Evgeny M. Yarichin
Trofimuk Institute of Petroleum Geology and Geophysics of Siberian Branch, Russian Academy of Sciences Novosibirsk, 630090, Russian Federation
Correspondence to: Evgeny M. Yarichin, Trofimuk Institute of Petroleum Geology and Geophysics of Siberian Branch, Russian Academy of Sciences Novosibirsk, 630090, Russian Federation.
Email: |  |
Copyright © 2012 Scientific & Academic Publishing. All Rights Reserved.
Abstract
Considered is a modern informational approach (post-Shannon’s era), suggested by the author in accordance with the methodology of modern fundamental science. This allowed one to substantiate coincidence of a notion of information with a notion of full energy-momentum tensor of a material system formed as a result of a strong interaction (interaction-measurement) of its physical and structural components in the effective Riemannian space-time. The fact that information contains physical (quantitative) and structural (qualitative) components gives grounds to introduce a new term - “full information”. An equivalent term - video-information - is best suited for some applications (for example, for technical vision). A relationship between the structural and physical components of video-information in general form is established with the help of conservation laws. Derived is a system of informational equations which partial solution shows that the correspondence principle holds true for the post-Shannon’s information approach, which can be considered as a development of the information approach to a more complex class of problems where the classical information approach is not applied. It is shown that video-informational processes border formally on paradoxical ones. Therefore, consistent study of video-informational processes is possible only in a weak field approximation when information becomes weak and characterizes space properties of the real outworld only “in the small”.
Keywords:
Interaction-measurement System Component, Flat Minkowski Space-time, Curved Effective Riemannian Space-time, Full Information, Video-information, Video-information Field, Weak Video-information, Field Equations of Video-information
Cite this paper: Evgeny M. Yarichin, Theory of Full (Video) Information: Bridging of the Gap between Quantity and Quality in Modern Paradigm of Information, International Journal of Information Science, Vol. 3 No. 3, 2013, pp. 37-56. doi: 10.5923/j.ijis.20130303.01.
1. Introduction
The present level of scientific understanding of the material outworld is enough to lay down an informational approach to the real world description, for example, to develop an information model of its visual perception and understanding with technical vision[1, 2]. The classical theory of information had a little effect on fundamental sciences, physics in particular[3]. It seems that too high expectations have been placed on mathematical identity of information (rather on the amount of information) and entropy, but they are not realized yet.The classical theory of information nowadays deals with the problems of information processing and transfer considering redundancy, noise, losses and coding. The theory of information has not addressed the fundamental problem of information creation leaving open the solution of the question: what is information and what are nonsense and noise. Generally the notion of information is not related to the characteristic aspects of statistical regularities («information in itself is not a special probabilistic notion»[A.N. Kolmogorov]). The post-Shannon’s informational approach considers this fact and allows one to give a subject second thought to the manifestation of quantitative (physical) and qualitative (structural) properties of the material world fully in accordance with the methodology and ideas of the present-day fundamental science.
2. Present-day Analysis of Physical-geometrical Properties of the Real World Objects
You can consider all the objects and processes of the real world as time, space and space-time physical structures often of topological nature (points, lines, surfaces, bodies). Therewith, information is a manifestation and a registered measure of various unary and binaryinteraction-measurements that construct and represent the real world[1]. In this connection one may consider that the laws of nature and society ensuring via definite interaction-measurements stable existence (conservation) of the real world and its social order manifest themselves by information as an adequate measure of these interaction-measurements[1]. It is proved at present that there are no substantive informal theories of ternary, tetrad and other interaction-measurements generating appropriate relations and systems of relations[4]. Hence, the nature has confined itself to unary and binary relations as real and complex numbers, and to unary and binary systems of relations as structures. In some complicated cases of multiple interaction-measurements in a system consisting of three, four, five components and etc. one should consider ranks of interactions. Therewith, the interaction-measurement of rank
builds up so many paired relations (numbers) or sets of relations (structures) as combinations of components by two one can build up, namely,
. Thus, the informational paradigm of the world (nature and society) as a system of concepts reflecting essential features of the reality at a macro level of the world outlook can be formulated as: Any stable existing system (natural or social) consisting of the interacting components with quantitative (physical, force) and qualitative (structural, abstract) properties is a result of the interaction-measurement of some rank and appears in the outworld as a measure of such interaction in the form of full information (quantitative and qualitative together).The use of the measure as a numerical and/or structural parameter of interaction, which can be both physical (force, energy-material) and structural (non-force, mathematically formal), is a scientific basis of the notion of measurement in “observations” of different nature. For example, information in technical vision as a measure is a far-reaching generalization of conventional numeric parameters (length, area, volume) of a geometrical figure that can be considered as relations. In this case, the geometrical figure is rather a family of sets (points, lines, surfaces) connected by definite systems of relations producing structures. This structures can be expressed (represented, “measured”) only with “measured” structures corresponding to some standard geometrical figures (forms). The varieties of the Euclidian symmetric forms (sphere, cone, cylinder of a regular even form without external irregularities) useful for preliminary evaluation of the form of a three-dimensional object can serve as an example. It is better to describe the phenomenology of the complex forms of natural objects that are not submitted to preliminary calculation within the frames of fractal and multifractal geometry, i.e. as a combination of irregular and geometrically chaotic structures that form measured structures allowing a more realistic representation (evaluation) of the form of the real world objects. It should be mentioned that the informational paradigm considering full (both physical and structural) interaction between the matter forms of the super sophisticated outworld as a basis of the informational world outlook gives just a general idea about the nature of information. To refine this idea one should consider simpler material systems where one can describe explicitly the Lagrangian density of the system forming interaction between the components of such a system[1].
2.1. Overall Physical-geometrical Relations in Activated (Binary) Material System
One of the manifestations of the video-informational ability of the matter is an evidence of the existence of material world objects within fixed boundaries (objects) forming different surfaces and hyper surfaces (in the Minkowski space). One may describe these surfaces in the Riemannian space-time that arises as a method of metric calculations in the Minkowski space.Let’s accept that the following principle of representation identity is the basis for video-informational ability of the real world representing a host of material objects informing about their existence by primary and/or secondary video-informational fields:Full information (video-information) as an aggregate of physical,
and structural,
properties of the material system considered together in the Minkowsky space with metric,
, can be identically presented by the physical properties in the effective Riemannian space-time with metric,
, induced by the structural properties of the system. Let’s offer an informational interpretation of the classical Lagrangian formalism which starting point is the construction of the action function. Actually, the action function (functional) in a conventional notion of an integral of a nonnegative function taken over some line, volume or some space-time domain is a natural generalization of the notion of the measure[5]. In its turn, the measure of the interaction-measurement in some material system (active, passive or activated) is interpreted naturally as appropriate information (unary or binary)[1]. The key point of the developed informational approach is a principle of representation identity (principle of video-informing) which, on the one hand, determines the equivalence of two techniques for describing physical and structural properties of the matter considered together. On the other hand, this principle defines the choice of the interaction Lagrangian of the physical and structural components of the matter without concrete definition of the nature of video-information which may be of any type (optical, thermal, radio wave and ultrasound). Let’s use the earlier built general Lagrangian density of an activated (binary) material system formed from the interaction of its physical and structural components[1]. The source scalar Lagrangian density of such a system is given by 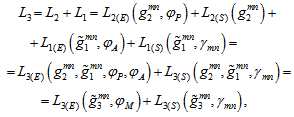 | (1) |
where
is the Lagrangian density of an active material subsystem generating a primary video-informational field used for activation in the effective Riemannian space with the metric tensor density,
is the Lagrangian density of a passive material subsystem generating a completely geometrized video-informational field in the general Riemannian space- time with the metric tensor,
[1];
is the Lagrangian density of a binary system generating a secondary video-informational field in the effective Riemannian space-time produced by the product of the metric spaces of the active and passive material subsystems with metric tensor densities
and
, respectively, which is equivalent to the sum of these metrics[1]; 
is the field tensor density of the structural component of a binary system;
is the physical field of a binary system ; is the physical field of a passive subsystem; is the physical field of an active subsystem; is a composition symbol (composed function
) appropriate to the whole of operations: simulation, realization and presentation (multiplication, convolution, substitution, nonlinear superposition), which allows one to present in a condensed form exceedingly lengthy mathematical expressions that do not require particularization. Expression (1) represents the Lagrangian density of the binary system formed from the interaction of the primary video-informational field and nonradiative surface of a controlled material object. Therewith, tensor field densities
and
of the physical and structural components of the binary system generate together amalgamated tensor density,
of the secondary video informational field inducing the effective Riemannian space-time where the principle of presentation identity holds true (this time for secondary video-information).Let’s use, following the Lagrangian formalism, invariance of action 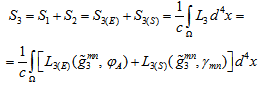 | (2) |
with infinitely small coordinate variation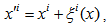 | (3) |
where ∫ − denotes multiple integral, ∫…∫, over some four-dimensional area
of the space-time with coordinates (aggregate of independent variables)
;
are physical and structural components of action ;
is infinitely small four-vector of displacement.The nature of the space-time geometry allows deriving of conservation laws of a complete system of interacting matter forms. Besides the integral conservation laws typical of the pseudo-Euclidean space (the Minkowski space), it is common practice to define two differential conservation laws- strong and weak ones[6]. Strong conservation laws present differential identities executing by invariance the action functions in a corresponding system with arbitrary coordinate transformation. One obtains weak conservation laws considering matter field equations in strong conservation laws. The function action invariance means that with the arbitrary infinitely small coordinate transformation (3) functional variation from action is zero 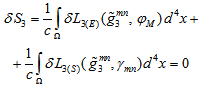 | (4) |
Using the metric form of the Lagrangian formalism; that is with no consideration of divergent expansion terms in the action functions of the binary system components, it is possible to obtain via standard calculations functional variations from the action of physical and structural components of the binary system. Following a summation thereof we obtain subject to (4) an expression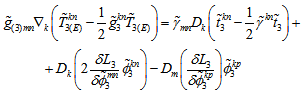 | (5) |
In this expression 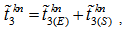 | (6) |
is the density of full energy–momentum tensor of the binary material system in the Minkowski space;
and
are the densities of energy-momentum tensors of the structural and physical components of the binary system in the Minkowski space, respectively;
is a symbol of covariant derivative in the effective Riemannian space-time ;
is a symbol of covariant derivative in the Minkowski space;
is the density of energy-momentum tensor of the physical component of the binary system in the effective Riemannian space-time ;
is a trace of the density of energy-momentum tensor of the physical component of the binary system in the effective Riemannian space-time;
are physical and structural components of energy-momentum tensor of the binary system in the Minkowski space;
is a trace of the density of energy-momentum tensor of the binary system in the Minkowski space.In line with the principle of least action the field equation of the binary system may be presented as 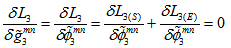 | (7) |
Considering these equations in Expression (5) we obtain the identity | (8) |
One can determine the density of full energy-momentum tensor of the activated system (activated video-information) by the following formula[6]
Identity (7), in view of these and above given formulas, may be written as 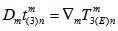 | (9) |
This equation- with due account for video-information identification with the density of full energy-momentum tensor of the material system in the Minkowski space- may serve as a formal substantiation of the principle of representation identity (principle of video-informing) accepted earlier[1]. Subject to this principle field variables are considered in global coordinates in the Minkowski space and in local coordinates in the effective Riemannian space. Since the effective Riemannian space has a “flat background”, its local coordinates are always the global coordinate functions of the Minkowski space. The fact that the structural component of video-information (partially geometrized and thus possessing definite physical properties) may be excluded from consideration when one describes the properties of the binary system in the Riemannian space-time generated by this video-information component is a result of the field approach application. In other words, the energy of the structural component of video-information (SCV) in the effective Riemannian space-time coordinates goes to the creation thereof. It is found that in the coordinates of the effective Riemannian space-time curved by the structural component of video-information the physical component of video-information (FCV) alone remains energetically significant. When conservation equations are applied to the physical component of the activated (binary) material system, equation (9) becomes 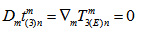 | (10) |
Since in line with Equation (10) equation of conservation of full energy-momentum tensor density in the Minkowski subspace − equation of conservation of full information or video-information −  | (11) |
is equal to covariant equation of conservation of video-information physical component in the effective Riemannian space-time, which, in its turn, is equal to field equations of the physical component of the unary system considered, one may use relation (11) instead of field equations of the physical component. This relation testifies that the structural component of video-information
in the Minkowski space coordinates behaves like the physical component of video-information,
, that is video-information,
, as a whole is an additive form of joint contributions of video-information of physical and structural components of the binary system considered.Conservation laws (10) and (11) present different recording formats of one and the same conservation law. From a conservation equation for the physical component of video - information in the effective Riemannian space-time it is not clear what is conserved, since in view of the covariant recording format this law is not the conservation law in the usual sense. In its turn, from the law of conservation of the density of full energy-momentum tensor
, in the Minkowski space it follows that the full energy-momentum tensor of the activated material system is the one conserved second rank tensor. Thus,
as the one conserved operator may be chosen as a source of the secondary video-informational field keeping to the cannons of classical theory. Thus, considering binary video-information as a source of the secondary video-informational field it is possible to identify full energy-momentum tensor
, of the binary material system with binary video-information as a measure of the physical-geometricalinteraction-measurement between physical and structural components (subsystems) of the binary system.Let’s point out formal analogy between gravitational and video-informational processes as a suggestive principle. Compatibility of physical and structural properties of the material world is a phenomenon that formally unites gravitation and video-information. A specific feature of this combined properties manifestation is that such a complicated interaction thereof is possible in curved space only. For gravitational processes curved space-time is generated by the physical matter properties (gravitating mass of the matter), whereas for video-informational processes it is generated by the structural properties of the matter (for example, by a complex space form of real objects ) which should be partially geometrized therefore, that is be something other than mathematical abstraction. Within the context of the suggested informational paradigm of the world, gravitational processes are likely to be considered as the processes of interaction-measurement over a range of gravitational (very-long) waves generating stable existing unary, binary or other ( including high rank) cosmic scale systems. This may imply a principle possibility of visualization of gravitating objects of the Universe, provided that the problems of gravitation wave reception and aperture synthesis of a relevant video-receiver are solved. Gravitational field under normal conditions is so weak that it is not yet registered at instrumental level. Video-informational fields of another physical nature are readily available and can be instrumentally generated and registered over a wide range of wave lengths of radio-wave, acoustic and optical radiation. Therewith, video-information considered as a source of the appropriate primary and secondary informational fields becomes weak due to considerable attenuation of these fields by the paraxial nature of physical channels of their distribution. Image that may be readily registered physically over a range of wavelengths of the appropriate video-informational field (radio wave, acoustic and optical) is a traditional form of the weak video-information evaluation. An image obtained may be used to activate video-informational processes of technical vision of high and super high hierarchy levels supporting structural interaction-measurements that yield video-information estimations interpreted as perception and/or “understanding” of weak video-information.
2.2. Complete System of Video-informational Equations of a Binary Material System
A system of video-informational equations of a binary (activated) material system[1] | (12) |
 | (13) |
may be set up provided that one derives an explicit system of equations for a secondary video-informational field which out of the principle of least action can be written as[6] 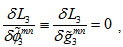 | (14) |
where
This is just possible with the known Lagrangian density,
which structure should be determined. It is better to take a gauge group of video-informational field transformations as a basis of such a construction and use a classical gauge principle. Subject to this principle the Lagrangian density of a relevant system of interacting fields changes to divergence only[7, 8, 9] when fields, which parameters depend in an arbitrary way on the point of space-time and are called gauge transformations (unlike global transformations which parameters are constant), are transformed. Complete system of video-informational Equations (12), (13) is similar to generally covariant equations of electrodynamics[1]. But in electrodynamics electromagnetic field is a vector one and conserved current density is a source thereof. The video-informational field being a physical-geometrical one becomes tensor independent of its nature (radio wave, acoustic and optical). Therewith, binary video-information presented by a sum of its physical and structural components is the source of the secondary video-informational field. Equation (13) excludes spin states 1 and 0’ leaving polarization field properties corresponding to spins 2 and 0[6, 10, 11]. The nature of activated video-information as aphysical-geometrical measure of the interaction-measurement between physical and structural components of the binary material system allows one to consider this system as some “measuring instrument”. Physical-geometrical “readings” of such an “instrument” will correspond to binary video-information (both physical and structural) that is the source of the secondary video-informational field generated in such a system. The use of the classical gauge principle for the sought Lagrangian density construction allows one to go from space transformations to gauge ones that are above-coordinate transformations acting on the vectors and tensors of the spaces of intrinsic field characteristics rather than on space-time vectors and tensors. Since metrics
, remains unchanged upon the calibration transformation, one can obtain the variation in the Lagrangian density for divergence only if we introduce strong restrictions on the structure of the Lagrangian density, what is typical of gauge transformations[8, 11]. Coordinate transformations, in its turn, do not impose any restrictions on the structure of the Lagrangian density of the material system. The fundamental difference between the gauge transformation and coordinate transformation lies in this. One may apply a well-known method that uses directly the properties of calibration invariance and plays the key part, for example, upon construction of a theory of non-Abelian calibration fields. Let’s mention that in line with the theory of interacting fields the Lagrangians may contain quadratic field items and items of cubic, fourth and higher order by field. Quadratic items result in linear items of field equations, and the higher order items lead to non-linear items. It is easy to solve linearized equations, whereas it is impossible to find general solution of nonlinear equations. The fulfillment of the requirements of the gauge principle regarding the structural component of the activated material system defines uniquely the structure of the appropriate Lagrangian density component that assumes the following form[10, 12]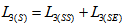
where
is the third rank tensor against general coordinate transformations. In this equation fully geometrized generatrix,
and partially geometrized generatrix,
of structural component,
take the following form
,Numerical factor
is a characteristic of the energy - materiality (corporeality) of a partially geometrized material envelope of a binary system (as part of an observed passive object of a complex form activated by an external field) at rest corresponding to a statistical condition when a secondary video-informational field is missing. The total Lagrangian density of the binary system may be written as | (14a) |
With the principle of least action based on (14a) it is possible to derive a complete system of equations for the binary system of interacting physical and structural field components: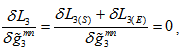 | (15) |
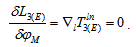 | (16) |
Such a system of equations with 15 unknown and 14 equations may be brought to the following system of equations[12]
where
is the second rank curvature tensor of the effective Riemannian space generated by the structural component of binary video-information;
is the scalar curvature of the effective Riemannian space of the binary system. Such a system of equations describes in general video-informational processes generated by the interaction-measurement between the structural and physical field components of the activated material system. The system of equations includes the metric tensor of the Minkowski space, and all field variables of the system of equations depend on the Minkowski space coordinates. It is the introduction of number factor
, allows one to derive the equations of the binary video-informational processes in the Minkowski space. These equations include the physical component of video-information in the effective Riemannian space, Riemannian metric, along with the metric tensor of the Minkowsky space. Thus, one may express the metric of this space in terms of the metric characterization of the effective Riemannian space. Numerical factor
transgresses a gauge group and thus eliminates degeneration against above-coordinate gauge transformations. One may consider inclusion and accounting of
as a parameter characterizing video-informational process from the energy-material side (material, physical) as some technique. The essence of this technique is that the arrangement of interaction between the structural and physical field components of the binary system followed by vanishing of numerical factor
provides the physical nature of the interaction-measurement between the field components of the binary system. As consequence, a stable existence of such a system followed by generation of a secondary video-informational field with binary video-information as a source is provided. Formal transition to numerical factor,
equal zero allows one to derive video-informational equations of the binary system of the following “simplified” form  | (17) |
 | (18) |
where
is the energy-momentum tensor density of the physical component of binary video-information in the effective Riemannian-space time;
is a trace of the physical component of binary video-information in the effective Riemannian space-time.A complete system of equations (17), (18) is similar to a system of equations (12), (13) and corresponds to a stable existing binary (activated) material system.Interaction-measurement of physical and structural field components is a system-forming factor of such a system. As a measure of such an interaction-measurement one may consider the density of the full energy-momentum tensor of the binary system in the Minkowski space; i.e. binary video-information. A system of equations (17), (18) coincides formally in appearance with a complete system of equations for the matter and gravitational field of the relativistic gravitation theory[6, 10, 12], but has another (video-informational) meaning corresponding to a global physical-geometrical description of a binary material system in the Minkowski space coordinates. Indeed, all field variables of the system of equations (17), (18) represent variables of the Minkowski space.
2.3. Structure of Binary Video-information and Interaction of its Physical (Quantitative) and Structural (Qualitative) Components
Whereas the peculiarities of the video-information structure in the Minkowski space are obvious for a binary form of video-information, such clarity is lacking in the Riemannian space-time. To achieve this, one should use a more general (more cumbersome) form of the Lagrangian formalism and go from the metric analysis of the action function of the binary system to canonical one. In this case one should take into account divergent members of the action function, which, after all, allows presenting the system of video-informational equations in a more general (canonical) form | (19) |
 | (20) |
Here
is the physical component of binary video-information in the effective Riemannian space;
is the canonical form of the structural component of binary video-information taking on the form | (21) |
where
. Owing to equations (19), (20) the law of conservation of binary video-information takes the form | (22) |
The covariant law of conservation of the physical component of binary video-information in the effective Riemannian space may be presented as follows: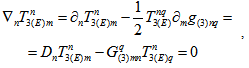 | (23) |
where the third rank tensor against general coordinate transformations,
, is given by
Comparing expressions (22) and (23) one obtains identity 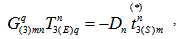 | (24) |
from which it follows that ● The physical and structural components of binary video-information – when the conservation laws are strictly met – are so interrelated that the structural component of video-information may be treated as a source of activation of the physical component of video-information . One may readily extend this result to unary video-information considering the formal analogy of the Lagrangian densities of the binary (activated) and unary (active) material systems[1]. Based on the above obtained results one may generally describe generation and transfer of video-information in space-time as follows:Video-information is a physical-geometrical measure of the interaction-measurement between the physical and structural components of any stable existing material system (unary or binary) possessing, in line with the strong conservation law, the one conserved second rank tensor in the Minkowski space which being provided with a complete set of physical-geometrical properties is a natural source of video-informational field (primary or secondary). One may achieve video-information transfer in space-time using a video-informational field of any physical nature in compliance with a weak conservation law resulting from a strong conservation law providing that it considers field equations for system-forming components of a relevant material system. The principle of representation identity known as a principle of video-informing applied to video-information allows one, coupled with a field approach based on the principle of least action, to lay down a notion of video-information as the only source of video-informational field. This field may be fully geometrized (passive) and thus exist within the boundaries defined by the form of a material object, or partially geometrized (active, activated) representing a primary or a secondary video-informational field. Therewith, geometrization is executed on a basis of simple geometry consisting in complex aggregate of axioms (more than two tens) and primitives for which one takes event points, metric (distance, interval) and continuum domains. For the mathematics simplicity within the context of the field approach it is commonly supposed that the Lagrangian density of the relevant material system doesn’t include derivatives above the first-order. As a result of this limitation the equations of the video-informational field are the second-order equations. But in spite of such a drastic reduction of the reality description to the level of analytically available approximation this approach allows one to determine the nature of the video-informational ability of the real world objects. In line with the principle of video-informing the structural component of video-information (SCV) may be excluded from consideration in the curvilinear coordinates of the effective Riemannian space-time; since its energy goes to generate this space. Therewith, the covariant equation of conservation of the physical component of video-information (PCV)
in the effective Riemannian space-time may be written as  | (25) |
The conservation law in the effective Riemannian space-time is not the conservation law in the usual sense. Indeed,
should not be conserved, since
. In this case the second summand in (25) shows that the physical component of video-information receives energy as if “accumulated” in the effective Riemannian space-time. This activating energy with which the effective Riemannian space-time is induced coincides with the energy of the structural component in the Minkowski space. Video-information (active or activated) is presented in the effective Riemannian space-time by its physical component only. It is peculiar to these kinds of video-information in the effective Riemannian space-time that the structural component thereof is not involved in video-informational field generation. As in the case of passive video-information, the structural component of active or activated video-information in the effective Riemannian space-time goes to zero outside the material object envelop, and for a free video-informational field we have 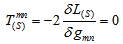 | (26) |
But it doesn’t follow from Expression (26) that the video-informational field is lacking the structural component, since another approach is required to determine an energy flow of video-informational field because this energy flow is not defined by density tensor components,
, of video-information calculated from the solutions of Equation (26), where they are zero. In[10, 13] the solutions of equations like (26) are considered as
, where values
an
are of one order of vanishing, whereas
describes waves diverging from the source, and
describes background. Energy transfer is executed by diverging waves only. Indeed, the energy flow is determined by value
, calculated from that part of the solutions of Equation (26) that describe diverging waves.Let’s consider within the context of the metric analysis the structure of binary video-information in the Minkowski space that is the one conserved source of the secondary video-informational field of the binary (activated) material system. By Expression (6, 11) binary video-information in the flat Minkowski space-time may be given by 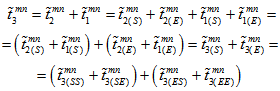 | (27) |
where
is the structural (partially geometrized) component of binary video–information (SCV);
is the physical (partially physicalized) component of binary video-information (PCV);
is the fully geometrized structural generatrix of SCV;
is the physical generatrix of SCV;
is the partially geometrized structural generatrix of PCV;
is the physical generatrix of PCV. Here, the structural component of video-information (SCV) of the binary system expressed by the components of unary video-information composing it or appropriate generatrices takes the following form | (28) |
The physical component of video-information (PCV) is built along similar lines  | (29) |
The availability of a pair of partially dual components having a pair of dual generatrices enables one to hypothesize on the natural fractality of binary information.In line with this hypothesis it is believed that the structure of video-information possesses self-similarity assuming the availability of scaling invariance or scaling. Indeed, according to expressions (27) - (29) binary video-information consists of the physical and structural components, each of which is broken into two dual (physical and structural) generatrices. In this connection, the structure of binary information is similar to that of a tree (a trunk splits into two separate branches, each of which is a trunk for other smaller branches) and resembles a structure of the simplest fractal (Figure 1).From Expression (29) it follows that the physical component of binary video-information
, is generated upon superposition of fully physicalized generatrix
of PCV and physical generatrix
of SCV of the considered binary system. Once generatrix
, possesses energy, this testifies to the corporeity (energy- materiality) of the material envelop of an activated passive object in the binary system «passive object – activating field», which generates the effective Riemannian space-time. In its turn, field generatrix
, being fully physicalized is a carrier of energy of an activating field. 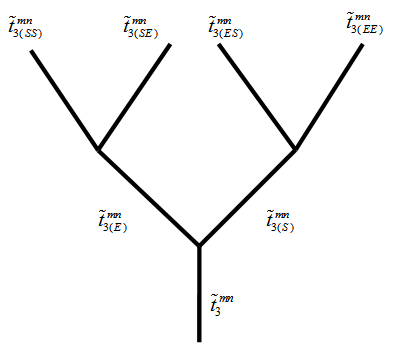 | Figure 1. “Fractal” of binary video-information determined by the structure of its physical and structural components, each of which is partially physicalized or geometrized |
In line with Expression (28), the structural component of binary video-information results from superposition of the following structures: fully geometrized structural generatrix,
, of SCV (of the surface structure of an activated object) and structural generatrix,
, of PCV (of the structure of an activating field front) of the considered binary system. Actually, this operation is a mathematical-formal interaction of the structures in the Minkowski space of the binary system which agrees with the effective Riemannian space-time with the metric tensor density
where
is the tensor of a fully geometrized video-informational field in the general Riemannian space that coincides with the metric tensor of the passive object surface of the binary system «passive object − activating field»;
is the tensor of an activating field in the effective Riemannian space with the metric tensor density
is the tensor of the secondary video-informational field of the binary system.An activation result takes on a form of binary video-information which is a conserved source of the secondary video-informational field in the Minkowski space, and is represented by the physical component of binary video-information in curvilinear coordinates of the effective Riemannian space-time. But looking far ahead, it should be noted that the last condition is not of primary importance in technical vision. Indeed, technical vision as a process of video-information (unary or binary) evaluation is invoked by weak video-information – a source of a weak video-informational field. Paraxial approximation holds independently of the nature of the weak video-informational field as the field propagates. Unlike general video-information weak video-information exists in the Minkowski space coordinates only where image is a conventional estimator of weak video-information. The results obtained for binary video-information may be extended to unary (active) video-information, including its structure. The only but significant difference is inability, in unary case, to activate structurally the passive component of such a material system. Indeed, one may examine active (“self-luminous”) material objects as objects with the unstructured internal activation of their observable surface. Thus, the above-given analysis testifies thatvideo-information may comprise own and joint physical-geometrical properties of material systems that become apparent by their primary and secondary video-informational fields. Therewith, video-information is a physical-geometrical measure of the interaction-measurement between the matter forms functioning as the components of an appropriate material system and may be of complex structure (Figure 2). All these considers video-informational paradigm which through the development of the above-given informational paradigm of the world may be formulated in expanded form as:Any stable existing material system of the real world is generated by the system-forming interaction(interaction-measurement) of the material components (matter forms) constituting it. Therewith, video-information – a source of video-informational field (primary, secondary or passive) – is a physical-geometrical measure of such aninteraction-measurement. Active or activated video-information is always represented by a sum (additive composition) of its physical and structural components in the global coordinates of the flat Minkowski space. The same video-information is presented by the physical component alone in the local coordinates of the curved Riemannian space. 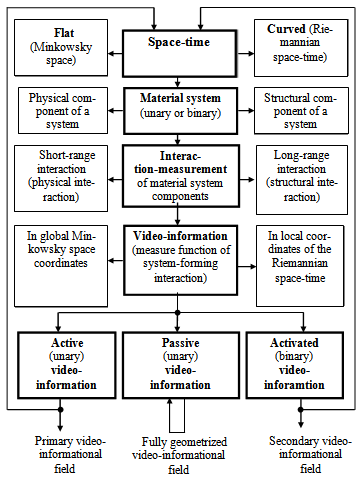 | Figure 2. Physical-geometrical aspects of video-information and video-informational fields |
3. Video-information and Weak Video-information: Quantitative and Qualitative Aspects of the Problem
One may state in line with the modern informational paradigm that full information or video-information proves itself in full (both physical and structural) interaction-measurements[1]. These interactions ensure stable existence (conservation) and variety of the space forms of the real world objects (material systems). A more complete representation of material systems is their video-information (unary or binary) that serves as a measure of the system-forming interaction-measurement between the intrinsic components of the active (self-radiating, unary) system or reciprocal components of the activated (reradiating, binary) system consisting of two independent material systems. Naturally, video-informational equation in the general case is a non-linear one and therefore its solution is a transcendental complex problem. There are two ways to avoid the difficulties. The first way is a long and a complex one connected with the search for a partial solution of exact field equations of video-information. The second way is a simpler one and reduces to a consecutive study of the solution of video-informational equations in weak field approximation. This approximation holds true at the initial stage of video-information evaluation in modern noncoherent and coherent systems of technical vision.The problem of the nonlinear video-informational equation solution nonsimple in itself becomes more complicated as it requires an answer to the question on the correlation between the qualitative (abstract: geometrical, logical) and quantitative (physical, energy-material) aspects of video-information as one of the most compound kinds of information. As is well known such a problem is a “stumbling stone” for modern synergetics[14, 15]. Indeed, dealing with quantitative characteristics one may evaluate (measure) them using some all-purpose unit–relation (as a real or a complex number). As modern synergetics sees it “there is no such thing”[14] to evaluate qualitative characteristics, and in this connection possible ways of correlating quantitative and qualitative aspects of video-information are of considerable interest. The fundamentals of this problem are rooted in different interpretations of the world outlook evidenced in ancient philosophy that is in the Plato and Aristotle philosophies. Indeed, the Platos world of ideas (the world of high reality) is independent of a material factor (matter, field) belonging to a lower world of the material reality. Such conception of the universe perceives the laws of the material world as the one describing projections or reflections of the world of high reality alone. But it was Plato who related numbers to bodies believing that only the objects assigned to an appropriate number were subject to investigation. The Aristotle philosophy doesn’t correlate directly numbers and objects and describes relations between the objects of nonnumeric nature.The modern natural-science approach to the fundamentals agrees with the Aristotle philosophy, and it is assumed that the notion of number as a quantitative characteristics of the real world is understood to be a more in-depth notion of a relation correlated in the real world with a ”pragmatic” process called measurement. An object of measurement composes properties existing in connection with empirical objects only, such as physical bodies, electromagnetic and acoustic waves and people. Measurement itself is a way to display a selected property of the matter (information) as a number (relation), some symbol or structure (system of relations). A scale makes the basis of measurement, and numbers, symbols and structures represent units of measurement. From the post-Shannon’s informational paradigm measurements in general represent strong interactions of physical and/or abstract nature between measured values and relevant measurement scales. As a result, stable existing physical, abstract or other (social) systems are developed. Among such systems are solar system, mathematics in whole, a system “teacher − pupils” and a system “president − citizenry”. Information of an appropriate nature will be a measure of a system-forming interaction (interaction-measurement) of the components in these systems. The laws allow one to substitute measurements for calculations (by definite rules, algorithms) that may be computerized. Therewith, the law is understood to be any equation (called as a law equation) for which a value of any one of the variable entering into it may be measured (determined) jointly and standalone by any method or methods; in this case these values of the law equation reduce this equation to identity. In other words, the law equation is nothing but identity specific and valid for any aggregate of the values of the variables (notions) entering into the equation measured (determined) together and standalone. In this case the word together means a set of conditions under which this phenomenon is observed and a complete set of variables (notions) entering into the law equation ( including methods and principles of measurements by these or other means) is measured (determined). The importance and efficiency of the laws are that the knowledge of the laws, exactly their equations, allows reducing the number of values to be measured by technical means (devices); thereby the rest values may be estimated by the law equations, i.e. “by formulas”.While on the subject of measurements, one means the existence of quantitative (extensional) scales expressed by numbers and admitting calculations. Choosing a qualitative (intensional) scale one determines precision and error required to solve the problem. With qualitative scales typical of highly generalized measurements introduction of ranking is possible via comparison rather than via “formula” evaluations. Indeed, quantitative measurements yield an extensional relation by which it is understood to be some set of ordered object pairs from a set over which this relation is given. For example, these may be constant facts stored in a database. Intensional relations as the circuit elements of the database (for example, attributes in the relational database circuit) result from qualitative measurements. In this sense, technical vision – in the context of the theory of measuring- may be referred to complete (both qualitative and quantitative) measurements that yield an aggregate estimate of semantic relations as pairs where the intensional component of the semantic (complete) relation possesses a quality of “name” of the extensional component of the same relation. Qualitative characteristics of the real world are usually realized as the laws of variations of quantitative characteristics in space-time, and one uses complex measured units- systems of relations (structures) to evaluate them. Thus, the notion of relation that grows to the notion of a system of relations for qualitative characteristics makes the basis for describing qualitative and quantitative characteristics of the real world. Since such a system at a set-theoretical level is a relation, one has to consider “relations of relations” describing qualitative characteristics of the real world. Considering that any relation is information the notion of “relation of relations” will be similar to the notion of “information of information”. In line with the accepted terminology this notion may be called metainformation or an information set which components have a definite structure unlike information itself which components are thought to be indivisible further; that is structureless. In this connection information characterized as a relation is a quantitative characteristics of the real world (for example, number as a measure of relations). At the next hierarchy level information is characterized by the notions of class and structures (system of relations) that are qualitative characteristics of the real world (for example, measured structures). Depending on the hierarchy level information may scale into metaclasses of information or metainformation.
3.1. Informational Interpretation of the Solution of Systems Video-informational Equations
Systems of video-informational equations are derived with the Lagrangian formalism based on the Lagrangian density of an appropriate material system[1]. These equations describe existence conditions of the structural and physical components of video-information. Owing to the nonlinear nature of these systems of equations there is no general solution thereof, and one should consider possible partial solutions. It is possible to assume that the systems of video-informational equation for active (activated) and passive video-information coincide in form with a system of equations of the relativistic gravitation theory (RGT)[6, 10, 12] and the system of Einstein’s equations for gravitational field[7]. Partial solutions of these systems of equations are well known, but the traditional astrophysical interpretation thereof distracts from the solved problem, and, namely, into the realm of topical issues on the structure of the Universe. Informational interpretation of partial solutions of the system of video-informational equations is quite another and has not been considered up to date. Let us consider first a “light form” partial solution of video-informational equations (17), (18) for the binary material system. It is easier to consider partial solution of this system of equations from spherically symmetric representations; that is to say for geometrical objects with spherical geometry. This circumstance allows one to use an extended spherical image (ESI) of the real world object, sometimes called as an extended Gaussian presentation[16, 17], to represent an object form. With such a technique the surface area of the object is taken equal to the total weight of a spherically symmetrical body; that is a part of video-information is somewhat dropped out in ESI, but this presentation appears to be the only one for convex objects. In other words, there are no two convex objects with one and the same ESI. In fact, ESI may be treated as mass density distributed over the Gaussian (unit) sphere. Herewith, it becomes possible to represent the object form attributing mass equal to the square of an appropriate polygon face approximating this geometrical object to every point of the sphere. The center of mass of ESI is at the origin of the coordinates, and the complete mass in the center of mass is equal to the complete mass of ESI, which, in its turn, is equal to the total surface area of the object. For convex objects such an image is single-valued; that is only one convex object corresponds to every ESI. This holds true both for a discrete event when mass equal to the square of an appropriate polygon face approximating the real object is attributed to every ESI point, and a continuous event when an object with a smooth curvilinear surface is represented. To find a solution of the system of video-informational equations (17), (18) means to build up the effective Riemannian manifold; that is to find metric tensor,
of this space defining a form of an interval for the spherically symmetrical effective Riemannian space-time. In this case it is convenient to express the coordinate in the Minkowski space as  | (30) |
and the general interval for the spherically symmetrical Riemannian space time takes the following form  | (31) |
where
is a spherical system of coordinates. Going from the statical spherically symmetrical representations one may derive the following partial solution of equations (17) as the principle constituent of the system of equations (17), (18) | (32) |
where
is the radial coordinate,
is the total mass of a spherically symmetrical object set equal to the surface area of the real world object. Comparing the objects similar in form but different in sizes one may normalize their ESI via division by the (full) total mass. It is always possible to introduce a system of units where mass has dimensions of length. Then introducing designation
and believing that mass is used as an equivalent to the surface area one may write expression (32) considering designations
and
as  | (33) |
where
is a value of the surface area of the activated (or active) convex object equal to the total mass of its ESI related to the surface structure (form) of this object;
is the maximum possible value of the surface area of a convex object corresponding to the most complex form of the possible forms of this object. Expression (33) is interpreted conceptually as a dependence of a “graduation scale” or a minimally solvable scale interval of a measuring macro device (for which a material system is taken). Here one considers a maximum of a structural scale of this macro device that is paired with a numerical value as variable
and some instantaneous value of the same structural scale paired with a numerical value as variable
.Let’s notice that the values
and
in expression (33) equal to the surface areas of the objects of some known form and of the most complex of the possible forms, respectively, may be normalized via division by the sum of these areas, which allows one to compare objects similar in form and different in sizes going to dimensionless numbers. It is important that for activated (active) video-information interval (33) of the Riemannian space-time becomes singular (degenerated) when the condition
is met, and losses its physical meaning (there is no adequate “interior” partial solution [10]) when
. For the passive material system, systems of video-informational equations coincide in appearance with the system of Einstein equations[1] which may be written as  | (34) |
where
is the metric tensor of the general Riemannian space-time ;
is the physical component of passive video-information in the same space-time.Initial situation upon solution of these equations is that it is impossible to define expressly video-informational field and the Riemannian space-time based on them alone, which leads to two different solutions of equations, and hence to different Riemannian spaces[10]. These intervals may be expressed as  | (35) |
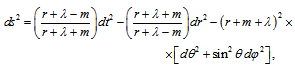 | (36) |
where
is an arbitrary parameter which can be set at different values. It is not easy to choose a solution, since the nature of physical processes relies thereon. In particular, Expression (35) corresponds to an interval known as the Schwarzschild interval; that is to the most popular version of the partial solution of this system of equations – the Schwarzschild solution 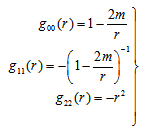 | (37) |
Expression (37) is the best known form of the Schwarzschild which rests the hypothesis of black holes on the possible existence of astrophysical objects near which the matter exhibits wild behavior difficult for prediction by the modern bulk matter physics. This branch of physics is subject to all grey areas and ambiguities of the modern behavior theory of the bulk matter for which there is a bulk of physical information. If however there are thunder-storms and hurricanes on the Earth that do not lend themselves to a precise theoretical modeling, precise prediction of their analogs in the turbulent magnetized plasma with a black hole accretion is questionable. The best in this paradoxical situation is to obtain rather crude models of the matter behavior near the black hole. Bulk physical information in a small volume is responsible for the violent behavior of the matter at the physical level. It seems reasonable to suggest that paradoxically complex qualitative structure of the matter in a small volume is also able to provide its high behavioral complexity, but at the structural (abstract, qualitative) level rather than at the physical one. For example, the great informational abilities that a human brain demonstrates as the one of the most outstanding representatives of the matter highly organized in the structural sense remain incomprehensible. When the human brain is functioning, the intellect of a human being determines the structural aspects of its informational interaction with the outworld (with the aim of its perception). Beyond question is the informational nature of intellect representing a hierarchically organized high-structured information macro-object constantly developing (via education and self-education) and functioning as a qualitative measuring scale able to evaluate the meaning of appropriate information upon informational interactions with the outworld. Therefore, the human brain is able to perceive (understand) only these material processes (physical and/or abstract) information on which may be “measured” by intellect, that is be expressed in its own measured structures. In other words, intellect relative to any information tends to perform the function of an information macro-object (measuring scale) and evaluate (measure) this information in the appropriate measured structures via an interaction-measurement using a “measurement system” thus synthesized. Once such a system is not generated, information is not evaluated by intellect, and the process providing a source of this information remains incomprehensible and may be treated by intellect, for example, as supernatural. Thus, the formation of intellect adequate to the processes taking place in the surrounding material world (nature and society) is of high importance. Inadequacy of intellect may be a result of its formation under dominating outdated conditions or upon a mental decease of a human being. All these lead to unavoidable errors in the world outlook which consequences may be fatal to the society. Ambiguity, uncertainty and complexity of the intelligent systems behavior are the main reason that hinders the creation thereof with the modern facilities. Effective use of the inherent highly organized qualitative (structural,) information arranged in a high-structure information macro-object known as intellect makes the basis of the systems formation. But the lack of intelligent systems theory creates insurmountable obstacle, and an approach known as “artificial intellect” and developed as symbiosis of psychologically supported models and numerous heuristics is other than a theory. The lack of a single meaningful approach yields a mess that complicates objective evaluation of what is intelligent system and what is not. Using numerous heuristics and flexibility of the modern computer technologies one has developed quite efficient “intelligent” systems which principle of operation is not quite evident. Therefore, ‘intelligent” characteristics of these systems are determined not at a design stage, but with diverse testing of the systems already developed at the heart of which is some intuitive psychological paradigm. Let’s retaining designations
and assuming that mass is used as an equivalent to the surface area with parameters
and
write Expression (35) as  | (38) |
Here parameters
and
coincide with similar parameters of expression (6).According to solution (38) there are no space objects (geometric bodies) for which ESI a condition
is met. It seems that a twofold divergence between the results of formulas (33) and (38) is due to the impossibility of unambiguous determination of a fully geometrized video-informational field of a passive material system based on solution (38) of Equation (34) alone.Expression (38) may be interpreted conceptually as a dependence of a “scale factor” or a minimally solvable scale interval of a measuring macro-device (for which a passive material system is taken ) considering a maximum value of structural parameter,
, of the scale of this macro-device and a current value of structural parameter, , connected functionally with a form of a real object. Thus, the results of partial solutions of video-informational equations differ for activated (active) and passive video-information, and there is no great surprise, since the interval defined by expressions (35), (38) cannot be an interval of the effective Riemannian space-time and is an interval of the general Riemannian space-time. Therewith, the interval defined by expressions (32), (33) is an interval of the effective Riemannian space-time. It is assumed generally that the system passive in video-informational sense can represent anything (including a paradoxical physical and geometrical object like “black hole”). If the passive system is capable of physical activation, it represents a material object nevertheless, even though with a fully geometrized video-informational field. This passive (unary) system responding to physical activation with the secondary video-informational field transforms to an activated (binary) material system. The above-given results are obtained with metaphors far away from the heart of the matter as it may be. But such an approach using as a directing theory results from the analysis of paradoxical situations that take place in that section of the modern physics with which there is a formal connection may improve understanding of the key aspects and peculiarities of video-informational processes that are found to border formally on paradoxical processes.
3.2. Weak Video-informational Field
Generation of primary (activating, irradiating) andreception of secondary (reflected, scattered) video-informational fields are practical problems of technical vision. It follows from the data given above that in-depth study of these problems is highly hindered by the nonlinear nature of video-informational equations. Hence, consecutive investigation into the problems of video-informational radiation, reradiation and reception connected with the peculiarities of the primary and secondaryvideo-informational field generation as well as with visual reception thereof becomes possible in a weak field approximation only. Let’s assume that in the entire space time, including the region occupied by a source, the secondary video-informational field is weak, that is | (39) |
Video-informational field equations of the activated (binary) material system may be written (with due account of their analogy with electromagnetic field equations[1]) as  | (40) |
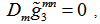 | (41) |
where
is video-information (full energy-momentum tensor in the Minkowsky space coordinates) of the activated material system;
is a covariant derivative in the Minkowsky space.Considering Equation (40) in a weak field approximation it is possible to define variable,
as follows [10]
where
is a solution of a linearized equation (40). It is known that Equation (40) being linearized becomes invariant to the local gage transformation  | (42) |
where four-vector
is an arbitrary infinitesimal parameter (group parameter of a gage group). With such field transformation,
increments (Lie variations),
and
, in linear approximation will be  | (43) |
Thus, the weak video-informational field possessing weak video-information as a source and initiating a process of technical vision keeps the gage principle by which transformation of type (43) is an above-coordinate gage transformation. Therefore, it affects vectors and tensors of the spaces of intrinsic weak field characteristics rather than space –time vectors and tensors. In the Cartesian (rectangular) coordinates of the Minkowski space and in a weak field approximation (39) the following series expansion may be found based on expression :With these expansions and a weak field approximation, such an important constituent of a system of video-informational equations (17), (18) as the second rank curvature tensor will be expressed as
or
.Thus, the system of video-informational equations (17), (18) in a weak field approximation and in the event of
may be expressed as follows in the rectangular system of coordinates: 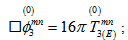 | (44) |
 | (45) |
Here, weak video-information,
, results from general video-information,
, after replacement of
and
in it.The covariant conservation law in a weak field approximation is written as  | (46) |
Weak video-information with no physical component, when
, becomes passive. Equation (18) for passive weak video-information can be rewritten as  | (47) |
Video-information here is fully geometrized and is described by the equation of a characteristic surface (characteristics, fronts) of the passive video-informational field that agrees with the equation of surface of an appropriate passive material object. One can further use a standard scheme of solution of equations (44) coinciding in form with the equation of retarded potentials[7]. Video-information,
, and weak video-informational field,
, are related by the known general solution as a “ retarded” integral  | (48) |
where
is a secondary weak video-informational field;
is weak activated video-information;
are coordinates of video-informational volume;
are coordinates of video-informational field;
is a distance from the element of video-informational volume,
, with coordinates
to the «measuring point» with coordinates
where a value of the field is sought. A triple integral within expression (48) reduced for simplicity may be brought to the form of
subject to the Cavalieri principle known from geometry. From this principle video-informational volume confined by the level surface is easily determined by assignment of the flat sectional areas of this volume normal to axis,
. Let’s write this expression using this principle and disclosing abridged notations of integrals in Expression (48) introduced earlier for simplicity as | (49) |
Here
are coordinates in plane section,
, of video-informational volume;
are transverse coordinates in plane,
, of video-informational volume;
is a selected value of an axial coordinate within video-informational volume. For simplify, axis, , is believed to be coincident with axis, , both in direction and by zero reading;
is a distance from an element of video-informational plane,
, with coordinates
to the point in the «domain of observation» with coordinates
. A triple integral in Expression (49) may be presented as a convolution over coordinates
, which makes it possible to simplify its recording form using a symbolic notation of convolution [18] 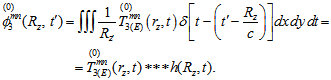 | (50) |
Here
is a weak secondary video-informational field of some cross-section,
, of video-informational volume,
; is a mathematical symbol of convolution;
is the Dirac aggregate function or, in other words, – function;
is a velocity of video-informational field distribution;
– is a pulse characteristic of a layer of homogenous and isotropic (empty) space[18]. For paraxial approximation when
, since near the axis
, the pulse characteristics of the space layer may be expressed in the following simplified form 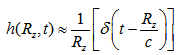 | (51) |
It should be mentioned that the conditions of the paraxial approximation applicability defined by term,
, are negligible owing to the multiplicative action of this term. Thus, the video-informational field (26) in a weak field approximation and empty space-time is expressed as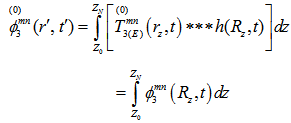 | (52) |
In other words, in a weak field approximation video-informational field in the domain of observation represents superposition of convoluted “cross-sections”,
, of video-informational volume,
, with pulse characteristics,
, of the space layer between these cross-sections and the domain of observation where the video-informational field takes the following form as per(49 – 52):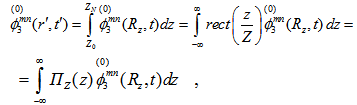 | (53) |
where
is a longitudinal size of video-informational volume along axis,
;
is a rectangular (gating, selection) function determined by expression 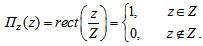 | (54) |
3.3. Video-information in a Weak-field Approximation
Prior to defining a weak primary or secondary video-informational field at the “exit” of the layer of a flat space-time (the Minkowski space) with pulse characteristics,
, one should understand what happens to video-information in a weak field approximation and what information becomes a source of such a field. To do this, one should turn to the principle of representation identity (principle of video-informing) in which the components and generators of video-information (unary and binary) in the Minkowski space and the effective Riemannian space–time are connected generally by the following schemes: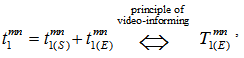 | (55) |
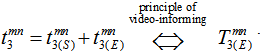 | (56) |
In a weak field approximation, physical components,
and
, of unary and binary video-information in the effective Riemannian space-time change to
and
by substitution of
and
. As a result of such approximation we obtain the just variables recorded in the coordinates of the flat Minkowski space. A peculiarity of a weak field mode is full geometrization of the structural component of weak (unary and binary) video-information. Indeed, the flat space-time as opposed to the effective Riemannian space-time does not require the use of the energy of the physical generator of the structural component of video-information to curve it. In the flat space-time
where, as everybody knows, covariant and contra variant indexes are indiscernible, the following expressions are valid
.These expressions allow one to present the expressions for weak video-information in the form  | (57) |
 | (58) |
Here
is weak passive video-information in the coordinates of the flat space-time derived from general passive video-information of the Riemannian space-time,
, where
is an energy-momentum pseudo tensor. The weak field approximation when
results in the flat space-time for which 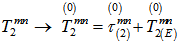 | (59) |
where
is a pseudo tensor in a weak field approximationCovariant conservation laws in a weak field approximation that is in the flat space-time take on the following form with regard to expressions (57) - (59)  | (60) |
 | (61) |
Let’s take into account that in the flat space-time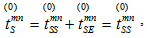 | (62) |
since
, that is the structural component of weak video-information becomes fully geometrized in the flat space-time as there is no need in inducing curvature of appropriate space-time. It follows from expressions (60) – (62) that | (63) |
 | (64) |
These expressions imply that in a weak field approximation the physical (quantitative) and structural (qualitative) components of video-information are in a relation of isomorphism, although they are presented in different units of measurement.It seems likely that this result can be considered as a formal interpretation of a so called “cohesiveness of the matter”[Marr D.] that establishes a correspondence between the physical image of the material object and geometry of its surface.As is stated above, video-information in a weak field approximation may be represented by a video-informational volume simple in structure confined by some level surface, 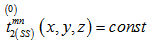 | (65) |
where
. Hence, weak video-information – owing to its full geometrization − may be represented by the structure of this video-informational volume. Thus, one may define weak video-information in the flat space-time where it matches geometrical properties of the observed surface area of the object (active, passive and activated) and is a source of an appropriate weak video-informational field (primary, fully geometrized or secondary). Weak video-information is evaluated by a weak video-informational field (primary or secondary), and this evaluation may be treated as some initial evaluation of the space content of the outworld characterizing its space properties only a ‘in small’.
3.4. Weak Video-informational Field as Space-time Signal
Equations (44) of the weak video-informational field are classical field equations. One may use them for consequential investigation into weak video-information that is a characteristic of the real world space content physically accessible to the technology of technical vision. Let’s write in short the solution of Equation (48) as a “retarded integral” as 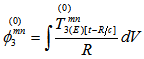 | (66) |
where index given in square brackets indicates that a value of weak information,
, should be taken at the instants of
, and the prime of a value,
, is omitted.Expression (66) can be called a retarded video-informational potential by analogy with the expression for the retarded potential. In line with the known technique of potential expansion in powers of
it is possible to obtain the following superposition of video-informational potentials 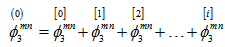 | (67) |
where term,
, is proportional to
.It follows from the given expression that a weak video-informational potential is determined by superposition of monopole,
, dipole,
, quadrupole,
and multipole,
(where
) partial informational potentials composing it. A difference in the surface video-informativity of one and the same object within different frequency ranges of electromagnetic spectrum results from Expression (67). For example, video-informational field within a long-wave (acoustic, radiowave) frequency range featuring a rather low video-informativity (radio and acoustic imaging) is determined with the known Kirchhoff integral formula approximation[18]; that is by superposition of the waves radiated by monopole and dipole sources alone arranged over the surface of the object under observation. In this case Expression (67) may be written as 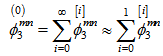 | (68) |
A precise decomposition of video-informational potential through additional wave recording from quadrupole or other multipole sources at the same surface of the object becomes possible within the optical range of electromagnetic spectrum due to a short wavelength; that is 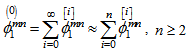 | (69) |
But one should bear in mind that the intensity of video-informational radiation (reradiation) of such sources is characterized by the fifth or higher – order effects by
[7]. This together with the condition of multipole decomposition (67) of video-informational potential
testify to infinitesimal physical (quantitative) effects supporting the key geometrical (qualitative) features of diversified object forms of the real world. Modern video-sensor systems designed to watch the object under appropriate (radio, acoustic, thermal or translucent) environments evaluate video-information processing weak video-informational fields that are generated either by the own (unary) radiation of the controlled objects or their mutual (binary) radiation (reradiation) that can be interpreted in physical sense as reflection or scattering. The structure and parameters of the weak video-informational field (independent of its physical nature) generated in the domain where the field is analyzed depend on the position and space characteristics of the object (its size, form and linear coordinates). Besides, the velocity of the observed object and instability of video-signal propagation medium exhibit their effect[18].Thus, weak unary or binary video-information generates a weak wave video-informational field which effects transfer of appropriate weak video-information upon propagation. In this sense, the weak video-informational field may be treated as a space-time signal. To extract weak video-information a video-sensor system uses not the entire field, but a restricted portion of it that falls within an aperture of the video-receiver. In the general case space and time processing of such video-data are interchangeable and definition of the separation conditions thereof, for example, in quasi-steady-state approximation[18] requires additional consideration.
3.5. Quantitative and Qualitative Aspects of Information
At the initial stage the development of the information theory reduced itself to the analysis of the simplest elementary informational relations peculiar to the interactions of inanimate nature world, where such properties of information as meaning, quality, value and other ones important for informational relations generated by interactions with the participation of the animated nature objects do not usually prove themselves. Hence, a refusal to consider meaning, value and qualities of information in whole is not a drawback of the initial development stage of the information theory and an artificial constraint imposed for simplification, but a natural consequence of the fact that at a level of theory one considered only those informational relations that did not exhibit qualitative properties of information. And a notion of amount of information introduced by Shannon and widely used at the initial stage of the theory development and formation is a characteristics describing informational relations of the real world on one side only. But it is this side has proved to be the most important at the initial development stage of the information theory, since it has laid down a meaningful approach to the development, investigation and evaluation of different technical systems focused on the transfer of information abstracting from the meaning thereof. The Shannon’s classical information theory has a little effect on the modern physics, since great expectations laid on the formal identity of information and entropy have not been justified yet. But it has been found that informational entropy is linked with physical entropy, and a notion of negentropy as a measure of informational entropy reduction or a measure of information obtained therewith has been introduced. The «quantitative» aspect of information is traditionally associated with the notion of entropy. Practically, entropy can be treated as a rather nonobjective way of integrating physical states into larger classes where the elements of each class are considered to be indiscernible among them; that is entropy refers not to separate states but the classes of states[20]. There is a popular opinion that “entropy is a notion with which one may play in a free-and-easy way!”[R. Penrose]. Thus, one should use a certain characteristics of the space-time geometry as a measure of entropy to provide objectivity thereof. Surface area (full mass of ESI) of some space-time object may serve as such characteristics. Then, in line with the notions of information by Shannon
and entropy
, partial solution (33) of the system of video-informational equations for active (unary) or activated (binary) video-information may be written as: | (70) |
Here
is a classical amount of information defined through a priori entropy,
, and a posteriori entropy,
.From relation (70) in full conformity with intuitive understanding of the amount of information,
, it follows that metrics retains sense with
, degenerates with
, and losses sense with
. One may treat Expression (70) as a consistent manifestation of the conformity principle according to which any new theory- having an old well proven one- should not be in full contrast with it, but give the same results in some special case. Therefore, the modern (post-Shannon’s) informational approach for which elaboration one used the paradigm of the fundamental modern science rather than heuristics may be treated as a consistent development of the classical informational approach to the problems where the qualitative aspects of information cannot be set aside. The use of the classical informational approach alone rules out the possibility for deriving a system of video-informational equations and obtaining a solution thereof as (70). Thus, information connected with the notion of entropy is − by Shannon − a very simplified (intuitive) treatment of the notion of information, and this has prevented subsequent investigators of informational processes from “perceiving” the availability of the qualitative and quantitative components by information. The term “amount of information”, introduced by Shannon recognizes intuitively the key characteristic of information - be a measurement result. But it is the intuitive informational approach has not allowed one to perceive the variety of possible measurements: quantitative (physical) and qualitative (structural, mathematical-formal) as well as joint qualitative and quantitative ones. Quantitative measurements give a relation (a number), and qualitative ones result in a structure (a system of relations). In this connection repeated attempts to apply the notion of “the amount of information” for evaluating the qualitative (structural) aspects of informational process were inefficient. Indeed, it is more difficult to understand the modern information theory, since its consequences are amazing and run counter to intuition. In other words, the combined physical and structural nature of information being full in general (qualitative and quantitative) is not apparent. Besides, this fundamental property of information has been disguised by the probabilistic formalism of the classical information theory that is justified upon solution of the problems of statistical communication theory and unsuitable for generation and evaluation of full information (video-information).
4. Conclusions
The modern informational approach as a development of the ideas of[1] assumes accounting of a complete set of the matter properties considered together and corresponds to the aims of the theory of categories which, in line with the modern tendency of axiomatic insight into the theory, suggests to ignore objects, abstract away from them and study relations between the objects alone. But due to a rather complex informational representation of the real world the functional-categorical form of informational approach bordering on metamathematics, metaphysics and even philosophy seems to be too general, since many important physical and geometrical aspects of informational process can be disguised when the probability formalism is used in the classical informational approach. The use of heuristics is typical of initial development stages of technics and technologies. In view of the above-stated provisions of the modern informational approach (post-Shannon’s) one may treat heuristics as a result of intuitive explanation of the causes of a stable existence of some physical, abstract or social phenomenon by human intelligence. This reason is determined in mental form as a macro-component of some hypothetic system-forming (strong) interaction(interaction-measurement) ensuring stable existence of the appropriate phenomenon. At the early stages of the real world perception such a system-forming macro-object intuitively generated takes on a directly visual image. Most often this results in the loss of validity when one shapes the ideas of the real world which being absurd and/or fatal are replaced by new intuitive ideas which validity is tested again by the practice of their using and etc. Sometimes using heuristics one achieves a definite progress involving a good initial explanation of some phenomenon in the absence of deep understanding thereof within the existing scientific paradigm. The formation of such an intuitive concept of a reality situation being other than a valid evaluation of it and partially characterizing this phenomenon permits one to give a direction of a practical phenomenon application. Application of such heuristic notions as amount of information and electric current in actual practice may serve as an example. Only in the process of the knowledge and experience accumulation one obtains theoretical support required for the really sense bearing progress. At present a stage of heuristic informatics development and its applied application – technical vision − is being completed. Further sense bearing and results oriented progress is impossible without a deep insight into completeness and variety of informational processes. In its turn, this is impossible without the expansion of the information paradigm to the level of modern advances of the fundamental physical and mathematical sciences. The present paper deals with a primary study of the theory of full information (video-information) designed to describe the material world properties at a macro-level. The author thinks that this theory can function as a linking science between modern physics and mathematics that are self-contained and often ignore each other. This yields from time to time crisis (dead-locked) situations in perception of the material world which physical and abstract properties are not always considered together. Sometimes one has to develop unique (by complexity, price and scale) scientific devices, of a global level at times, to settle such situations. Theory of full information – when one considers together general physical (quantitative) and structural (qualitative) properties of the matter- has a combinative nature. Thus, the arrival of the modern information theory “in orbit” of fundamental sciences makes it possible not only to use constructively the advances of the modern fundamental science in the theory development, but to generate new and sudden ideas that lie outside loosely connected fundamental sciences (physics and mathematics) that have generated a theory of full information. This permits a better real world understanding through the development of its much problematical fully physicalized picture into a more flexible and well-knit full informational picture where physical (quantitative) and structural (qualitative) properties of the matter are considered together. Evolutionary development of the full informational (video-informational) approach suggested by the author follows the direction of precise, concrete definition and detailed elaboration of the notion of full information (video-information), its components and generators. This modern information approach to the understanding of macro-properties of the material world is based on the recognition of the fundamental role ofinteraction-measurements as strong interactions (physical and/or structural). These interactions executing their system-forming functions structure the super complex world surrounding us and permit one to consider it as a great variety of stable existing complex (in form and content) living and inanimate material systems. Only recently one has perceived a necessity of combined consideration of the physical (quantitative) and structural (qualitative) nature of such systems. This has allowed one to reconsider in the context of the modern informational positions approaches to the construction of intelligent systems which informational nature is not ignored anymore and turns to be determinative. In such a system full information is sensed, perceived and understood as a logically derived result of appropriate measurements at low, middle, high and super high (intelligent) hierarchy levels of the system. As a whole, one should consider the appearance of intelligent systems as a response of the scientific-technical progress to an ever-increasing complexity of the problems facing the human society as it develops. Adequate (intelligent) evaluation of full information is the key one of such problems. One may treat this evaluation of the surrounding world “in whole” as a result of informational “enhancement” of the available estimator of full weak information describing the same properties “in small”. This initial estimator of full information is derived at the stage of sensation of the intelligent system and describes the surrounding world weakly (roughly). The following stages (perception and understanding) of the information evaluation by the intelligent system introduce the required amplification of information changing the initial estimator of full information to the status “in whole”.[21] The modern (post-Shannon’s) information approach doesn’t contradict the classical information theory one, but sets the information paradigm at a fundamental scientific level and permits a proper definition of the notion of information. Theory of full information allows to substantiate the structure of an information mechanism, which for ensure the adequacy of the evaluation of full information should be an intelligent with the need to.[21]
References
[1] | E.M.Yarichin Informational Paradigm of Technical Vision. Pattern Recognition and Image Analysis, 2008, Vol.18, №.1, p.p. 163 – 176. |
[2] | E.M.Yarichin. Theory of Full Information(Video-information). IEEE International Siberian Conference on Control and Communications (SIBCON-2011). IEEE Xplore, Digital Library. |
[3] | H.F.Harmuth Informational Theory Appling to Space-Time Physics. Trans. from English. – M.:Mir, 1989 |
[4] | Yu.S.Vladimirov. Metaphysics. – М.: BINOM. Laboratorya znanii. 2002. |
[5] | А.N. Kolmogorov, S. V. Fomin. Elements of the theory of functions and functional analysis – М.:Nauka. 1981 |
[6] | А.А. Logunov. Lectures on the theory of relativity and gravitation: Modern analysis of the problem. – М. Nauka, 1987 |
[7] | L.D. Landau, Е.М.Lifshitz. Course of theoretical physics: Vol. 10. Theory of field. – 7-th ed., rev. – М.: Nauka. 1988 |
[8] | N.P.Konopleva, V.N.Popov Calibration fields. /3-d ed. rev. – M.:Editorial URSS, 2000 |
[9] | V.А.Rubakov. Classical calibration fields.– М.:Editotial URSS, 1999 |
[10] | А.А.Logunov, М.А. Mestvirishvili. Relativistic theory of gravitation. – М.:Nauka, 1989 |
[11] | S.Weinberg. Gravitation and cosmology. Principles and applications of general theory of relativity. Trans. from Eng. Ed. by Ya. I. Smorodinskii. – Volgograd: Platon, 2000 |
[12] | А.А.Logunov. Theory of gravitational field. – М.:Nauka, 2001 |
[13] | Yu.М. Loskutov. Positive definiteness of gravitational radiation intensity in the theory of gravity with a nonzero graviton mass. Theoret. Math.. Vol. 107, №2. May,1996, p.p. .329-343 |
[14] | H. Haken, М. Haken-Krell. Secrets of perception. Trans. from Eng. – М.: Institut kompyutorrnyh issledovanii , 2002. |
[15] | H. Haken. Information and self-regulation. Macroscopic approach to complex systems. Trans. from Eng. 2-nd ed. – M: KomKniga. 2005 |
[16] | B.K. P.Horn. Robot vision. Trans. from Eng. – М.: Mir, 1989 |
[17] | B.К.P.Horn. Extended Gaussian mapping – TIIER, Vol.72, №12, 1984, p.p. 7-24 |
[18] | Е.I. Kachanov, Е.D. Pigulevsky, Е.М. Yarichin “Methods and means of hydroacoustic holography”. – L.: Sudostroenie, 1989. |
[19] | D. Marr. Vision. Computational investigation into the human representation and processing of visual information. Trans. from Eng. – М.: Radio i svyaz, 1987. |
[20] | R. Penrose. Singularities and time asymmetry. In book.: General relativity theory. Tran. from Eng./ Ed. by S. Hoking. V. Izrael – М.:Mir. 1983 |
[21] | E.M.Yarichin. Informational Mechanism of Intellectual Technical Vision. IEEE International Siberian Conference on Control and Communications (SIBCON-2011). IEEE Xplore, Digital Library. |