Mehrdad Poorhosein1, Hossein Afzalimehr1, Jueyi Sui2, Vijay P. Singh3, Sahar Azareh1
1Department of water engineering, Isfahan University of Technology, Isfahan, Iran
2Environmental Engineering program, University of Northern British Colombia, Prince George, Canada
3Department of Civil and Environmental Engineering, Texas A&M Univ., USA
Correspondence to: Hossein Afzalimehr, Department of water engineering, Isfahan University of Technology, Isfahan, Iran.
Email: |  |
Copyright © 2014 Scientific & Academic Publishing. All Rights Reserved.
Abstract
Bed load transport is of fundamental importance in river engineering. Although there are many bed load transport equations, there is still no consensus on which equation is the best and how to select a particular equation for a particular river. Often there are large discrepancies in the bed load transport values yielded by different equations and between those and observed values. Further, in many practical applications empirical bed load equations are found to produce just as good bed load results as physically based equations. This study develops and tests two regression based equations for two rivers in Iran. One equation is based on hydraulic parameters and the other on geometric parameters. It is found that the Shields parameter, Froude number and shape factor are among the controlling hydraulic parameters of bed load, while the grain size distribution and the water slope are the significant geometric parameters that affect the bed load. A linear function showing more than 60% variability in bed load values seems sufficient to describe the bed load data rather than a non-linear function. The suggested equations are calibrated by laboratory data.
Keywords:
Bed load transport, Geometric parameters, Hydraulic parameters, Shields parameter-sections
Cite this paper: Mehrdad Poorhosein, Hossein Afzalimehr, Jueyi Sui, Vijay P. Singh, Sahar Azareh, Empirical Bed Load Transport Equations, International Journal of Hydraulic Engineering, Vol. 3 No. 3, 2014, pp. 93-101. doi: 10.5923/j.ijhe.20140303.03.
1. Introduction
Bed-load transport is a fundamental physical process in alluvial rivers, constructing and maintaining a dynamically stable channel geometry that reflects both the quantity of water and sediment delivered from the watershed [9]. Bed load transport in rivers is the main link between river hydraulics and river form and has a significant effect on restoring the channel geometry. In addition, the reproductive success of salmonids and other riverine communities are affected by the size of transported sediment on the channel bed and banks [19]. However, because the collection of good quality bed load transport data is expensive and time consuming, we frequently have to estimate bed load transport rates by existing equations [12]. However, the evaluation of equation performance in gravel-bed rivers has been limited due to the small number of available data sets, and those assessments that have been made are discouraging, commonly reporting orders of magnitude error [13, 23]. It seems that despite over a century of research, scientists are unable to consistently and reliably predict bed load transport rates [12]. This is particularly difficult in gravel-bed rivers, where the presence of a coarse surface layer acts to constrain the availability and mobility of the finer subsurface bed material [4]. The derivation of bed load transport equations can be classified into three categories: (a) formulation based on advection, (b) formulation based on energy concept, and (c) graphical methods and empirical equations based on regression analysis (e.g., Peter Meyer and Muller's equation) probability concepts (e.g., Einstein) [17]. All existing bed-load equations invariably depend on calibration by data from laboratory experiments or field observations. Consequently, equations are applied to the conditions under which they are developed, and appreciable discrepancies between equations are observed [24]. During the past several years, there have been a number of studies focusing on the challenges of measuring and calculating the transport of bed load in streams with bed material consisting of sand and gravel [5, 6, 15]. Gomez and Church [13] performed an analysis of 12 bed load transport equations using 88 bed load transport observations from 4 natural gravel-bed rivers and 45 bed load transport observations from 3 flumes and concluded that none of the selected formulae performed consistently well. Researchers have made great strides to seek a suitable theory to characterize bed load transport. Two representative theories have been presented in literature: one was developed by Einstein, on the basis of probability theory, and the other was proposed by Bagnold [3, 10, 13] based on the energy conservation principle. In the literature on the selected methods, approaches and even the utilized dimensional and dimensionless parameters, there is no consensus among researchers on how to select the best representative equation, since each equation has its own limitations and the range of application within the selected data set [18, 20]. Barry et al. [4] found no consistent relationship between equation performance and the degree of equation calibration and complexity. They evaluated the performance of eight different equations of four common bed load transport equations, each of which are calibrated to some degree with site-specific data and vary in their complexity and difficulty of use. They found considerable differences in equation performance, there is no consistent relationship between performance and degree of formula calibration or complexity at the 24 Idaho sites. The depth distribution of gravel river bed due to bed-load transport was studied by DeVries [7]. Regression equations through a probabilistic approach which may not have any physical meaning relating to the mechanics of total-load transport equations are the pioneers of this approach, since they assumed that the beginning and cessation of sediment motion can be expressed in terms of probability [10, 22]. In fact, all applied equations to predict the bed load transport are modified by using empirical constants, showing there is no pure equation for sediment transport evaluation. For example, Bagnold's empirical bed load equation was a stream power correlation modified by an empirical scaling of depth and grain size [18]. Bagnold's failure to present the rational result was due to his failure to find the full dependence of the bed load transport on governing physical processes, even though that understanding had previously been reached by Einstein. Myer-Peter and Muller's equation is an empirical equation, using threshold parameters (Shields and critical Shields parameters) to predict bed load transport.In this study, a new data set is presented for the bed load transport for the first time in literature and using these measured data in two Iranian rivers, the effect of hydraulic and geometric parameters on the bed load transport is investigated by a linear method. Finally, the suggested linear equations are calibrated by using accurate laboratory data.
2. Material and Methods
2.1. Study Area
The study areas in this research are located in two regions of central and northern Iran in the basins of Caspian Sea and Zayandehroud. The Zayandehroud basin is located in southwest central part of Iran between (31° 30’ -33° 32’ N and 49°30’ -52° 49’ E). The Zayandehroud River, with a watershed of 27,100 km2 and average slope of 0.15%, was considered for sampling 9 cross-sections in 3 river reaches called Skandarie, Langan and Ghodjanak. The Zayandehroud starts in the Zagros Mountains and flows 400 kilometers (200 mi) eastward before ending in the Gavkhouni swamp, a seasonal salt lake, southeast of Isfahan city. The Zayandehroud River basin has an area of 41,500 square kilometers, an altitude from 3,974 meters to 1,466 meters, an average rainfall of 130 millimeters and a monthly average temperature of 3°C to 30°C. The flow of the river has been estimated at 1.2 cubic kilometers (0.29 cu mi) per annum, or 38 cubic meters (1,340 cu ft) per second. Figure 1 shows a view of the three selected river reaches of Zayandehroud in this study. Another area where this study was conducted is located in Caspian Sea basin in the north of Iran between 3716’ - 3822’N and 5355’ - 5548’E. The selected river is the Babolroud River which is one of the main rivers in the Caspian Sea basin. The area of Babolroud basin is 1746.42 km2, with an annual discharge 10 m3/s and an average slope of 0.74%, located between coordinates 3625’ northern latitude and 5242’ east latitude. Sampling was performed in 10 cross-sections in 3 reaches, with names as Kelarikolah, Daronkolah and Anarestan. Figure 2, shows a view of the three selected reaches of this river.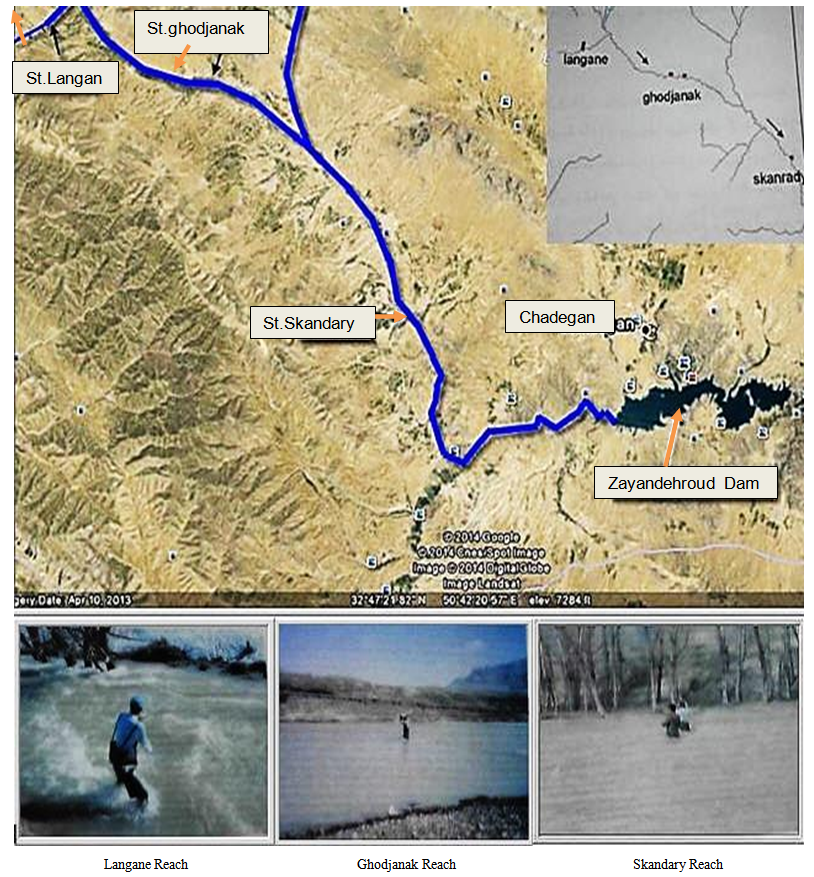 | Figure 1. View of the three river reaches of the Zayandehroud River |
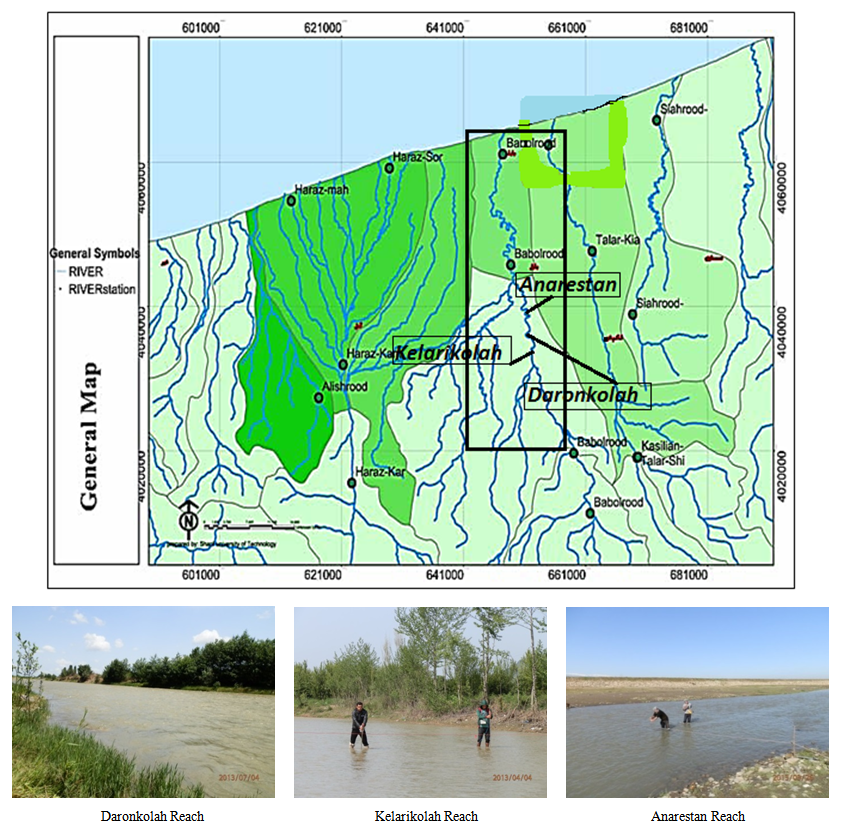 | Figure 2. View of the three selected river reaches of the Babolroud River |
2.2. Methods of Sampling and Analysis of Measured Data
It is recognized that the deployment of bed load sampler in the Babolroud and Zayandehroud Rivers is extremely difficult and potentially hazardous due to the large variations of depth and velocity, and floating debris that may be encountered. At each site, bed load, velocity, water slope, bed grain size were measured. In this study the bed load was measured by a Haley Smith sampler [16]. The Helley-Smith sampler weighing about 5 kg with an opening of 7.62×7.62 cm having a sediment collector bag made up of polyester with dimensions of 0.25 mm holes. Helley and Smith (1971) developed this method of handheld pressure difference bed load sampler with an intake nozzle of varying dimensions, a nylon mesh catchment bag attached to the rear of the nozzle, and a handle. The Helley-smith sampler gives a hydraulic efficiency between 0.9 to 1.1 for particles ranging from 0.5 to 16 mm as long as the extent of sampler fill is less than 30% [8]. Advantages of the Helley-smith sampler include portability and ease of using on an unaltered streambed. An example of Helley-Smith instrument is shown in Figure 3.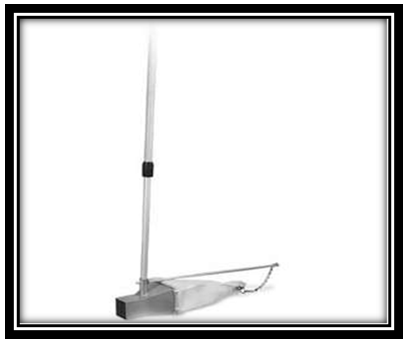 | Figure 3. Helley Smith sampler instrument |
After sampling sediment grains using a sieve, it was found that the median size of grain was 4.43 mm. The bed load discharges of the Babolroud and Zayandehroud rivers were calculated as 5.08 ton/day and 29.66 ton/day, respectively. Using a butterfly current meter on the horizontal axis, point velocity measurements were made in the vertical direction from the bed to the water surface. Each point velocity measurement was taken with three repeats at an interval of 50 s to get an accurate average value at that point. For the bed slope at each cross section, two cross sections were selected along a reach and the water surface was marked at both cross sections. The slope was determined by dividing the water surface difference by the distance between two cross sections. This slope value corresponded to the central axis of the channel in the selected reaches. The river bed material size was determined by Wolman's method at all locations. The average flow depth of each cross section was determined by dividing the cross sectional area (A) by the top width (T) as H = A/T. Flow discharge was determined by using a station hydraulic geometry measurements for each section. Accordingly, at first, flow velocity was measured at different parts of a section and then by measuring cross sectional area, flow discharge was estimated using the continuity equation. Shear velocity (u*) was calculated using the boundary-layer characteristics method (BLCM) using each velocity profile as follow [2]: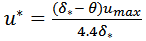 | (1) |
in which δ* (m) is the displacement thickness; ϴ(m) is the momentum thickness and umax (m/s) is maximum velocity observed in a velocity profile; these thicknesses are defined as [2]: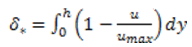 | (2) |
 | (3) |
where h is the local flow depth at each cross section which is different from hydraulic depth (H). Since the collection of bed load data is very expensive, risky, and time consuming, there are few papers in literature which present these data. The authors carried out a research to find bed load data to use in this study, finding Graf and Suszka’s data set [14]. Graf and Suszka presented their bed load data for relatively steep slopes with high precision. These data set are used to calibrate the linear model suggested in our study. A summary of calculations of various parameters, such as hydraulic flow depth (H), flow (Q) and bed load discharges (Q’b), grain size (D50 and D90), and cross sectional shear velocity (U*) is presented in table 1. The field data were collected in this study and laboratory data were used from Graf and Suszka’s paper [14].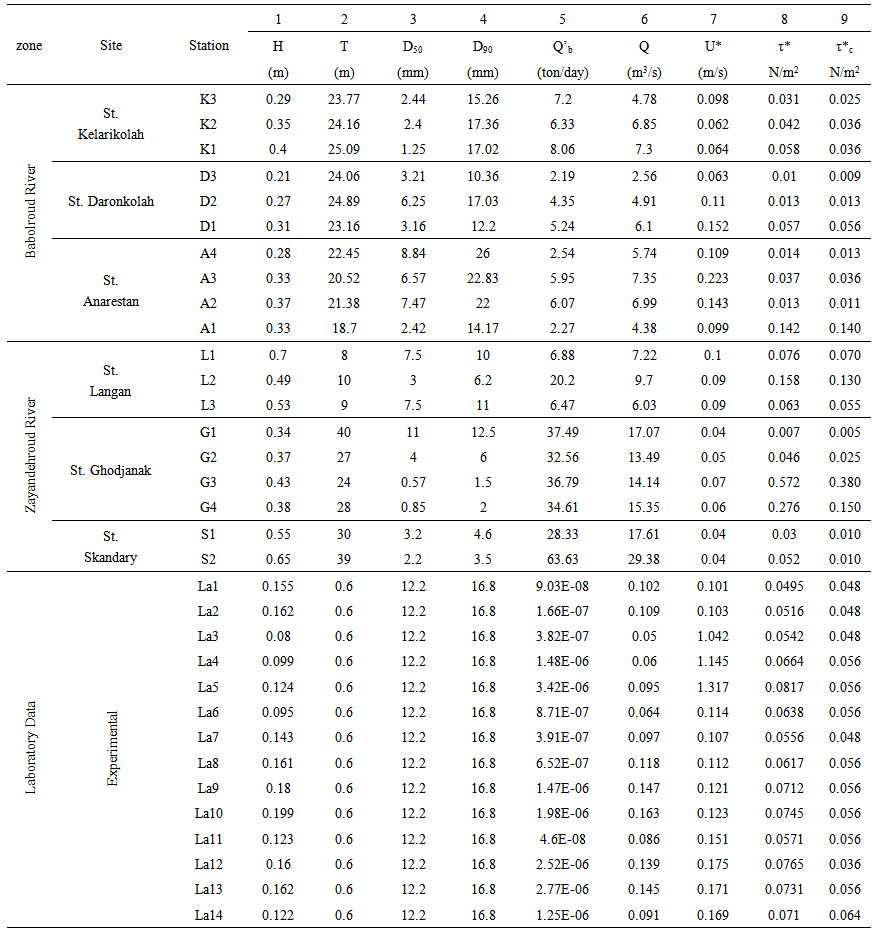 | Table 1. Computational parameters for data collected |
Information Table 1:h = the average depth of each section (m), T = the width of the water surface (m), D50 = the median diameter of bed load particles (mm) and D90 = the grain diameter at which 90% of the sediment sample is finer than, Q’b = the bed load transport rate (ton/day), Q = the flow discharge (m3/s), and u* = the equivalent cross-sectional shear velocity. τ* = the Shields parameter which is written as follows: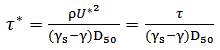 | (4) |
where ρ is the density of water, ɣs and ɣ are specific weights of sediment and water, respectively.Using the trial and error method the Meyer-Peter Muller's equation is approximated as: | (5) |
qb=Bed load discharge per unit width (ton/day/m), τ*c = the critical Shields parameter, SG = specific gravity (ρs/ρ) in which ρs is the sediment density, ρ is water density, and g = gravitational acceleration.Meyer-Peter Muller's equation is purely empirical one and should be applied for large sediment grain and wide channels (i.e. large aspect ratio T/H). The same thing is true for Einstein's equation. Engelund and Hansen [11] revealed that Einstein's bed load equation differ considerably from experimental data for large amounts of bed load transport. Accordingly, the empirical equations should not be applied outside of their domain of validity.
2.3. Empirical Equations for Bed Load Transport
To investigate the effect of hydraulic and geometric parameters on the bed load transport rate, a number of hydraulic and geometric parameters, such as average velocity, shear stress, hydraulic depth, water slope, and sediment particle diameter, were applied in dimensionless forms. The ability of prediction of the empirical equations was investigated by the measured data. Dimensionless hydraulic parameters are the Froude number (Fr), the relative Shields parameter (τ*/ τ*c ), and the shape factor (T/H). The dimensional geometric parameters are the particle parameter (D*), which is calculated from the following equation [21]: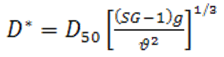 | (6) |
Where SG is specific gravity (ρs/ρ), ρs is sediment density and ρ is water density, g is gravitational acceleration and ν is the fluid kinematic viscosity. Table 2 presents the dimensionless parameters used for multiple regression in this study. 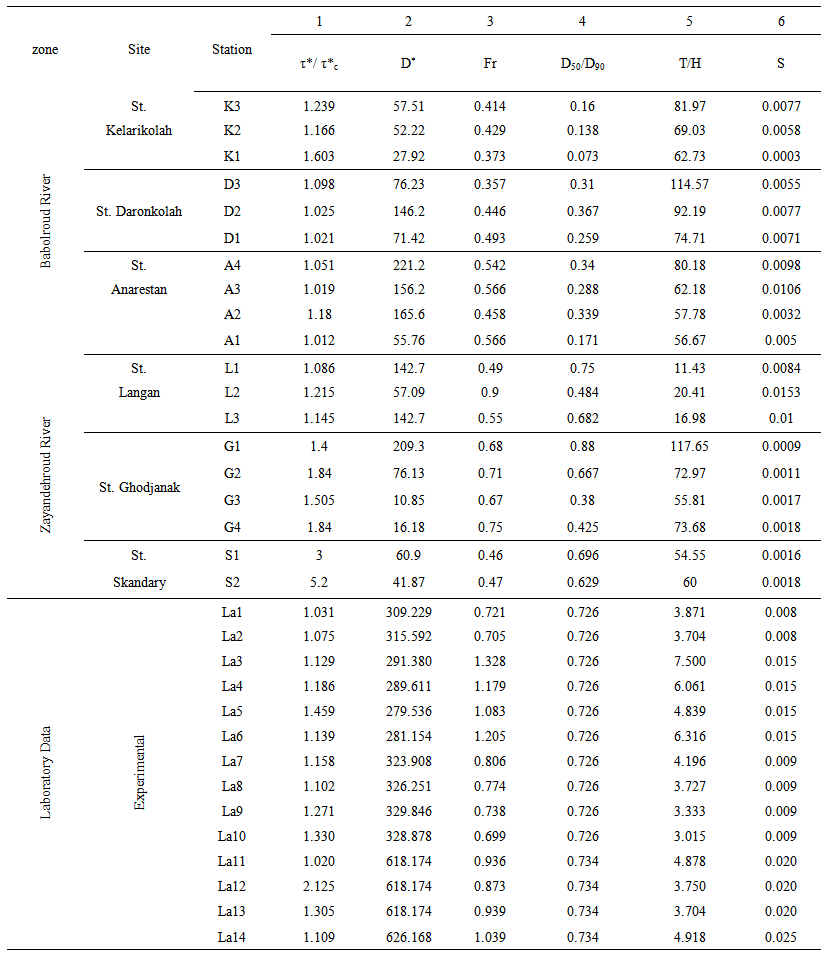 | Table 2. Dimensionless parameters appropriate for multiple regression |
Eighty percent of data from 33 cross-sections of the two rivers and laboratory were employed to develop the empirical equations and twenty percent of data were considered to calibrate these equations. Using the Kolmogorov – Smirnov test in the SAS software, the normality of data was confirmed. Two dimensionless equations were derived for this study: equation (7) using hydraulic parameters and equation (8) using geometric parameters: | (7) |
 | (8) |
In Equations 4 and 5 bed load rates (Qb) is equal: | (9) |
The reason for presenting the bed load equations in terms of dimensionless parameters is to keep generality, therefore, the equations and results are transferable across a range of scales. Statistical benchmarks that confirm the validity of equations (7) and (8) in the SAS software were calculated, as shown in Table 3. In this table, the Durbin - Watson (DW) statistic shows the independence of errors that should be in the range of 1.5 to 2.5. Also by other criteria, such as R2, RMSE, CV (coefficient of variation), the error ranges were acceptable. | Table 3. Statistical benchmarks to confirm relations extracted from SAS software for 80% of data |
Table 3, presents a summary of statistical analysis of equations (7) and (8). This table shows that the contributions of the relative Shields parameter (τ*/ τ*c), the Froude number (Fr) and the shape factor (T/H) on the bed load evaluation. Some pioneer researchers (e.g., Meyer-Peter and Muller) used the Shields parameter and its critical value in their equations, however, the contribution of Froude number and the shape factor is novel. Using 280 gravel-bed rivers from different regions of the world, Afzalimehr and Anctil [1] found that the three parameters of (τ*/ τ*c), Fr and T/H in equation (7) are among the controlling parameters to estimate flow resistance. Application of these parameters to predict bed load transport by using a linear equation (7) with high coefficient of determination (R2 = 0.75) reveals that these parameters are also suitable to formulate bed load motion.Figure 4, compares the predictions of equations (7) and (8) with measured data. Accordingly, both hydraulic and geometric parameters play important roles in the bed load prediction, showing the coefficient of determination (R2) more than 60% (see table 4). Furthermore, most of used data in this study are located within the range of ± 100 % which is considered as reasonable range for bed load transport studies [21].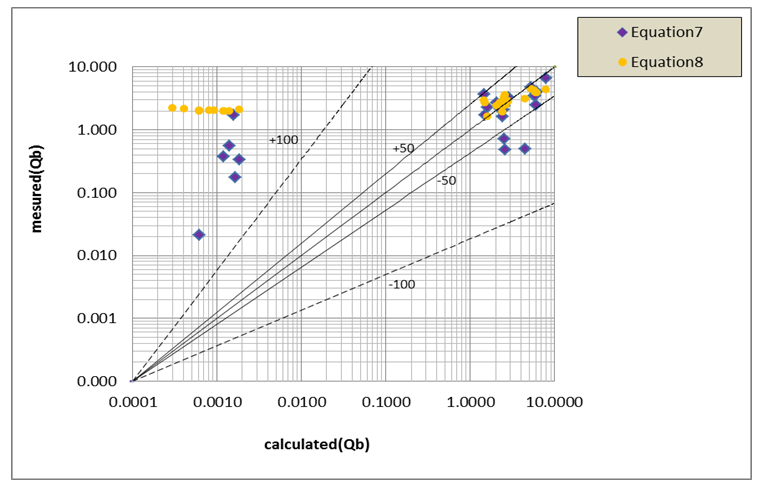 | Figure 4. The comparison of measured Qb and computed bed load |
 | Table 4. Benchmarks of statistical criteria calculated for 20% of the test data |
3. Conclusions
The following conclusions are drawn from this study:1- Considering no consistent relationship between the performance and degree of equation calibration or complexity in the existing data in the literature, a linear function seems (Equations 7 and 8) sufficient to describe our data rather than fitting by a power function as suggested by other studies [4]. The results of this study will be especially useful for designers and planners in mountainous regions, where large slopes and small relative depths are frequently encountered. 2- Results show that calibration and validation of both hydraulic and geometric parameters play important roles in the bed load prediction, with a coefficient of determination (R2) more than 60%. 3- Hydraulic parameters-based regression equations are more accurate than the geometric parameters-based regression equation. 4- The Shields parameter, the shape factor and the Froude number are among the controlling hydraulic parameters of bed load transport, showing a coefficient determination R2 = 0.75 for developed equation in table 3 and R2 = 0.87 for calibrated data in table 4. On the other hand, grain size distribution and the water slope play a significant role as geometric parameters of sediment transport, showing the coefficient determination of more than 55 percent.5- The available data do not allow to present any confident equation of bed load transport for a wide range of flow and morphologic conditions based on physical concepts due to weak correlation between physical process and sediment transport evaluation, showing great uncertainly on the prediction of bed load transport. The overall inaccuracy may not be less than a factor 2 as had stated by Van Rijn [21]. More bed load data are required to collect in coarse-bedded rivers in order to generalize the findings of this study.
List of Symbols
u*: Minor shear velocity (m/s)umax : Maximum velocity observed in a velocity profile (m/s)δ*: Displacement thickness (m)ϴ: Momentum thickness (m)h: Local flow depth at each cross sectionH: Hydraulic depth at each cross section (m)T: Width of the water surface (m)D50: Median diameter of bed load particles (mm)D90: Grain diameter at which 90% of the sediment sample is finer than (mm)Q’b: Bed load transport rate (ton/day)Q: Flow discharge (m3/s)U*: Equivalent cross-sectional shear velocity (m/s)
A: Total area of the cross-section
: Area between the axisτ*: Shields parameter (N/m2)τ: Shear stress (N/m2)ρ: Water density (kg/m3)ρs : Sediment density (kg/m3)ɣs : Specific weights of sediment (N/m3)ɣ: Specific weights of water (N/m3)qb: Bed load discharge per unit width (ton/day/m)τ*c: Critical Shields parameter (N/m2)SG: Specific gravityg: Gravitational acceleration (m/s2)
: Fluid kinematic viscosity (m2/s)S: Bed slope (m/m)D50/D90: Parameter related to the ratio of particle diameters Fr: Froude number τ*/ τ*c : Relative shields parameter T/H: Shape factor D*: particle parameterQb1: Bed load discharge for hydraulic parameters (ton/day)Qb2: Bed load discharge for geometric parameters (ton/day)DW: Durbin – WatsonR2: Correlation or (R) SquareRMSE: Root Mean Square ErrorCV: Coefficient of VariationMAE: Mean Absolute Error
References
[1] | H. Afzalimehr and F. Anctil “Estimation of Gravel-bed River flow resistance”. J. Hydr. Eng, ASCE, 124(10);1054-1058, 1998. |
[2] | H. Afzalimehr and F. Anctil, “Accelerating shear velocity in gravel-bed channels”. Journal of Hydrological Sciences, 1: 34-47, 2000. |
[3] | R. A. Bagnold, “An empirical correlation of bed load transport rates in flumes and natural river.” Proc. R. Soc. London, Ser. A, 372, 453-473, 1980. |
[4] | J. J. Barry, J. M. Buffington and J. G. King, “A general power equation for predicting bed load transport rates in gravel bed rivers”. Water Resour Res,. 40, W104001,DOI:10.1029/WR003190, 2004. |
[5] | K. Bunte, K, S.R. Potyondy and J. P. Ryan, “Measurement of coarse gravel and cobble transport using portable bed load traps”. J. Hydr. Eng, ASCE,130 (9): 879-893, 2004. |
[6] | N. Claude, S. Rodrigues,V. Bustillo, J. G. Breheret, J. J. Macaire and P. Juge, “Estimating bed load transport in a large sand-gravel bed river from direct sampling, dune tracking and empirical formulas”. Geomorphology. 179: 40-57, 2012. |
[7] | P. DeVries, “Bed-load layer thickness and disturbance depth in gravel bed streams”. J. Hydr. Eng, ASCE,128(11): 983–991, 2002. |
[8] | W. W. Emmett, “A Field Calibration of the Sediment Trapping Characteristics of the Hellay-Smith Bed Load Sampler”. U.S. Geological Srvey Prof. pp:1139, 1980. |
[9] | W. W. Emmett and M. G. Wolman, “Effective discharge and gravel-bed rivers”. Earth. Surf. Proc. Landforms, 26:1369–1380, 2001. |
[10] | H. A. Einstein, “The bed load function for sediment transportation in open channel flows”. Technical Bulletin No. 1026, U.S. Dept. of Agriculture Soil Conservation Service, Washington. D.C, 1950. |
[11] | F. Engelund and E. A. Hansen, “Monograph on Sediment Transport in Alluvial Streams”. 3rd edn (Teknish Forlag: Copenhagen, Denmark), pages 62, 1972. |
[12] | B. Gomez, “The potential rate of bed load transport”. Proceedings, National Academy of Sciences,73(103):17170-17173, 2006. |
[13] | B. Gomez and M. Church, “An assessment of bed load sediment transport formulae for gravel bed rivers”. Water Resour. Res. 25: 1161-1186, 1989. |
[14] | W. H. Graf, and L. Suszka, L. “Sediment transport in step channels”. Journal of Hydroscience and Hydraulic Engineering, 5(1): 11-26, 1987. |
[15] | H. Habersack and J.B. Laronne, “Evaluation and improvement of bed load discharge formulas based on Helley-Smith sampling in an alpine gravel bed river”. J. Hydr. Eng, ASCE, 128 (5): 484-499, 2002. |
[16] | E.J. Halley, W. Smith, “Development and calibration of a pressure difference bed load sampler” U.S. Geol. Surv. Open File Rep, 1971. |
[17] | P. Julien, “Erosion, and sedimentation” New York: Cambridge University Press, 2010. |
[18] | Y. Martin, “Evaluation of bed load transport formulae using field evidence from the Vedder River, British Columbia” Geomorphology, 53: 75–95, 2003. |
[19] | D.W. Reiser, “Sediment in gravel bed rivers: ecological and biological considerations, in Gravel Bed Rivers in the Environment”. ed. P.C. Klingeman, R.L, 1988. |
[20] | S. Sinnakaudan, A. Ghani Ahmad and N.A. Zakaria, “Multiple linear regression model for total bed material load prediction”. J. Hydr. Eng, ASCE, 132 (5): 521–528, 2006. |
[21] | L. C. Van Rijn, “Sediment transport, Part 1: Bed Load Transport”. J. Hydr. Eng, ASCE, 110(10):1431-1456, 1984. |
[22] | C.T. Yang, “Sediment Transport Theory and Practice”. McGraw-Hill, New York, 1996. |
[23] | C. T. Yang and C. Huang, “Applicability of sediment transport formulas”. Inter. J. Sediment. Res, 16: 335-353, 2001. |
[24] | D. Y. Zhong, G. Wang and L. Zhang, L. “A Bed-load function based on kinetic theory” Inter. J. Sediment. Res, 27: 460-472, 2012. |