K. S. Wanjala1, W. K. Njoroge1, J. M. Ngaruiya2
1Department of Physics, Kenyatta University, Nairobi, Kenya
2Department of Physics, Jomo Kenyatta University of Agriculture and Technology, Nairobi, Kenya
Correspondence to: K. S. Wanjala, Department of Physics, Kenyatta University, Nairobi, Kenya.
Email: |  |
Copyright © 2016 Scientific & Academic Publishing. All Rights Reserved.
This work is licensed under the Creative Commons Attribution International License (CC BY).
http://creativecommons.org/licenses/by/4.0/

Abstract
Tin Doped Zinc Sulphide (ZnS:Sn) thin films are a special kind of material that exhibit electrical conductivity and at the same time, high transmittance in visible region making it suitable for Solar Cell applications. To study the effect of Sn doping on optical and electrical properties of ZnS thin films, a series of investigations were made. Thin films were deposited on glass substrate from ZnCl2, and SC (NH2)2, in ammonium solution with different low tin concentration, utilizing a chemical bath deposition technique. Optical and electrical characterization of the films with different amounts of dopant was carried out using UV-VIS-NIR spectrophotometer Solid State 3700 DUV and Four Point-Probes. All films demonstrated transmittance above 70% for wavelength above 540nm. On average reflectance was below 25% for the same wavelength. Electrical resistivity increased with increase in Sn ion concentration and it ranged between, 9.62 × 101 Ω-cm to 1.19 × 102 Ω-cm. A comparison of the obtained results revealed that low concentration of Sn doping improves transmittance of ZnS films and makes them suitable for application as window layer of ZnS:Sn/CuO solar cell.
Keywords:
Chemical Bath Deposition, Transmittance, Reflectance, Sn doped ZnS, Electrical Properties
Cite this paper: K. S. Wanjala, W. K. Njoroge, J. M. Ngaruiya, Optical and Electrical Characterization of ZnS:Sn Thin Films for Solar Cell Application, International Journal of Energy Engineering, Vol. 6 No. 1, 2016, pp. 1-7. doi: 10.5923/j.ijee.20160601.01.
1. Introduction
Zinc sulphide (ZnS) is an important groups II-VI semiconductor material with a wide direct band gap of 3.9eV bulk [1]. It is typically encountered in the more stable cubic form, known also as zinc blende or sphalerite. The hexagonal form is also known both as a synthetic material and as the mineral wurtzite. The cubic form has a band gap of 3.54eV at 300 K whereas the hexagonal form has a band gap of 3.91eV. ZnS has a melting point (phase transition) of 1020°C. The cubic form is stable at room temperature while less dense hexagonal form (wurtzite) is stable above 1020°C atmospheric pressure [2]. ZnS thin films have been found valuable in various devices. Applications of ZnS thin films which cover a wide area of interest including antireflection coating for the solar cell [3]. It forms environmental friendly buffer layer as compared to CdS layer in CIS based thin film solar cell [4]. ZnS forms wide band gap films for electroluminescent and optoelectronic devices [5]. It is also used in Photosynthetic coating [6], Blue light emitting laser diodes and acting as α- particle detector and Optoelectronic material [7]. ZnS is a well-known phosphor material with various luminescence properties like photoluminescence (PL) and electroluminescence (EL). It has been suitably used as a window layer in heterojunction photovoltaic solar cells because it decreases window absorption loses and improves the short circuit current of the cell [8]. As the applications of ZnS thin films increase, study on this material also becomes popular among researchers because of their merits such as being light and their easiness in fabrication and the possibility of forming thin film circuits. Techniques more frequently used in the preparation of ZnS films include Spray Pyrolysis [9], electro deposition [10] and chemical bath deposition technique [7], among others. Recently more attention has been bestowed on the development of cost effective thin films deposited techniques, especially in the field of photovoltaic technology, for preparation of quality alternative window layers for devices over large areas in order to economize the technology. In view of this, a simple chemical bath deposition (CBD) technique is hereby investigated for the preparation of ZnS film. Low cost and simple apparatus were used to perform the deposition. This paper presents a study of the optical and electrical properties of the resulting ZnS thin films doped with tin.
2. Experimental Procedure
The CBD of tin doped ZnS thin films was prepared from tin and zinc salts (SnCl2 and ZnC12) and an organic sulphur containing reducing agent (thiourea). The solutions of zinc chloride, thiourea and ammonia solution [NH4OH] were prepared from accurately weighed solids. ZnS:Sn thin films were obtained by mixing zinc chloride (ZnCl2) and ammonia (NH3) into a beaker. First ZnCl2 was introduced into the beaker followed by NH3. The mixture was immersed into a heated water bath. Thiourea (SC (NH2)2) was then added into the mixed solution under stirring condition. Thereafter tin chloride solution, used as Sn source for doping of ZnS Was added. NH4OH was added drop-wise using a burette to maintain alkaline solution at a pH of 9. When the deposition temperature reached 80±1°C, pre-cleaned glass slides were introduced vertically inside the substrate holder, dipped into the solution and left for deposition for 30 minutes. After completion of film deposition, the samples were removed from the beaker and allowed to cool. The deposited films were then rinsed with de-ionized water to remove soluble impurities and dried. The film samples were prepared at different tin chloride concentration ratios by volume. The deposition parameters are summarized in table 1.Table 1. Ions concentration ratios by volume in the samples 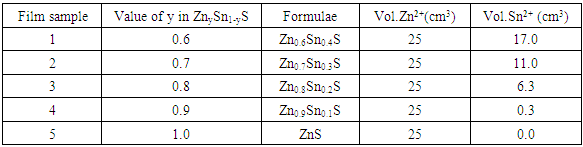 |
| |
|
3. Results and Discussions
3.1. Optical Characterization of ZnS:Sn Thin Films
Optical effects deal with transitions between bands and or energy levels inside the band gap. Thus optical measurements are of importance to analyze semiconductors. Figure 1 shows spectra transmittance of transmittance of ZnySn1-yS thin films.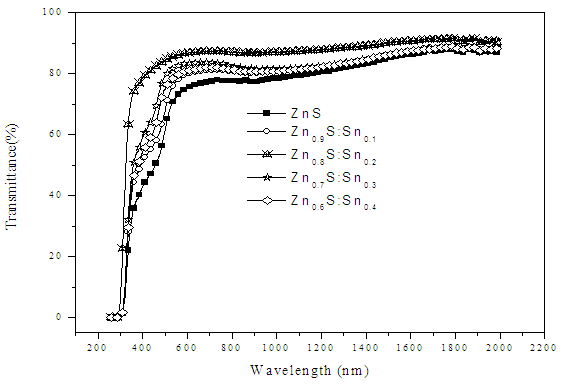 | Figure 1. A graph of transmittance (%) against wavelength (nm) |
It is observed that tin doped ZnS thin films had a higher transmittance than undoped ZnS. All films demonstrated transmittance above 70% for wavelength above 540nm. Below 400 nm there was a sharp fall in the percentage transmittance of the films, an indication of a strong increase in absorption, [11]. This is attributed to rapid change in the optical absorption coefficient, and is an indication that some states have been created in the region between the conduction and valence band. This can also be attributed to the increase in fundamental absorption as photon striking increases with increase in carrier concentration [12]. The band gap values increased with increase in doping concentration which could be attributed to the Burstein-Moss shift [11]. This is due to increase in n-type carrier concentration as the absorption edge shifts to higher energy levels. The optimized transmittance was obtained from figure 2 below.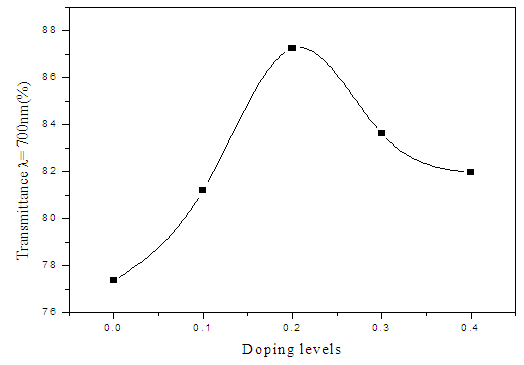 | Figure 2. Optimized transmittance against doping levels of Sn |
Figure 2 shows that optimized transmittance with Sn doping is obtained at 0.2 tin concentrations. The optimized transmittance at λ=700nm was found to be 87.3%. This transmittance value of tin doped zinc sulphide is good for the window material to be used in solar cells [13]. This value of high transmittance is comparable with the values for doped ZnS thin films deposited by authors [14]. Figure 3 illustrates the experimental and simulated data for ZnS0.8:Sn0.2 which fitted well hence producing reliable optical constants. The optical properties were then extracted from SCOUT software simulation.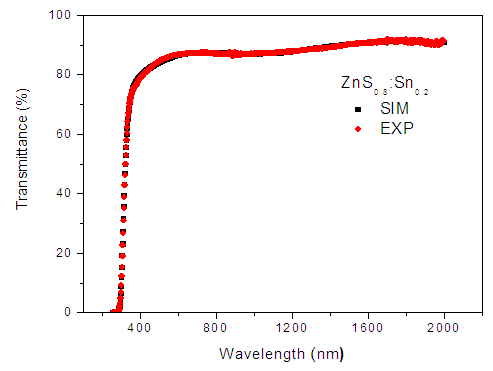 | Figure 3. Transmission simulated curves for Zn0.8S:Sn0.2 thin films |
Figure 4 shows the optical reflectance spectra for Sn doped ZnS thin films. It is observed that average reflectance was below 25% for all films. ZnS thin films had the greatest reflectance of about 25% than all ZnySn1-yS films. Results from reflectance spectra show that the presence of Sn in ZnS thin films reduces reflectance of ZnS thin films in the visible and infrared range. This reduced reflectance characteristics is so imperative since it being the window layer part of the solar cell, reflectivity is supposed to be as low as possible.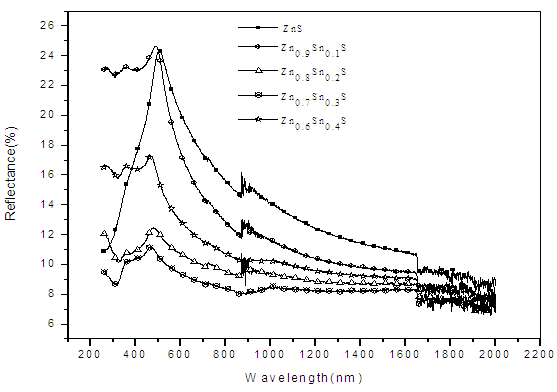 | Figure 4. A graph of Reflectance (%) against wavelength (nm) |
From the reflectance spectra, discontinuities seen at wavelength above 1600nm are as a result of source turnover from a tungsten lamp to the deuterium one so as to enable measurements close to the UV range. From the optimized data (figure 5), Zn0.7Sn0.3S had the least reflectance of 8.6% at a wavelength 700nm.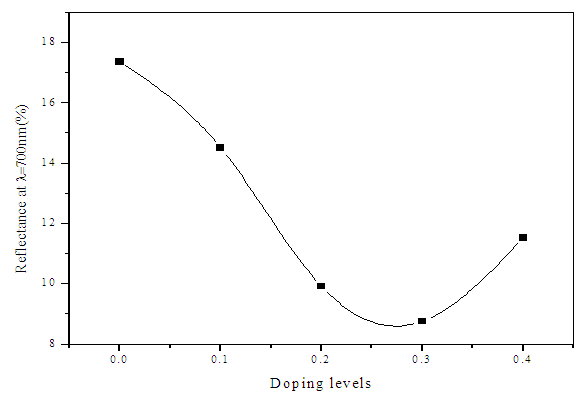 | Figure 5. Optimized Reflectance with doping levels of Sn |
The absorbance values for all the films were calculated from transmittance and reflectance data using the expression: 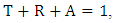 | (1) |
So that A = 1 – [T + R]From figure 6, ZnySn1-yS films have good absorption at short wavelength region, the absorption decreased with increasing wavelength of solar radiation.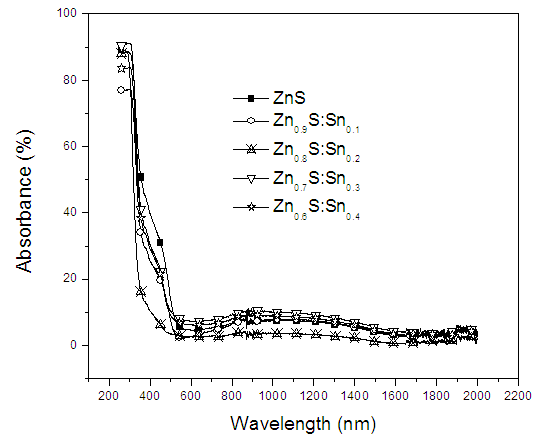 | Figure 6. A graph of absorbance (%) against wavelength (nm) |
From figure 7, there was a decrease in absorbance up to a doping level 0.2 of tin at λ=700nm, then a slight increase in absorption. The increase in absorption occurs when the photon energy is equal to the value of the energy gap when electronic transfers between the valence band and conduction band begin. Thin films of Zn0.8Sn0.2S had the lowest of absorbance of 2.8% at wavelength 700nm.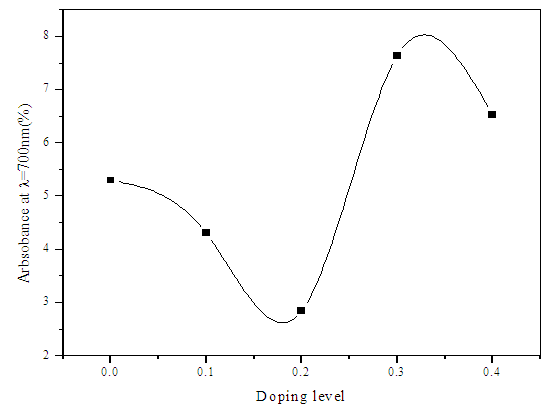 | Figure 7. Absorbance with doping levels of Sn at λ= 700nm |
Band gaps were obtained by plotting (hv) against (αhv)2 and extrapolating the linear part. By extrapolating the linear portions of the plots of (αhv)2 against hv to where (αhv)2 = 0, the value where the extrapolated line cuts energy (hv) axis is the band gap. Figure 8 shows how band gaps of doped and undoped ZnS films were obtained.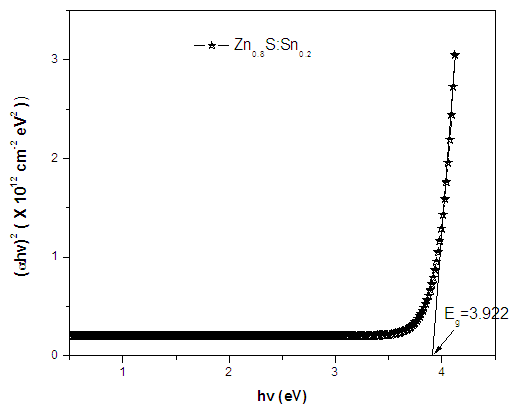 | Figure 8. Graphical determination of band gap of Zn0.8Sn0.2S thin film |
Figure 9 illustrates a graph of how the band gaps varied with concentration of tin in the bath.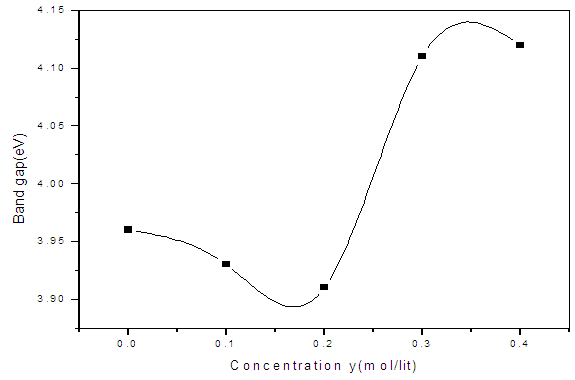 | Figure 9. The plot of band gap energy (eV) of ZnS:Sn thin films versus doping concentrations y |
From figure 9, energy band gaps of ZnS:Sn films decreased with increase of doping concentration of tin to the minimum band gap of 3.92 eV for Zn0.8S:Sn0.2 thin film. Decrease in optical band gap energy can be attributed to creation of new donor levels in the forbidden zone; and a shift in the Fermi level causing the band structure of the films. Further increase of doping concentration of tin resulted to increase of band gap with highest one at 4.12 eV for Zn0.6S:Sn0.4 film. This is attributed to absorption edge being pushed to higher energies as a result of all states close to the conduction band being populated with excited electrons from the valence band. As doping concentration was increased, more and more donor states were produced which pushed Fermi level higher in energy. Values of the band gaps were in the range of 3.91 to 4.12eV as seen from figure 9. These Band gap values are in agreement with [15], obtained in determination of optical properties of ZnS thin films which were in the range 3.64 - 4.00eV.The values of refractive indices of ZnS:Sn deduced from scout fitting of reflectance data shows that refractive index decreases with increasing wavelength of incident photon as depicted in spectral plots in figure 10. This is because at higher wavelengths, photon energy is less than band gap hence is transmitted. Zn0.8S:Sn0.2 films have higher refractive indices within the visible range of the spectrum which is less than 1.5 at λ = 400nm. This has an implication that reflectance decreases with increase in refractive index [16]. It was also observed that tin concentration in the bath increased the refractive index. This is a good observation for window layers that are required to have high transmittance properties.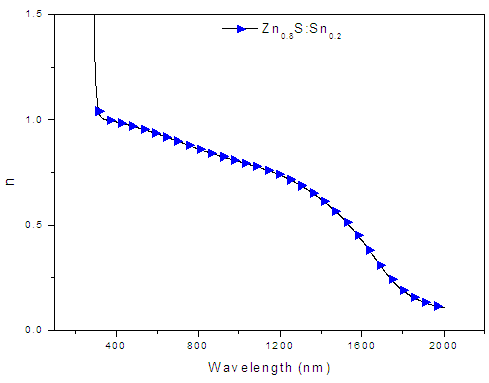 | Figure 10. A graph of refractive index (n) against wavelength (nm) for Zn0.8Sn0.2S films |
3.2. Electrical Properties of ZnS:Sn Thin Films
The sheet resistivity of ZnS:Sn films was measured by using the four point probe. From figure 11 it is observed that resistivity increased with increase in Sn ion concentration and it ranged between, 9.62 × 101 Ω-cm to 1.19 × 102 Ω-cm.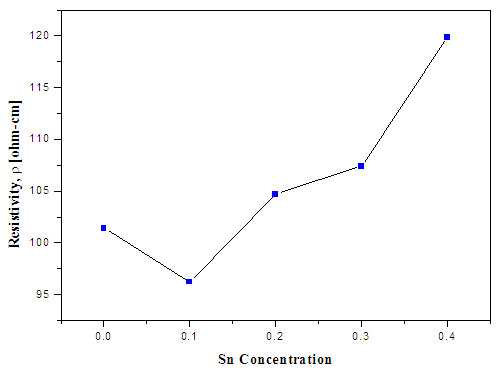 | Figure 11. Sheet resistivity against Sn concentration in ZnS:Sn films |
The low sheet resistance obtained at 0.1 is due to the Sn substitution at Zn in the grain boundaries leaving additional electrons acting as donors. At higher doping concentration, segregation of Sn takes place resulting in the increase of resistance [17]. Though the film resistivity increases it can be used for photovoltaic applications with adjustments on resistivity either by further doping with dopants that reduce resistivity or introducing low resistivity grids. Similar sheet resistivity measurements for ZnS were obtained using Van der Pauw technique in the order of 101 Ω-cm to 102 Ω-cm and electrical conductivity of 10-2 to 10-3 [Ω-cm]-1.
4. Conclusions
ZnS:Sn films were successfully deposited on glass substrate by chemical bath deposition technique at 80°C. Electrical and optical properties of the deposited thin films have been found to be significantly dependent on Sn concentration during deposition. From optimized data, Zn0.8S:Sn0.2 films had high transmittance of 87% at λ=700nm and corresponding reflectance of 10%. The band gap obtained ranged from 3.91eV to 4.12 eV with Zn0.8S:Sn0.2 films having band gap energy of 3.92eV. Refractive index and dielectric constants decreased with increase in Sn concentration indicating an increase in transparency. Their resistivity increased with increase in zinc concentrations and it ranged from 1.01 × 102 Ω-cm to 1.20 × 102Ω-cm. This was attributed to the introduction of tin into the molecular interspacing in ZnS crystals. Pure ZnS films had a resistivity of 1.01 × 102 Ω-cm. We recommend some work to be done on effect of Sn on structural properties of Zn0.8S:Sn0.2 films.
ACKNOWLEDGEMENTS
The authors are grateful to the assistance offered by the Physics Department of Kenyatta University where this study was carried out. They also acknowledge the University of Nairobi department of Physics [Chiromo Campus] for kindly providing the UV-VIS-NIR spectrophotometer 3700 for the spectral analysis of the deposited thin films.
References
[1] | Bhaskarjyoti Bodo, Divya Prakash, and Kalita, P. K. (2012). Synthesis and Characterization of ZnS:Mn Nanopartilces. International Journal of Applied Physics and Mathematics 2:3. |
[2] | Xie Hai-Qing, Chen Yuan, Huang Wei-Qing, Huang Gui-Fang, Peng Ping, Peng Li, Wang Tai-Hong, Zeng Yun “Optical Characteristics of La-Doped ZnS Thin Films Prepared by Chemical Bath Deposition.” China Physics Letter. Vol. 28, No. 2 (2011) 027806. |
[3] | M. S. Shinde, P. B. Ahirrao, R. S. Patil1, “Structural, Optical and Electrical Properties of Nanocrystalline ZnS thin films Deposited by Novel Chemical Route” Archives of Applied Science Research, 2011, 3 (2):311-317. |
[4] | Nasr B T, N. Kamoun, M. Kanzari, R. Bennaceur (2006). Effect of pH on the properties of ZnS thin films grown by chemical bath deposition. Thin Solid Films, 500: 4 – 8. |
[5] | Rahdar, A., Arbabi, V., and Ghanbari, H., (2012). Study of Electr-Optical properties of ZnS nanoparticles prepared by Colloidal Particles method. World Academy of Science, Engineering and Technology 61:657. |
[6] | Ali A. Yousif, Aseel A. Jasib, Int. J. Innovative Sci., Engin. Technol. 2 No 3, 886 (2015). |
[7] | G. Nabiyouni, R. Sahraei, M. Toghiany1, M. H. Majles Ara and K. Hedayati. Preparation and characterization of nanostructured ZnS thin films grown on glass and n-type si substrates using a new chemical bath deposition technique: Rev.Adv.Mater.Sci. 27(2011) 52-57. |
[8] | Tamargo, M.C. (2002). II-VI Semiconductor materials and their applications. Taylor & Francis, 96-97. |
[9] | A. Djelloul, M. Adnane1, Y. Larbah, T. Sahraoui1, C. Zegadi1, A. Maha, B. Rahal: Properties Study of ZnS Thin Films Prepared by Spray Pyrolysis Method, Journal of Nano- and Electronic physics Vol. 7 No 4, 04045 (2015). |
[10] | J. S. Patil, S.S Dhasade, J.V Thombare, V. J. Fulari (2015) Structural, Morphological and Optical Studies of ZnS Thin Films Produced by Electrodeposition Method. ISSN-Science- 0250-5347, Volume No. 41 (2), 2014-2015. |
[11] | Kim, H., R. Auyeung, C. Y., and Pique, A., (2009). Thin Solid Films, 516: 5052. |
[12] | Kumar, T. and Sankaranarayanan, S. (2009). Growth and characterisation of CdZnS thin films by short duration microwave assisted chemical bath deposition technique. Chalcogenide Letters, 6: 555- 562. |
[13] | Nadeem M. Y., and Waqas Ahmed. Optical Properties of ZnS Thin Films (2000). Turkish Journal of Physics 24:651-659. |
[14] | Anthoy Owusu (2012), Investigating the optical properties of ZnS thin films deposited from Chemical Acids Baths. Thesis 2012. |
[15] | Shelake. V, M.P Bhole, D.S Patil. (2008). Aluminium doped Zinc oxide films as a transparent conducting electrode for organic light emitting deveces. Optoelectronics and advanced materials-Rapid Communications.2:353-355. |
[16] | Murali, K. R., and Kumaresan, S., (2009). “Characteristics of brush plated ZnS films.” Chalcogenide Letters, 6:17 – 22. |
[17] | Shinde, M. S., Ahirrao, P. B., and Patil, R. S. (2011). Structural, Optical and Electrical Properties of Nanocrystalline ZnS thin films Deposited by Novel Chemical Route. Archives of Applied Science Research, 3(2): 311-317. |