Jumanov Г. T., Abdukarimov A. O., Ablakulova N. A., Khojiboboeva S. H., Kushiev H. H.
Laboratory of Experimental Biology, Gulistan State University, Uzbekistan
Correspondence to: Abdukarimov A. O., Laboratory of Experimental Biology, Gulistan State University, Uzbekistan.
Email: |  |
Copyright © 2023 The Author(s). Published by Scientific & Academic Publishing.
This work is licensed under the Creative Commons Attribution International License (CC BY).
http://creativecommons.org/licenses/by/4.0/

Abstract
In this research work, genomic DNA from samples of 10 varieties of soft wheat was extracted by the STAB method. Quantitative and qualitative indicators of the isolated DNA were determined by spectrophotometric (Nootropic) and visual (gel electrophoresis) methods. PCR amplification was carried out on the basis of 10 pairs of SSR markers (Simple sequence repeat), in which isolated DNA samples were associated with wheat salt resistance genes. PCR products were analyzed by gel electrophoresis. The information structure of polymorphism(PIC) and heterozygosity(he) of the selected SSR markers were determined in the piccalc program. Based on the genetic polymorphism data obtained on the basis of SSR markers, a phylogenetic family tree of varieties was compiled using the UPGMA methodology with the help of Mega X program.
Keywords:
Soft wheat, Salinity, SSR markers, PCR, Phylogenetic family tree
Cite this paper: Jumanov Г. T., Abdukarimov A. O., Ablakulova N. A., Khojiboboeva S. H., Kushiev H. H., Molecular Genetic Evaluation of Wheat Varieties Based on SSR Markers, International Journal of Genetic Engineering, Vol. 11 No. 1, 2023, pp. 5-10. doi: 10.5923/j.ijge.20231101.02.
1. Introduction
Soft wheat (Triticum Aestivum L.)- one of the most widely grown and consumed food crops on earth. The choice of varieties suitable for soil and climatic conditions, resistant to stressors, allows you to get a high and high-quality harvest. Traditional methods require a lot of time and painstaking work when choosing varietal samples. The advantage of molecular genetic approaches to the creation of plant varieties in a short time and the selection of varieties resistant to conditions has been demonstrated by many researchers. On the other hand, the use of molecular genetic and biotechnological methods makes it possible to obtain relatively fast and accurate results, as well as to conduct a complete assessment of varieties and select samples suitable for soil and climatic conditions.Accordingly, today special attention is paid to the use of molecular genetic methods in the selection or creation of suitable conditions, productive wheat varieties to meet the needs of the population in food. To do this, it is necessary to identify the genes or QTL (Quantitative Trait Loci) loci associated with valuable farm traits and identify the DNA markers attached to them [1-3]. The application of DNA marker technology to practical selection is called MBS (marker-based selection). Using this method, the breeder will be able to direct the desired gene to the genotype of interest to him when introduced into any variety and, as a result, create a new variety, line or source resource that he wishes.The technology of barcoding (genetic passport) based on DNA markers serves to solve many problems existing in agriculture. Molecular identification of plant varieties of the genus of soft wheat (Triticum Aestivum) is an important factor in the successful management of germplasm resources and targeted breeding programs [4,5]. To date, hundreds of wheat varieties have been bred in our country, and it is not possible to fully differentiate them phenotypically. Although the created varieties differ in a number of characteristics, most of them look the same externally. This, in turn, creates various inconveniences for the authors of the variety and its breeders. In this aspect, it is important to create a molecular passport of wheat varieties, this technology allows you to study the variety of varieties, to identify inter-variety differences molecular.
2. Objects and Methods of the Research
The object of the research. Based on morphobiological characteristics, the domestic varieties Gazgan, Babur, Druzhba, Turkestan, Omad, Bunyodkor and varieties Antonina, Zvezda and Grekum created by breeders of the Russian state, widely cultivated in the grain fields of the Syrdarya region, as well as the Agro27 line created by scientists of the Kashkadarya Southern Research Institute of Agriculture, were selected as the object of research. The plant material was obtained from the collection of the Genbank of salt-resistant plants of the laboratory “Experimental Biology” of Gulistan State University.In order to define the genetic distinction (polimorfism) among the types of wheat altogether 10 pairs of SSR primers have been selected from the base TaSSRDb (triticum aestivum simple sequence data base) http://webtom.cabgrid.res.in/wheatssr/) (Table 1).Table 1. List of primers selected to identify genetic differences between wheat varietal samples 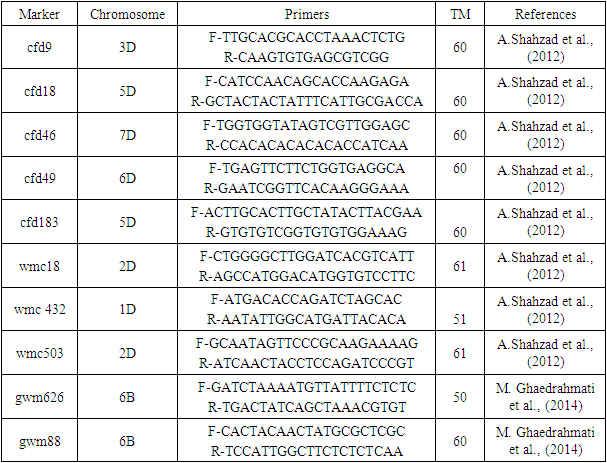 |
| |
|
These 10 selected SSR markers are markers associated with wheat salt resistance genes. 8 of them (cfd 9, cfd 18, cfd 46, cfd 49, cfd183, wmc18, wmc432 va wmc503) are markers associated with genes responsible for plant growth, root length, intensity of development, number of buds, weight of new shoots, salt resistance index. 2 (gwm88 and gwm626) of them are markers linking plant stems with their genes responsible for weight, as well as chlorophyll content [6,7].
3. Methods of the Research
DNA isolation. Young leaf tissues of the selected varieties were collected, and genomic DNA was isolated from them in the following order, based on a modified version of the STAB method [8]:1. Leaf samples collected from 100 mg- were stored in the refrigerator for at least 12 hours in - 20°C.2. The plant tissue was thoroughly crushed using a whisk with nitrogen liquid3. An extraction buffer of STAB 600 µl was added and thoroughly mixed4. Stored in the autoblot at 60-65°C for about 20-24 minutes (stirring every 10 minutes)5. 800 ml of chloroform / isoamyl alcohol is added in a ratio (24:1) (mixed manually until a homogeneous layer is formed), placed in a centrifuge at a speed of 12,000 a / min for 7 minutes to separate the aqueous and chloroform phases6. 600 ml are taken into a new 1.5 ml tube, chloroform / isoamyl (24: 1) is added from above in a ratio of 1:1 and placed in a centrifuge at a speed of 12,000 Ail/min for 7 minutes7. 600 µl is taken into a new 1.5 ml tube, 600 µl isopropanol is added, slowly stirred and left at 4°C8. The sample was centrifuged at a speed of 12,000 a/min for 10 minutes and isopropanol was carefully drained9. Added 800 µl of 75% ethanol10. Centrifugation at 12,000 rpm for 10 minutes11. Stage 10 was repeated for the 2nd time12. Air drying of sludge (drying equipment)then a 1/10-fold te buffer from 50 to 100 µl was added, depending on the size of the DNA (the tube can be turned upside down or made vortex to release DNA)13. Aged at room temperature for 1 hour14. RNase 1 ml (10 mg/ml) was added, then stored at 37°C for 30 minutes15. DNA was stored at 4°C for short-term use (per month) and at - 20°C for long-term use (in years).Spectrophotometric measurement. Advanced molecular biology techniques require clean and fast DNA extraction. Many of them have difficulty isolating DNA due to the high content of secondary metabolites, such as polysaccharides, polyphenols, in plant tissues. Phenolic compounds, as well as protein substances, prevent subsequent reactions (PCR) in molecular genetic studies. To carry out the PCR reaction on DNA samples extracted from plants, the optimal indicator is the concentration of DNA above 20 ng /ml, the purity index is 1.8-2.0 in the ratio of 260/280, 1.8-2.2 in the ratio of 260/230. In addition, the value of 260/280 above the established norm (up to 2.5) does not pose a problem for PCR [9].In the processes of nucleic acid extraction, residual chemical contamination can lead to an overestimation of the concentration of nucleic acids and/or negatively affect the subsequent analysis. The spectrophotometric analysis of DNA evaluates its concentration and degree of purity. When assessing the level of DNA purity, the A260 / a280 ratio was used to determine the degree of protein contamination. Also, the A260 / A230 ratio is used to determine the degree of contamination with other organic substances (chemicals used for extraction and secondary plant metabolites).In the spectrophotometric analysis of DNA, the ratio of concentration, purity (a260/a280 ratio) and absorption (a260/A230 ratio) was measured using a nanodropone c spectrophotometer (Thermo Scientific, USA), taking 2 µl from each sample.After spectrophotometric measurement, genomic DNA extracted from each sample was examined by electrophoresis in 0.9% agarosagel. The concentrations of the isolated genomic DNA of the studied samples were determined using the equipment of the Quant-c Transluminator (Helicon) by comparing (visually) with the DNA λ fagi, which had a certain concentration. Their concentration was brought to the working concentration (25 ng / ml) and stored frozen in a freezer at -20°C until further molecular studies.PСR analysis. The PСR reaction (hot-start software) was carried out in a volume of 10 µl in the following order. 1 µl contains MgCl2 containing 10xpzr buffers, 0.2 µl BSA (bovine serum albumin), 0.08 µl 25 µm mixture of dNTP (dATP, dGTP, dTTP and dCTP), 0.6 µl direct (F) and reverse (R) primers, 0.2 µl DNA polymerase Taq, 1 µl DNA matrix, 6.32 µl distilled water. The amplification process was carried out on Miniamplus Thermal Cycler equipment (Thermofisher, the USA) in the following time and temperature modes:- initial denaturation 5 min at 94°C; denaturation 45 sec at 94°C, 40 cycles; annealing (depending on the melting temperature of soils) 45 sec at ± 55°C; elongation 2 min at 72°C; final elongation 10 min at 72°C;The amplification products were separated horizontally at a voltage of 100 V (40 minutes) in a 2.5% agarose gel (1xtris-borate EDTA buffer) loaded with ethidium bromide. The DNA molecular weight marker (DNA ladder hyperladder 50 BP) was used to determine the size of the amplified fragment. Amplified DNA lines were visually examined and photographed using Quantum c (Helicon) Transluminator equipment.Genotyping. Using the MS Excel computer soaftware, SSR symbols were evaluated as the presence (1) or absence (0) of reinforced bands [10]. The studied wheat samples were labeled based on differences in the size of alleles of each locus of the SSR marker (Table 2). A special GelAnalyzer program was used for genotyping the results obtained.Table 2. Molecular differences (polymorphism) between the parental genotypes of the cfd49 SSR marker 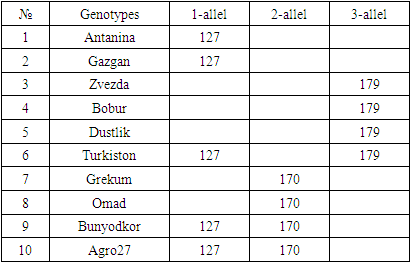 |
| |
|
4. Obtained Results and Their Analysis
When measuring the quantitative and qualitative parameters of DNA molecules using a nanodropic spectrophotometer, the DNA concentration averaged 1757.5 NG/µl. Also, the A260/A280 coefficient showed an average value of 2.04, and the A260/A230 coefficient showed an average value of 1.86 (Table 3).Table 3. Results of spectrophotometric analysis of DNA samples 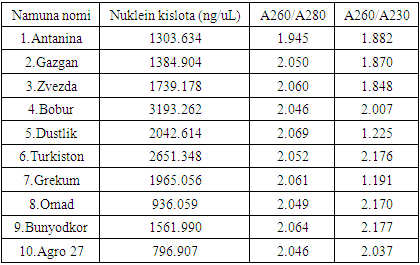 |
| |
|
The amount of total genomic DNA isolated gave 0.9% absorption during electrophoresis in agarose gel and examination in ultraviolet light on Quantum C Transluminator equipment (Fig.1).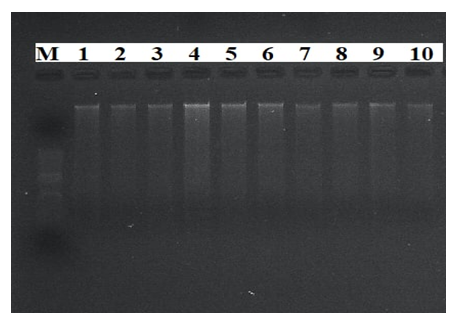 | Figure 1. Visual image of DNA molecules in 0.9% agarose gel. M-molecular weight marker (Hyper leader 50 bp), 1-10-genomic DNA isolated from experimental plants; 1) Antanina, 2) Gazgan, 3) Zvezda, 4) Bobur, 5) Dustlik, 6) Turkiston, 7) Grekum, 8) Omad, 9) Bunyodkor, 10) Agro 27 |
The analysis of PCR products by gel electrophoresis revealed high polymorphism of SSR markers cfd 9 and cfd18. Of the 10 SSR markers selected for the study, 9 showed polymorphism (Fig.2). Among the markers selected for the study, the cfd183 marker was identified as monomorphic (no molecular discrepancies were observed between the study samples) (Fig.3).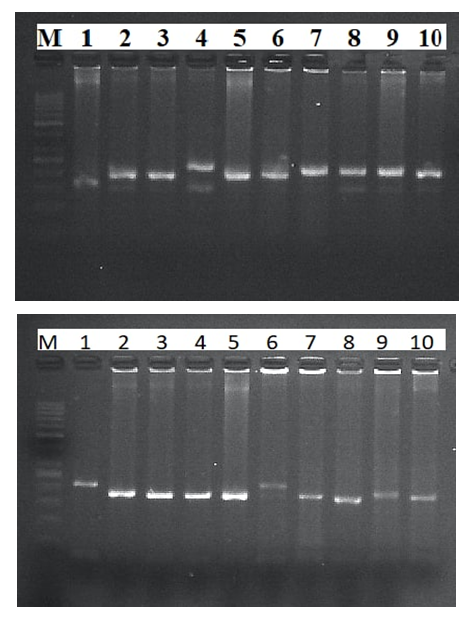 | Figure 2. PCR analysis of the study samples polymorphic marker cfd9, as well as electrophoregram of microsatellite markers wmc432. M-molecular weight marker (Hyper leader 50 bp), 1-10-genomic DNA isolated from experimental plants; 1) Antanina, 2) Gazgan, 3) Zvezda, 4) Bobur, 5) Dustlik, 6) Turkiston, 7) Grekum, 8) Omad, 9) Bunyodkor, 10) Agro 27 |
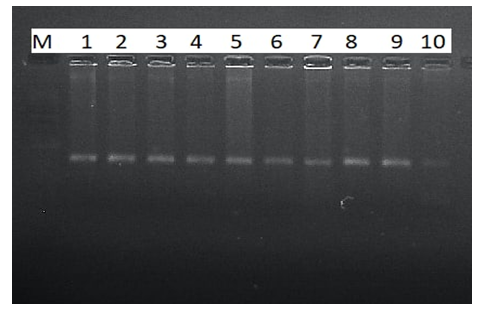 | Figure 3. PCR analysis of the study samples electrophoregram of the microsatellite marker of the monomer cfd183. M-molecular weight marker (Hyper leader 50 bp), 1-10-genomic DNA isolated from experimental plants; 1) Antanina, 2) Gazgan, 3) Zvezda, 4) Bobur, 5) Dustlik, 6) Turkiston, 7) Grekum, 8) Omad, 9)Bunyodkor, 10) Agro 27 |
These identified polymorphic SSR markers will be used in further studies for molecular mapping to identify genes responsible for resistance to salinization of winter wheat.SSR marking polymorphism indicators were evaluated by using the PICCalc program [11,12] and calculated using the Liu method [13] (Table 4).Table 4. Number of SSR marker alleles, information structure of heterozygosity and polymorphism 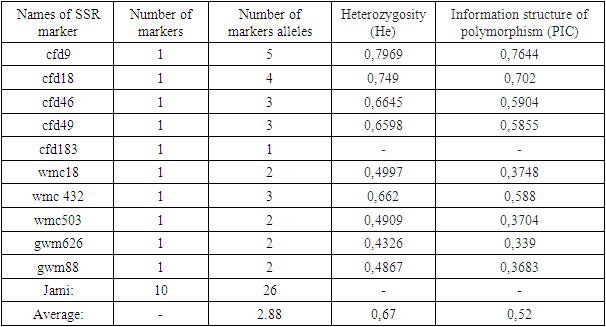 |
| |
|
The PIC value can be used to assess the degree of gene change in a plant. If the PIC value is > 0.5, the marker location is considered highly differentiated, and if PIC<0.25, the locus is considered low-differentiated [11,12]. The values of polymorphism data (PIC) of the analyzed microsatellite markers ranged from 34 to 76%, with an average of 52%. Among the 10 SSR markers used in this study, the cfd9 primer has the highest pic value of 0.76 pic. According to the PIC values of each marker; the lowest pic value was observed in the gwm626 primer with a PIC of 0.34.The most commonly used criterion for checking genetic variability in a population is heterozygosity [14]. The indicators of heterozygosity (He) were close to the values of PIC. It was found that the highest he value was observed in the cfd9 primer with a value of 0.79, and the lowest he value was observed in the gwm626 primer with a value of 0.43. In this study, the mean value of heterozygosity (he) using SSR markers was 0.67, and the range was from 0.43 to 0.79. Thus, 9 primers used in this study turned out to be highly informative. The results obtained using SSRIs are potential markers that can be used as markers in the selection of genotypes of resistance to salt stress due to molecular plant breeding.Using the genetic polymorphism data obtained on the basis of SSR markers, a phylogenetic family tree of varietal wheat samples was compiled by the Mega X computer program using the UPGMA method (Fig.4).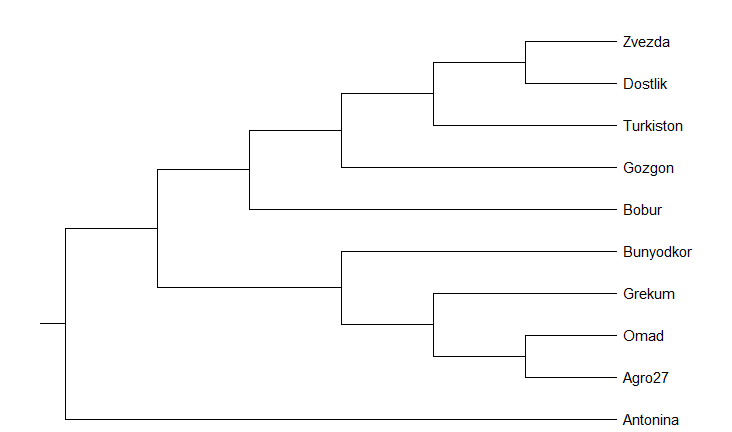 | Figure 4. Phylogenetic family tree based on polymorphism among SSR polymorphic markers |
According to the results of the analysis, the Antonin variety was isolated into a separate cluster, which means that it is genetically different from other genotypes. The second cluster was also divided into 2 groups, and the genotypes Agro 27, Omad, Grekum and Bunyodkor formed a separate group. The genotypes of Babur, Gaza, Turkestan, friendship and the star made up the second group. According to the analysis in the dendrogram, the genotype closest to the salt-resistant friendship variety is the Zvezda variety, and the genotype, the longest, is the Antonina variety. The proximity of the Zvezda, Babur, Gazgan and Turkestan varieties to the Druzhba variety, resistant to salinization, among the wheat genotypes selected for research work, testifies to the common origin of these genotypes.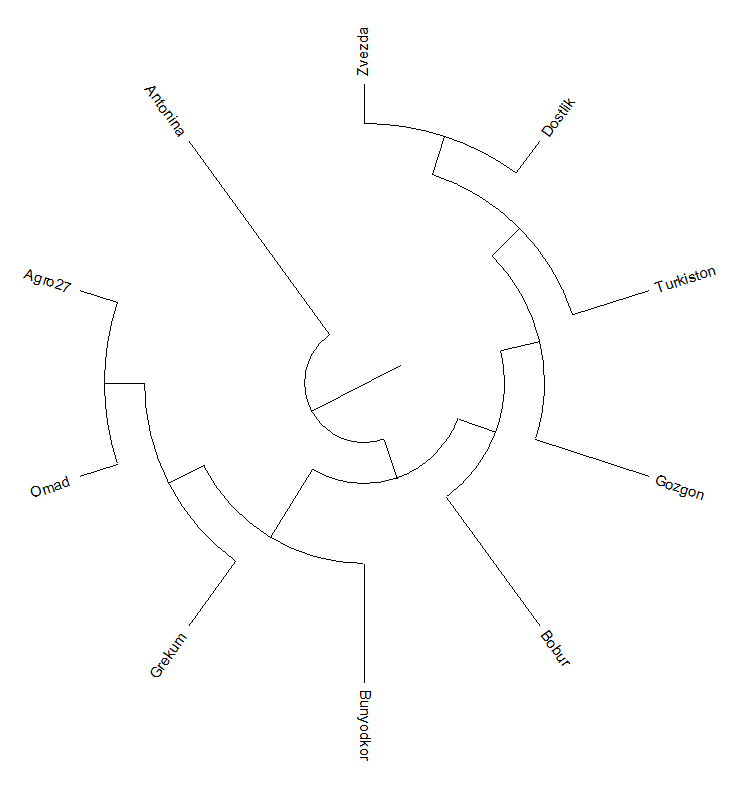 | Figure 5. Phylogenetic family tree based on polymorphism among SSR polymorphic markers |
DNA barcoding. After the PCR and genotyping phase of the study, barcoding based on cross-genetic polymorphism data of samples was performed in the Tec-IT Barcode Studio 16.3 computer program [15,16]:1. Name of the variety -Antanina. Genetic passport: A2, B4, D1, E2, F3, I22. Name of the variety -Gazgan. Genetic passport: A1, A3, B3, C2, D1, E2, F2, H1, H2, I13. Name of the variety -Zvezda. Genetic passport: A3, B2, C2, D3, E2, F2, G2, H2, I24. Name of the variety -Bobur. Genetic passport: A1, A5, B1, C3, D3, E2, F2, G1, H2, I15. Name of the variety -Dustlik. Genetic passport: A3, B2, C2, D1, D3, E2, F2, G1, G2, H1, H2, I26. Name of the variety -Turkiston. Genetic passport: A3, B1, C2, D3, E2, F3, G1, H1, H2, I27. Name of the variety -Grekum. Genetic passport: A4, B1, C2, D2, E1, F2, G1, H1, H2, I28. Name of the variety -Omad. Genetic passport: A1, A4, B1, C2, D2, E1, F1, G1, H1, H2, I29. Name of the variety -Bunyodkor. Genetic passport: A1, A4, B1, C1, D1, D2, E2, F2, G1, H1, H2, I110. Name of the variety -Agro27. Genetic passport: A1, A4, B1, C2, D1, D2, E2, F1, G1, H1, H2, I2
5. Conclusions
Of the 10 SSR (Simple sequence repeat) markers associated with wheat salt resistance markers, 9 had genetic differences between the study materials. These identified polymorphic SSR markers will be used in further studies for molecular mapping to identify genes responsible for resistance to salinization of winter wheat. Based on the obtained data of genetic polymorphism, a phylogenetic family tree of 10 varieties of wheat samples was compiled. According to phylogenetic analysis, the proximity of the varieties Zvezda, Babur, Gazgan and Turkestan to the variety Druzhba, resistant to salinization, among the selected wheat genotypes, indicates the common origin of these genotypes.
References
[1] | Huang XQ, Cöster H, Ganal MW, Röder MS (2003) Advanced backcross QTL analysis for the identification of quantitative trait loci alleles from wild relatives of wheat (Triticum aestivum L.). Theor Appl Genet 106:1379–1389. |
[2] | Ražná K1, Ablakulova N, Žiarovská J, Kyseľ M, Cagáň Ľ, Khojiboboevich KK and Bakievich GM, The Effect of Seed-Priming by Cobalt-Diglycyrrhizinate on Wheat (Triticum Aestivum L.) Genome, Open Acces Journal of Agricultural Research ISSN: 2474-8846, pp. 3-4. |
[3] | Katarína Ražná, Nodira Ablakulova, Jana Žiarovská, Matúš Kyseľ, Khabibjhan K. Kushiev, Maxmudjhan B. Gafurov & Ľudovít Cagáň, Molecular characterization of the effect of plant-based elicitor using microRNAs markers in wheat genome, Institute of Molecular Biology, Slovak Academy of Sciences, June 2020 Biologia 75(12), pp. 2-3. |
[4] | W John Kress 1, David L Erickson DNA barcodes: methods and protocols Methods Mol Biol. 2012; 858: 3-8. doi: 10.1007/978-1-61779-591-6_1. |
[5] | Shneer VS. [DNA barcoding of animal and plant species as an approach for their molecular identification and describing of diversity].. Zh Obshch Biol. 2009. PMID: 19799325 Review. Russian. |
[6] | A. Shahzad, M. Ahmad, M. Iqbal, Ahmed, and G.M. Ali. Evaluation of wheat landrace genotypes for salinity tolerance at vegetative stage by using morphological and molecular markers Article in Genetics and molecular research: GMR· March 2012 DOI: 10.4238/2012.March.19.2. |
[7] | M Ghaedrahmati and et all Mapping QTLs Associated with Salt Tolerance Related Traits in Seedling Stage of Wheat (Triticum aestivumL) November 2014 Journal of Agricultural Science and Technology 16(6): 1413-1428. |
[8] | J. J. Doyle and J. L. Doyle, “Isolation of Plant DNA from Fresh Tissue,” Focus, Vol. 12, No. 1, 1990, pp. 13-15. |
[9] | William W. Wilfinger, Karol Mackey, and Piotr Chomczynski, Effect of pH and Ionic Strength on the Spectrophotometric Assessment of Nucleic Acid Purity: BioTechniques 22: 474-481 (March 1997). |
[10] | Nei M, Li WH. Mathematical model for studying variation in terms of restriction endonucleases. Proc Natl Acad Sci. 1979; 76: 5269–5273. doi: 10.1073/pnas.76.10.5269. [PMC free article] [PubMed] [CrossRef] [Google Scholar] |
[11] | Botstein D, White R, Skolnick M, Davis R. Construction of a genetic linkage map in man using restriction fragment length polymorphisms. Am J Hum Genet. 1980; 32: 314–331. [PMC free article] [PubMed] [Google Scholar] |
[12] | Nagy S, Poczai P, Cernák I, Gorji AM, Hegedűs G, Taller J. PICcalc: an online program to calculate polymorphic information content for molecular genetic studies. Biochem Genet. 2012; 50(9–10): 670–672. doi: 10.1007/s10528-012-9509-1. [PubMed] [CrossRef] [Google Scholar] |
[13] | Liu B H. Statistical genomics: linkage, mapping and QTL analysis. Boca Raton: CRC Press; 1997. [Google Scholar] |
[14] | Jaccard P. Nouvelles research sur la distribution florare. Bull De Lasociete Vaudoise Des Sciences Naturelles. 1908; 44: 223–270. [Google Scholar] [Ref list] |
[15] | W John Kress 1, David L Erickson DNA barcodes: methods and protocols Methods Mol Biol. 2012; 858: 3-8. doi: 10.1007/978-1-61779-591-6_1. |
[16] | Shneer VS. [DNA barcoding of animal and plant species as an approach for their molecular identification and describing of diversity]. Zh Obshch Biol. 2009. PMID: 19799325 Review. Russian. |