Khudoykulova Shoira Narzullaevna
Samarkand State Medical University, Samarkand, Uzbekistan
Correspondence to: Khudoykulova Shoira Narzullaevna, Samarkand State Medical University, Samarkand, Uzbekistan.
Email: |  |
Copyright © 2024 The Author(s). Published by Scientific & Academic Publishing.
This work is licensed under the Creative Commons Attribution International License (CC BY).
http://creativecommons.org/licenses/by/4.0/

Abstract
The results of multifractal parameterization of digitized images of histological sections of the cornea of experimental animals with different psychotypes of behavior exposed to a rotating electric field for ten and twenty days to identify the response to stress are presented. Quantitative estimates of stress adaptation in experimental animals exposed to a rotating electric field were obtained based on generalized Renyi dimensions. The difference in generalized entropies gives a relative assessment of the dynamic state of the tissue structure of the cornea of an experimental animal based on a statistical description of its states in the “normal” state.
Keywords:
Psychotype, Adaptation, Multifractal ordering, Entropy, Self-organization, Stress
Cite this paper: Khudoykulova Shoira Narzullaevna, Influence of the Time of Exposure to a Rotating Electric Field on the Parameters of the Structure of Corneal Tissues of Animals with Different Prognostic Stress Resistance, International Journal of Genetic Engineering, Vol. 12 No. 5, 2024, pp. 62-67. doi: 10.5923/j.ijge.20241205.02.
1. Introduction
The development of methods and algorithms for recognizing structural changes in tissues under various physiological and pathological conditions on raster images of corneal sections is an urgent task both in scientific and applied terms in keratoconus [3,4]. The complexity of prognostic assessment of the functional state of corneal tissues on the verge of so-called norms and pathologies as a result of imbalance between the body and the environment is a multi-criteria task and requires to make a decision, consider the conditions for the optimality of the formation process and the properties of the Pareto distribution function of the elements of the fractal set of the corneal structure. The ability of the body to adapt to changes in the environment, to maintain its homeostasis in the event of adverse factors, including information influences, is important for the prevention of overstrain and exhaustion of adaptation mechanisms.Informational effects include biological effects caused by fields of "non-thermal" action, below the threshold of activation of protective biological mechanisms, therefore capable of accumulating at the subcellular level – the level of genetic processes [Oleynik V.P. Fundamentals of interaction of physical fields with biological objects. - Kharkov: National Aerospace University named after N.E. Zhukovsky "KhAI", 2006. -61p.Bingi V.N., Savin A.V. Physical problems of the action of weak magnetic fields on biological systems. UFN. 2003. -T.173, No3. P.265-300]. The issues of the influence of weak EMFs on living systems are still far from being solved [Rybakov Yu.M., dissertation, Doctor of Biological Sciences, General Impact on the Body of Weak Low-Frequency Vortex Magnetic in the Development of the Tumor Process. Moscow. 2013, 258 p.]The biological action of ultraweak agents (weak magnetic fields of B<1 mT and a frequency of 10-100 Hz) is a fundamental scientific problem of an acutely applied nature.The results of a study of the effect of a rotating electric field (REF) on the reproductive system of female rats noted structural changes in the placenta, fetal growth retardation, and an increase in the incidence of embryonic mortality [1]. The formation of biological reactions is facilitated by the processes occurring at different levels of the organization of the organism from molecular and cellular processes to physiological changes in the functional state of the organism as a whole, causing adaptive reactions of its regulatory systems. The results of the impact of REF were studied on the cornea of experimental animals, quantitative estimates of the animal's stress response were obtained, taking into account the psychotype of behavior [2]. Illarionov V.E. comprehensively considered the basics of information medicine within the framework of the use of EMF with various characteristics [Illarionov V.E. Magnetotherapy. Moscow: Librokom. 2009, 136 p.].Recognizing EMF as a universal carrier of bioinformation and applying the information theory developed for communication networks in living nature, the authors [Gerasimov I.G., Laptev et al. Electromagnetobiology and clinical experiment in physiology. Monograph. Moscow-Tver-Tula: Triada Publishing House, 2008, 160 p.]. come to an erroneous statement about a possible indirect channel of information transmission, which is to be determined. The author proposes to solve the issue of information transfer along with other unstudied issues in his innovative study of the information impact on the morpho-physiological and fractal parameters of the corneal structure. Control in cybernetics is based on the use of the main function of information - the removal of uncertainty of the state of the system. In nature, development is a spontaneous process accompanied by an increase in entropy and the transition of a biological system to an equilibrium state, according to the second law of thermodynamics, information increases accordingly, since an open biological system does not have any predetermined goals and objectives of development. Therefore, the behavior of an open biological system is nonlinear and is considered on the basis of synergetics and fractal geometry, identifying invariant manifolds and using them in synergetic models. Evolution in animate and inanimate nature proceeds on the basis of the law of hierarchical growth of entropy, the memorization of information in the structure is based on the static and dynamic stability of the elements of the structure [Khazen A.M. Introduction of the measure of information into the axiomatic basis of mechanics. (Second edition) Moscow: RAUB, 1998. 170 p.]. Based on the study of various physical and metric relations in isomorphic objects in the process of functioning, the formation of various static and dynamic structures is observed, which are necessary for their survival in a constantly changing environment [Rapoport A. "Mathematical Aspekt of General Systems analysis". General Systems, vol. XI, 1966. P.3-11.]. For the description of natural materials, multifractal formalism, statistical and information physics, and symmetry theory are promising [5-7]. The information remains virtually unchanged until the properties of the symmetry change. With the concept of entropy and information, theoretical ideas about the "order" and "organization" of complex systems in materials science are developing. [The multifractal parameterization methodology is a way of quantifying the observed structures of materials [9] and is described by the spectrum of generalized Rényi dimensions Dq [10]. According to the works of Y.L. Klimontovich [13], the normal functioning of an organism is possible only if there is a certain uncertainty in the choice of stable states by the system, quantitatively estimated by the "norm of chaos". Entropy is a macroscopic parameter that corresponds to the level of chaos in the system. The mathematical meaning of entropy from the works of K. Shannon [12], entropy is a measure of the uncertainty of a certain situation. additive value. Entropy estimate: H=∑ik (Pi∙log2Pi), where Pi is the probability of the i–th state of the K possible. In the case when all states are equally probable, the entropy of the system is maximal Hmax = log2K. For one state with a probability equal to 1, the entropy of the system is equal to zero. The transition from a less ordered to a more ordered state in the process of system self-organization is accompanied by a change in entropy from Hmax to zero.Biological systems are an example of chaotic systems that are self-organized, as a living organism self-organizes from a chromosome set in the womb. It is most relevant to differentiate the information-entropy spectra of information systems of the structure of corneal tissues of control and field-exposed experimental animals to determine the functional state of information systems among themselves, and for spectra to classify the manifestations of their large-scale invariances in order to identify and diagnose the functional state of the organism as a whole. Biologists see fractal scaling as a universal principle of morphogenesis, noting the importance of penetrating into the mechanisms of coding and reproduction of fractal models of biological objects [Gleick J. Chaos: the creation of a new science. St. Petersburg: Amphora, 2001. pp. 142-146.]. Within the framework of synergetics, Haken identified the principle of subordination, which allows complex chaotic processes to be described by a small number of independent variables describing fractal properties [Haken G. Information and Self-Organization. 1991.]. The determination of the spectrum of generalized Rényi dimensions allows the description of complexity and statistical properties based on multifractal parameterization of the image of the corneal structure.Objective. To identify the effect of the time of exposure to a rotating electric field on the parameters of the structure of corneal tissues of animals with different prognostic stress resistance and to obtain quantitative estimates.
2. Object and Method of Research
The object of the study was rats. With the help of the "Open Field" method at the Department of Normal Physiology of the Izhevsk State Medical Academy, animals were divided into three psychotypes: stable, unstable, ambivalent. Experiments were conducted on the effect of a rotating electric field changing according to the sinusoidal law with a frequency of 50 Hz on experimental animals for ten and twenty days was taken and recorded in accordance with the requirements of histological examinations. Optical images of histological sections of the cornea of experimental animals with different psychotypes of behavior were studied at x10 and x20 objective magnification. The degree of inhomogeneity and disorder of any system is described by a change in structural entropy: ∆S = ∆Sconf + ∆Sel + ∆Smagne, where ∆Sconf is a change in entropy due to transformations of the spatial arrangement of active elements in the structure, ∆Sel and ∆Smagne are associated with the electric and magnetic interactions occurring in the system.As the structure becomes more complex, there is an increase in entropy. As a result of multifractal parameterization of images of histological sections of the cornea, the spectrum of generalized Rényi dimensions Dq and the following parameters are determined:at q = 0, D0= lnN, D0 is the Hausdorff–Bezikovich dimension that characterizes a homogeneous fractal (the dimension of self-similarity), N is the number of states of the system;at q = 1, D1=–∑PilnPi (information entropy);at q = 2, D2 – correlation entropy;at q = ∞, D∞=ln(1/Pmax), Pmax is the relative representation of the dominant structural phase;at q = –∞, D–∞ = ln(1/Pmin), Pmin – relative representation of a rare defect;D1 – D∞ is a measure of the order of the set;D-∞ - D+∞ - can be considered as a measure of randomness. In the literature, D+40 and D–40 are considered as minimum and maximum sparsity at q=40.Non-equilibrium phase transitions and transient processes of adaptation in the structure are observed by effects of different scales, in the form of jumps in entropy D1 and information resonances (randomness) ∆D = D-40 – D+40 during information transformations. The active elements of the structure show stability of relatively small perturbations of the scale parameter q during information transformations.The conditional criterion of the relative degree of randomness of the state of an open dynamical system is determined in relation to the state of the system taken as "physical chaos", the degree of randomness of the generalized information entropy of the active elements of the structure of which is maximum. The state of "healthy" (control) experimental animals and their generalized entropy H0 = ∑D1i, where D1i- information entropy of the active element of the structure. Parameters of the structure of the tissues of experimental animals: generalized entropy H=∑D1i= ln K, where K is the information capacity of the system; the maximum value in bits of information entropy Hmax=log2K; configuration entropy H* caused by transformations of the spatial arrangement of the active elements of the structure; the reserve of information memory of the structure ∆H=H max-H*; the reserve of information memory of the structure ∆H/Hmax∙100%.
3. Results and Discussion
Table 1 shows the structural parameters of the corneal tissues of control animals. At different magnifications, scale levels of the structure are observed that are not correlated with each other, so control animals were used for each magnification.Table 1. Information-entropic parameters of corneal tissues in control animals  |
| |
|
In the control rat 3k10, with an increase of x10, a long-range order of interaction of the active elements of the structure is observed (no information resonances are observed with an increase in scale, in this regard, the configuration entropy is insignificant H*=0.57), the generalized entropy is H0=∑D1i=5.15. The reserve of information memory of the quality of the functional properties of the structure is 92%. With an increase of x20 in a control rat 6k20, the long-range order of interaction is observed with d=31 px, H0=∑D1i=6.95. The reserve of information memory of the quality of the functional properties of the structure is 67%.In the control rat 8k20, the long-range order of interaction of the active elements of the structure is not observed, H0 = ∑D1i=6.76. The reserve of information memory of the quality of the functional properties of the structure is 38%. To control changes in the structure of corneal tissues of experimental animals, the parameters of animals 3k10 and 6k20 were used. Table 2. Information-Entropy Parameters of Corneal Tissues of Animals with a Stable Psychotype, After 10 Days of HEP  |
| |
|
Table 3. Information-Entropy Parameters of Corneal Tissues of Animals with Unstable Psychotype, After 10 Days of VEP  |
| |
|
As a result of 10 days of exposure to a rotating electric field in a rat with an unstable psychotype, the generalized entropy ∑D1i=H at an increase in x20 is higher than at x10. For a rat with a stable psychotype, the inverse ratio of generalized entropies is observed. The stock of information memory practically does not change with increase.Table 4. Information-Entropy Parameters of Corneal Tissues of Animals with a Stable Psychotype, After 20 Days of VEP  |
| |
|
Table 5. Information-entropic parameters of corneal tissues of animals with unstable psychotype, after 20 days of HEP  |
| |
|
With an increase in x10, a decrease in the level of generalized entropy of a rat with a stable psychotype is observed as a result of an increase in the duration of field exposure from 8.2 to 6.65. With an increase in x20, the generalized entropy changes insignificantly with an increase in the duration of the field impact. There is a noticeable decrease in the stock of information memory from (93... 94%) to (0... 21%) as a result of field exposure.Table 6 shows estimates of the degree of randomness of the structure of corneal tissues in animals after field exposure, taking into account the psychotype. Adaptation to field action takes place in the process of dynamic self-organization, which leads to a decrease in entropy and an increase in orderliness (∆S≤0). The processes of thermodynamic self-organization are caused by the complication of the structure, adaptation is accompanied by an increase in the number of structural defects, while entropy increases (∆S‹0).Table 6. Change in the conditional degree of randomness H-H0 relative to the norm 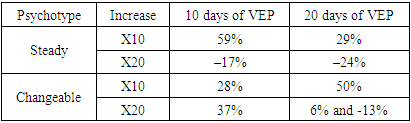 |
| |
|
With an increase of 10 in a rat with a stable psychotype, the criterion of the degree of randomness increases to 59% and decreases to 29%, which indicates degradation of the structure. With an increase of 20, the criterion decreases from -17% to -24%, which is also a deviation from the norm as a result of degradation (Table 7). For a rat with a stable psychotype, there is an increase in entropy ∆S >0 at the level of cell size d = 38... 41 pixels, D1=0.72... 0,73, Ks=(44… 49)∙10-3 at x10. After 20 days of HEP, degradation is observed at the levels of d=18..20, d=35... 41, d=43… 48, D1=0.62 and D1=0.56... 0.59. The parameter of level ordering is equal to Ks=(44... 49)∙10-3 and Ks=(70... 85)∙10-3.With an increase 10 in a rat with an unstable psychotype, the degree of randomness increases from 28% at 10 days of VEP to 50% at an increase in exposure to 20 days. In a rat with an unstable psychotype, after 10 days of exposure, adaptation occurs without degradation of the structural state of tissues in the process of dynamic self-organization, which is observed in Table 7 at x10. The degree of randomness with an increase of 20 decreases from 37% at 10 days of WEP to 6% and -13% with an increase in the duration of field exposure. With an increase of 10, degradation after 20 days of HEP is detected at the level of d=32..35pcs. and d=38... 43 pixels, D1=0.62... 0,63, Ks=(69… 96)∙10-3.Table 7. Analysis of adaptation processes based on the relationship between structural entropy D1 and orderliness Ks=D 1-D40 after 10 days of HEP, increase x10 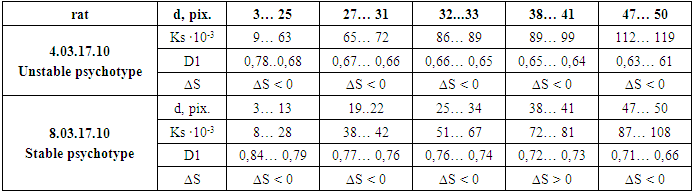 |
| |
|
Table 8. Analysis of adaptation processes based on the relationship between structural entropy D1 and orderliness Ks=D 1-D40 after 20 days of HEP, increase x10 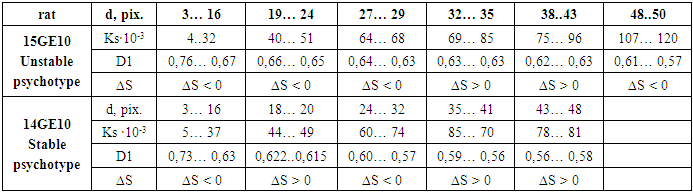 |
| |
|
Table 9. Analysis of adaptation processes based on the relationship between structural entropy D1 and orderliness Ks=D 1-D40 after 10 days of HEP, increase x20 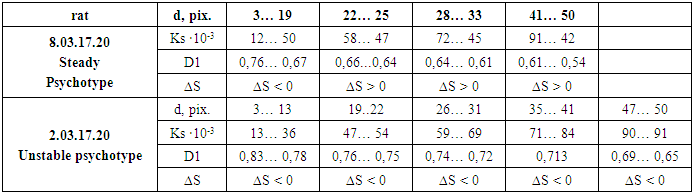 |
| |
|
Table 10. Analysis of adaptation processes based on the relationship between structural entropy D1 and orderliness Ks=D 1-D40 after 20 days of HEP, increase x20 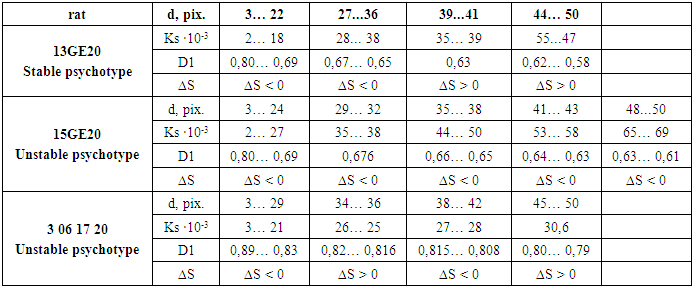 |
| |
|
At an increase of 20, degradation as a result of field exposure in a rat with a stable psychotype manifests itself at the levels of d=22..50 pcs., D1=0.54... 0,66, Ks=(42… 91)∙10-3 at 10 days of HEP. After 20 days of HEP, degradation is observed at levels d=41... 50, D1=0.58….0,63, Ks=(35… 55)∙10-3.In a rat with an unstable psychotype, an increase in x20 degradation does not reveal after 10 days of HEP. After 20 days of VEP, degradation is not observed in the animal 15GE20. In the animal on 3.06.17.20, after 20 days of VEP, degradation was detected at levels d=34... 36 pixels, d=45... 50 pixels, D1=0.79... 0,82, Ks= (25… 26)∙10-3 and Ks=(30.4... 30,6)∙10-3.
4. Conclusions
Quantitative estimates of stress adaptation of experimental animals exposed to a rotating electric field based on generalized Rényi dimensions have been obtained.The difference in generalized entropies gives a relative estimate of the dynamic state of the corneal tissue structure of an experimental animal on the basis of a statistical description of its "normal" states. There are three groups of stress response to field exposure:- entropy decreases, while order increases;- Entropy increases: insignificantly and abnormally compared to the "norm".Based on the analysis of changes in the relationship between informational entropy and the orderliness of the structure of corneal tissues in experimental animals, differences in structural changes in animals with different behavioral psychotypes are revealed: animals with a stable psychotype are less adapted to variability, uncertainty of external environmental parameters, compared to an unstable behavioral psychotype.
References
[1] | Zainaeva T. P., Egorkina S. B. Influence of the rotating electric field on the mother-placenta-fetus system in rats with different prognostic stress resistance. 2016. No 8. Pp. 3–7. |
[2] | Laptev D. S., Egorkina S. B., Stepanov V. A., Belykh V. V. Study of Structural Changes in Tissues under the Conditions of a Rotating Electric Field by the Method of Fractal Parameterization. 2019. Vol. 17, No 4, pp. 32-40. |
[3] | Indicators of the volume of corneal tissues in normal and keratoconus / A. N. Kulikov, E. V. Kudryashova, V. N. Gavrilyuk, D. S. Maltsev // Modern technologies in ophthalmology. 2019. Iss. No5 (30). P. 292. |
[4] | Abovyan A. A., Zilfyan A. A. Rol' matrixnoy metalloproteinase-9 v diagnostike keratoconus [The role of matrix metalloproteinase-9 in the diagnosis of keratoconus]. 2018. No 5. C. 263–265. |
[5] | Vstovsky G.V., Kolmakov A.G. Use of Information Theory Approaches in Condensed Matter Physics. Scientific. Conf. "Synergetics 2000. Self-Organizing Processes in Systems and Technologies", Komsomolsk-on-Amur, 2000, pp. 55-65. |
[6] | Vstovsky G.V., Kolmakov A.G., Bunin I.Zh. Introduction to Multifractal Parameterization of Material Structures., Izhevsk: "Regular and Chaotic Dynamics", 2001, 115 p. |
[7] | Vstovsky G.V. Elements of Information Physics.-Moscow: MGIU. 2002.-260 p. |
[8] | Belykh V.V., Muravyov V.V., Stepanov V.A. Application of the Generalized Pareto Distribution for Determining the Structural Parameters of Graphite in Cast Iron. Vestnik of Izhevsk State Technical University named after M.T. Kalashnikov. 2019. T. 22. № 1. Pp. 52-61. |
[9] | Ivannikov V.P., Stepanov V.A., Belykh V.V., Sufiyanov V.G. Fractal analysis of radiographs // Vestnik-IzhGTU. №3. 2009. – P. 150-154. |
[10] | Bozhokin S.V. Fractals and Multifractals / S.V. Bozhokin, D.A. Parshin.-Izhevsk: Research Center "Regular and Chaotic Dynamics".-2001.-128 p. |
[11] | Belykh V.V., Muravyov V.V., Stepanov V.A. Entropy assessment of the quality and resource of functional properties of the steel structure. 2020. № 3-4. (accepted for publication). |
[12] | Shenon K. Works on Information Theory and Cybernetics. Moscow, Foreign Literature Publ., 1963. 829 p. (In Russian) |
[13] | Klimontovich Y. L. Introduction to the Physics of Open Systems. Moscow, Janus-K Publ., 2002. 284 c. |