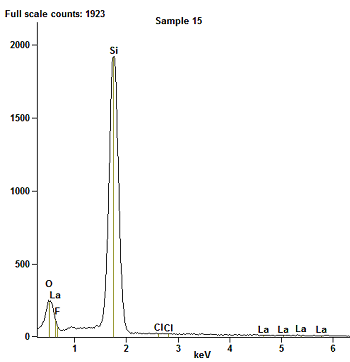 | Figure 4. EDX spectra of 10-reaction cycle LaF3 on PS |
The quantitative analysis shows a very low concentration of lanthanum and fluorine (0.06 at.%) compared with the silicon concentration (64.3 at.%) and oxygen (35.56 at.%).The SEM image and the corresponding EDX spectra of 20 and 30-cycle of LaF3 deposition has been shown in Fig 3(b) and (c). As the number of cycle of deposition increases, the LaF3 covers the whole pores and surface and the concentration of lanthanum and fluorine increases to 0.4 at.%and 2.84 at.% respectively. Fig 5 shows the growth of La and F with deposition cycle. 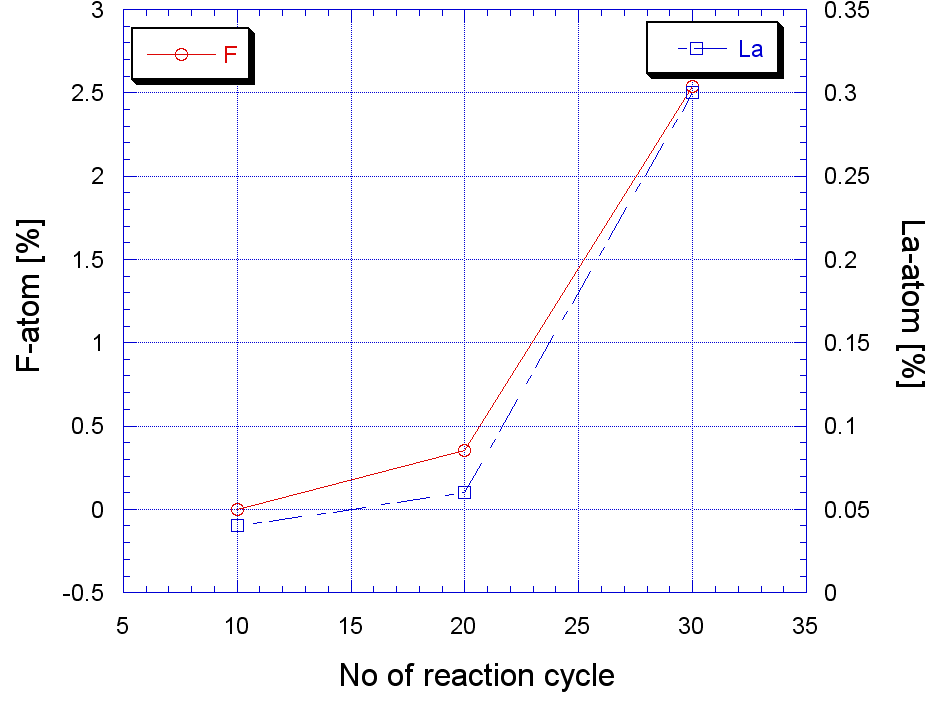 | Figure 5. Growth of La and F |
Since in the chemical reaction our source of F was only HF, even the 10-reaction cycle could not make appreciable passivation. The resulting LaF3 film was not stoichiometric. The fluorine to lanthanum ratio was 9 instead of 3. This is quite normal for the as-deposited films. The Fig. 6 tells us another story, as the reaction cycle increases the amount of Cl and also oxygen decreases. At the surface of 30-reaction cycle, there was no Cl but an appreciable amount of oxygen that might have incorporated during the crystallization process. Voids and large amounts of cracks were found on the thinner LaF3 surface (Fig 3(b)). For thicker surface the voids disappeared, but a high density of cracks can be noticed. 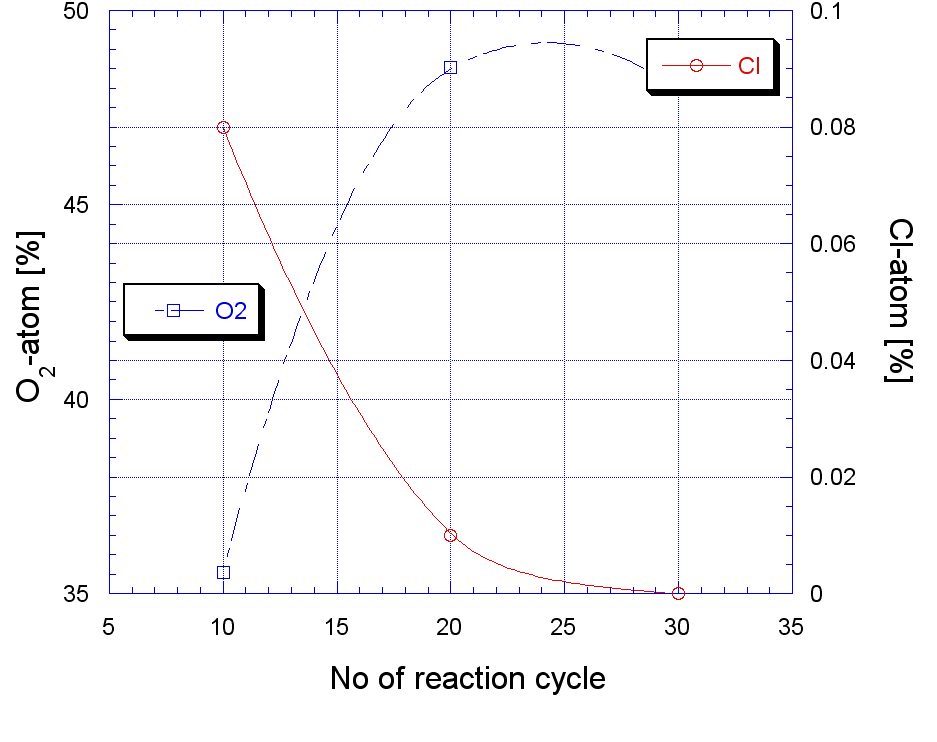 | Figure 6. Growth of oxygen and Cl with reaction cycle |
The structural analysis of LaF3 thin film was carried out using X-ray diffractometer varying diffraction angle 2θ from 20° to 60°. Fig. 7 shows the XRD pattern of LaF3 thin film deposited by CBD method onto the PS. The presence of a number of peaks in pattern is the indication of polycrystalline nature of the material. 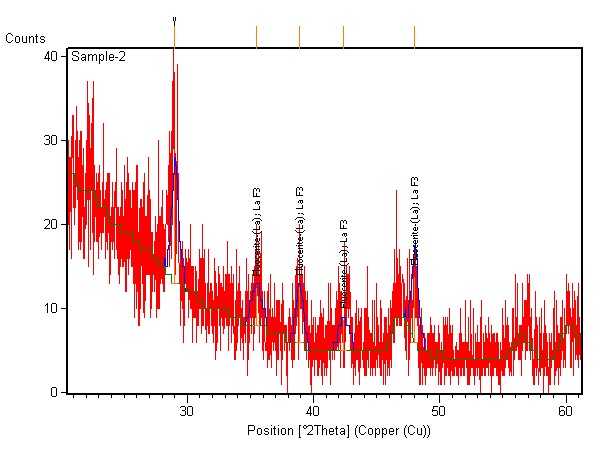 | Figure 7. XRD spectra of as-deposited LaF3 thinfilm |
The position and the relative intensity are identified from the ICSD file. The position of these peaks closely resembles of hexagonal (P-3c1) and polycrystalline structure with ref 01-082-0687. The comparison of the observed diffraction peak pattern with standard diffraction data confirmed that the deposited material is the hexagonal LaF3. The individual crystalline size in the films has been determined using Scherer’s formula, and was found of the order of 9-13 nm. PL of various LaF3-passivated PS samples were investigated. An excitation wavelength of 320 nm was used for the PL studies. We obtained Gaussian shaped PL with a peak around 636 nm. The PL spectra of PS containing three different layers of LaF3 have been compared in Fig 8. These results clearly show that no interaction between LaF3 and PS has occurred in the LaF3/PS system under the described condition. The mixture of two materials did not modify their intrinsic properties. The PL intensity was lowest for the 10 reaction cycle where according to the EDX analysis, amount of La and F were very low but oxygen was highest. This reduced intensity was lack of surface passivation. The intensity was highest for the 20 reaction cycle. As the LaF3 got thicker (30 reaction cycle) the LaF3 layer became less transparent and why the intensity was less than that of 20 reaction cycle. 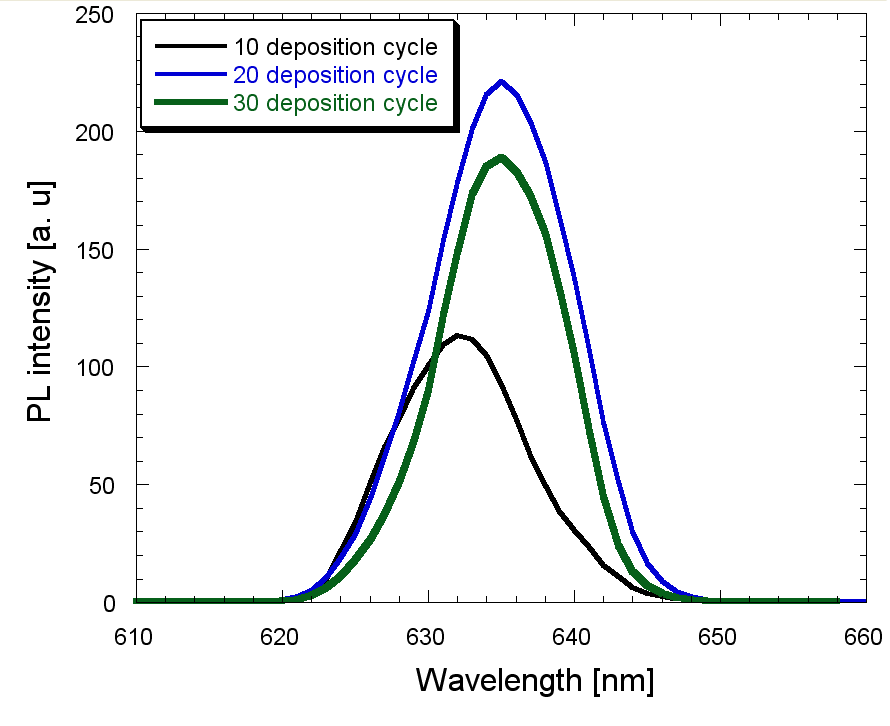 | Figure 8. PL spectra of PS passivated by LaF3 with different reaction cycle |
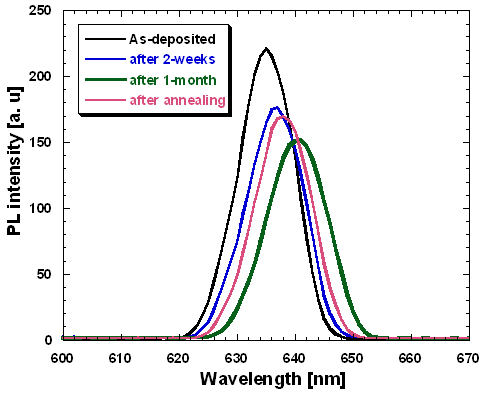 | Figure 9. PL investigation of 20 cycle-deposited LaF3 on PS |
As shown in Fig 9, the PL intensity of the as-passivated PS suffered by aging. When the whole structure was annealed at 400C for 10 minute the PL intensity was recovered. During the chemical deposition of LaF3 oxygen was also incorporated in the LaF3 film probably as water molecule that with time degrades the PL intensity. When heat treated, the oxygen goes away and the PL was recovered.
4. Conclusions
Porous silicon has been tried to passivate with LaF3 deposited by a novel chemical-bath technique that involves only one-step chemical reaction of LaCl3 and HF. The EDX analysis shows that the amount of lanthanum and fluorine increases as the reaction cycle increases. The deposited LaF3 film was amorphous in nature. For a 320 nm excitation the PL peak appeared around 636 nm. The PL showed aging but heat treatment (annealing) could recover the PL intensity due to annealing. It can be concluded that optimized thickness of LaF3 deposited in this novel CBD technique may enable the porous silicon to be an important photonic material.
ACKNOWLEDGEMENTS
This work has been supported by the TWAS and COMSTEC research grant.
References
[1] | Boukherroub R et al. (2000). “Thermal route for chemical modification and photoluminescence stabilization of porous silicon”, Phys. Stat. Sol. (A), 182, 117-121. |
[2] | Gelloz B et al. (2003). “Stabilization of porous silicon electroluminescence by surface passivation with controlled covalent bonds”, Appl. Phys. Lett., 83, 2342-2344. |
[3] | Prabakaran R et al. (2008). “The effects of ZnO coating on the photoluminescence properties of porous silicon for the advanced optoelectronic devices”, J. Non-Crys. Sol., 354, 2181–2185. |
[4] | Rahmani M et al. (2008). “Photoluminescence enhancement and stabiliz ation of porous silicon passivated by Iron”, J. Lumi., 128, 1763-1766 |
[5] | Macedo A. G et al. (2008). “Enhanced lifetime in porous silicon light-emitting diodes with fluorine doped tin oxide electrodes”, Thin Sol. Films, 517, 870-873 |
[6] | Thoma E. D, Shields H, Zhang Y, McCollum B. C, Williams R. T (1997), “EPR and Luminescence Studies of LaF3 and CeF3 under X-Ray and Laser Irradiation”, J. Lumin.,71, 93 - 104. |
[7] | Lide D. R (2007), “Physical Constants of Organic Compounds”, CRC Handbook of Chemistry and Physics, (87th Edition), Taylor and Francis, Boca Raton, FL. |
[8] | Hopkins R. H, Hoffman R.A, Kramer W. E (1975). "Moisture resistant optical films: their production and characterization", Appl. Opt., 14, 2631. |
[9] | Khatun H, et al. (2010), “Investigation on LaF3/porous-silicon system for photonic application”, Chin. Opt. Lett., Accepted for publication. |
[10] | Macleod H. A (1982). "Microstructure of Optical Thin Films," Proc. Soc. Photo-Opt. Instrum. Eng. 325, 21 |
[11] | Wolkin M. V, Joune J, and Fauchet P. M (1999), “Electronic states and luminescence in porous silicon quantum dots: the role of oxygen”, Phys. Rev. Lett. 82, 197-200. |
[12] | Maruyama T. and Ohtani S (1994), “Photoluminescence of porous silicon exposed to ambient air”, Appl. Phys. Lett. 65, 1346-1348. |
[13] | Patnaik P (2002). “Handbook of Inorganic Chemicals”, McGraw-Hill Professional, New York. 448. |
[14] | Herino R (2000). “Nanocomposite materials from porous silicon”, Mater. Sc. Eng. B, 69–70, 70–76 |