Duleeka Gunarathne1, Sumudu Sajeewareka Jatunarachchi2, Nihal Srikantha Senanayake2, Bo Wei3
1National Engineering Research & Development Centre of Sri Lanka, Industrial Estate, Ekala, Ja-ela, Sri Lanka
2Department of Mechanical Engineering, The Open University of Sri Lanka, Nawala, Nugegoda, Sri Lanka
3Department of Energy Technology, Royal Institute of Technology, Stockholm, Sweden
Correspondence to: Nihal Srikantha Senanayake, Department of Mechanical Engineering, The Open University of Sri Lanka, Nawala, Nugegoda, Sri Lanka.
Email: |  |
Copyright © 2012 Scientific & Academic Publishing. All Rights Reserved.
Abstract
Biomass gasification to produce combustible gas is one of the promising sustainable energy options available for many countries. At present, a few small scale community based power generation systems using biomass gasifiers are in operation in Sri Lanka. However, due to insufficient knowledge on the relationships between important parameters, these systems are not operating properly in full capacity. This stands as an obstacle to further expand the use of gasifier technology. Hence, in order to improve the knowledge of the gasification and to simplify the process, researches are being carried out to determine the relationships between different parameters and to optimize the power generation. The objective of this study was to identify most influential parameters related to fuel wood gasification using a down draft gasifier to improve the gasification processes. A downdraft gasifier of 10kW electrical capacity was used to study the effect of equivalence ratio (ER) on the specific gas production, the calorific value of gas produced and the efficiency using three throat diameters (125mm, 150mm and 175mm). Trials were carried out for each throat diameter by varying the supply air flow to change the ER, and the gas samples were tested for its composition under steady state running condition. Using mass balances for each chemical element(C and N), the conversion efficiency, heating value and the specific gas production were determined. The results showed that for all throat diameters the calorific value of gas reduced with the increase of ER giving highest calorific values at an ER value around 0.36. The highest specific gas production was obtained at 0.425ER and throat diameter 175mm. The specific gas production increased up to a certain limit with ER under all throat diameters. The conversion efficiency reduced with ER in a similar pattern for all three throat diameters. However, the gas composition did not show appreciable change with the change of throat diameter.
Keywords:
Renewable Energy, Gasifier, Throat Diameter, Equivalence Ratio
Cite this paper: Duleeka Gunarathne, Sumudu Sajeewareka Jatunarachchi, Nihal Srikantha Senanayake, Bo Wei, The Effect of Throat Diameter on the Performance a Downdraft Biomass Gasifier, International Journal of Energy Engineering, Vol. 3 No. 3, 2013, pp. 171-175. doi: 10.5923/j.ijee.20130303.07.
1. Introduction
The current status of the world’s energy consumption and energy mix, the continuous growth of the world population from 6.8 to about 9 billion by 2050, and today’s still growing global energy demand will inevitably lead to a conflict between a happy planet (stable ecosystems, clean environment) and a happy world population. Thus, the question arises how the world’s unceasing demand for energy can be reconciled with the absolute necessity to preserve the integrity of the biosphere[1]. Evidence suggests that conventional oil production has a limited capacity to meet growing demand, and most additional demand will have to be met by unconventional sources. Since the globe is turning towards the sustainable development, renewable energy technologies are getting more attention of all the people these days. Depletion of fossil fuels and increasing the climate change has resulted in this dramatic change[2].Sri Lanka heavily depends on the imported fossil fuel for industrial use and electricity power generation. Even though the country has several hydropower plants, fossil fuel has to be used to supplement the electrical power demand, especially under dry weather conditions. The electricity generation in the country was 10,714GWh in 2010 that was an increase of 8.4 per cent compared to the previous year due to the growth in economic activities. The share of hydro power in total power generation was only 52.6% per cent which was 39.3% in the previous year reflecting the changes in rainfall in catchment areas[3]. Further, in the areas of low population densities and under difficult -to -reach geographical conditions, it is not economically feasible to extend the national electricity grid for entire population in Sri Lanka. Therefore, the power requirements of this un-served remote people have to be met by means of off-grid technologies, which also contribute to preserve the environment. Basically there are four options available for off-grid power generation in Sri Lanka, namely hydro power, solar power, wind power and dendro (biomass) power. The large capacity hydro power generation has reached the full potential and also it does not provide a solution to power demand of isolated and difficult-to-reach areas. Micro hydro schemes are the least cost technology but that is limited to hilly and wet areas due to geographical and climate conditions required. Solar power systems are limited due to high cost involved. Wind energy systems are less feasible due to unavailability of required wind speed throughout the year. Therefore, most suitable option for Sri Lankan dry zones with bare lands for plantation of firewood and who cannot afford too much money for solar panels is dendro power schemes. Biomass gasification is a promising renewable energy technology for supplying thermal energy and generating electric power. It is vital to use biomass for stand-alone power generation in remote areas where the national grid is not available. Unlike thermal applications, power generation demands low tar producer gas which in turn prefer down-draft gasifier, as down-draft gasifier generally produce low particulate and low-tar gas. Biomass gasification is a thermo-chemical process that uses partial oxidation to convert organically derived carbonaceous feedstock into a gaseous product known as synthesis (producer) gas. This gas mainly consists of hydrogen and carbon monoxide, with lesser amount of carbon dioxide, water, methane, higher hydrocarbons, and nitrogen. The reactions occurring in the process are given as follows[4].
All the reactions are in equilibrium, hence the products of gasification process consists of a mixture of carbon monoxide, carbon dioxide, methane, hydrogen and water vapour. An oxidation zone is formed at the level where air is introduced. Oxidation reactions are highly exothermic and result in a temperature rise up to 1200 - 1500°C.The performance of biomass gasifiers is determined in terms of the cold gas and the mass conversion efficiencies, the flow rate of the producer gas and the calorific value[5]. Studies have been reported on how the performance of gasifier varies with equivalence ratio[5, 6]. In these studies they have studied the change of gas calorific value with equivalence ratio. The calorific value was found to be increasing with the equivalence ratio, but tends to reduce after a certain critical value. On other hand, gas production rate per unit weight of biomass was found to be a maximum close to the equivalence ratio value that gave maximum calorific value.The throat diameter also has an effect on the conversion efficiency of the gasifier and it has been reported that smaller throat diameters gives that higher conversion efficiency. The efficiency also has been found to increase with the distance from the top reduction zone to the throat location[7].According to the studies by Sharma in 2011 any factor results in higher reaction temperatures due to the gasification reactions (increase of air/fuel ratio) or operating conditions (increase of gas flow rate) gives better gasifier performance[8].The present study was conducted to investigate the effect of throat diameter on the performance of a down draft gasifier in terms of cold gas efficiency, the producer gas calorific value and the specific gas production.
2. Method
A down draft gasifier of 10 kW electrical capacity was used in the study. Experimental trials were carried out using three sizes of throat diameters. The throat diameters used were 125mm, 150mm and 175mm. For each size of throat, 6 experimental trails were carried out with different settings of air supply in order to vary the equivalence ratio. The equivalence ratio is the ratio of operating air-fuel ratio (A/F)op to the Stoichiometric air – fuel ratio (A/F)st. Wood sticks made out rubber trees cut to size approximately 25mm x 25mm diameter were used as the fuel. 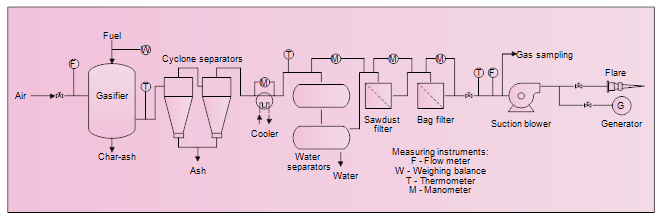 | Figure 1. Gasifier system indicating measuring points |
After about half an hour in stabilized condition of the gasification, three samples of producer gas were collected and the compositions were analyzed. Pressure readings were taken at gas cooler, water separator, sawdust filter and bag filter with manometers installed at these locations as shown in Figure 1.
3. Theoretical Considerations
Lower Heating Value of Fuel WoodBomb calorimeter measures the Higher Heating Value (HHV). The LHV is computed using the following equation. | (1) |
Fm = Weight fraction of moisture = 0.2226[9]hw = Heat of vaporization of water = 2.283MJ/kgGas to Fuel Ratio (G/F)In order to determine the Producer Gas to Fuel Ratio (G/F) carbon mass balance in the gasifier is used.Using Carbon mass balance;
Cf = Rate of C input in kg/hCg = Rate of C leaving gasifier with gas in kg/hCc-a = Rate of C leaving the gasifier with char- ash in kg/hCt = Rate of C leaving the gasifier with tar in kg/hAssuming carbon in char-ash and tar is negligible compared to carbon in the producer gas; | (2) |
Mass percentage of carbon in dry fuel wood is taken as 52.2%[10]. | (3) |
Where, F = Fuel consumption rate in kg/hFrom Equations (2) and (3); | (4) |
The mass of C in 1m3 of producer gas, Cgv is computed as follows:
Vc = Volume fraction of C containing compound
= Density of compoundwc = C weight per mole of compoundIf G is producer gas flow rate in m3/h, | (5) |
From equations (4) and (5);
 | (6) |
Air to Gas Ratio (A/G)In order to determine the Air flow to Gas flow (A/G) nitrogen balance is used.Using Nitrogen mass balance;
Nf = Rate of nitrogen input to the gasifier in kg/hNa = Rate of nitrogen input to the gasifier with air kg/hNg = Rate of nitrogen leaving the gasifier with air kg/hAssuming nitrogen in fuel is very small compared to the nitrogen in air; | (7) |
Taking mass of nitrogen in 1m3 of air as 0.75; | (8) |
Where A= Supply air flow rate (m3/h)From Equations (7) and (8); | (9) |
The mass of N in 1m3 of producer gas (Ngv) is computed as similar to that of C. Then, | (10) |
From Equations (9) and (10);
 | (11) |
Equivalence Ratio (ER)Equivalence Ratio reflects the combined effect of air flow rate and fuel flow rate. This is defined as the ratio of operating air-fuel ratio to Stoichiometric air-fuel ratio. 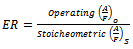 | (12) |
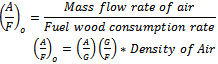 | (13) |
Stoichiometric air-fuel ratio is taken as 6.36 kg of air per kg of wood[9]Lower Heating Value of GasLower Heating value (LHV) of producer gas is determined from the chemical composition of the gas and LHV of individual components.
Gasification Efficiency or cold gas efficiency
4. Results and Analysis
The calorific value of the producer gas, the cold gas efficiency and the specific gas production computed for all three throat diameters for different equivalence ratios are graphically presented in Figures 2, 3 and 4 respectively.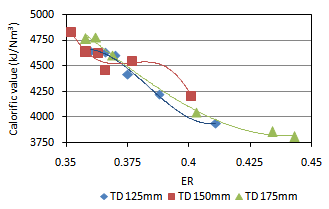 | Figure 2. Change of Calorific value with ER for different throat diameters |
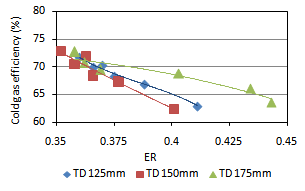 | Figure 3. Change of cold gas efficiency with ER for different throat diameters |
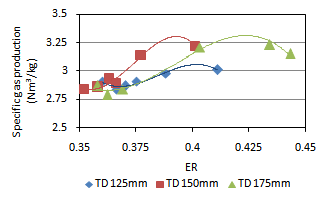 | Figure 4. Change of specific gas production with ER for different throat diameters |
5. Discussion
The calorific value of the producer gas showed decesing trend with the increase of equivalence ratio for all throat diameters used as evident from Figure 2. With equivalence ratio around 0.36 the calorific value was found to be more or less equal to 4.6MJNm-3 for all throat diametres. As far as cold gas efficiency is concerned its value for all throat diamteres was found to be converging to a fixed value a little higher than 70% at equivalnce ratio of 0.365. The results also showed that cold gas efficiency tends to decline with the incraese of equivalnce ratio. Opposeing to this decreaing trend in the gas calorific value and the cold gas efficiency, the specific gas production increased with the increase of equivalnec ratio upto a certain a certain value of equivalence ratio that varied according to the throat diameter (Figure 4). The maximum specific gas production rate of just above 3.25 Nm3/kg was seen near 0.425 equivalence ratio with the 175mm throat diameter. These results are in agreement with the studies reported by Zainal et al (2002) in which they found that calorific value and the cold gas efficieny increased with the increasae of equivalnce ratio up to a certain point[5]. However, the effect throat diameter was not addressed in the study. The linear incraesse of specific gas prodcution with equivalence ratio as reported by them was not establihed in the present study and it was found to show an slightly decresaing trend after a a cerain value of equivalnce ratio. The conclusion that cumulative conversion efficiency was incrased with the smaller throat diameters as reported in a previous study[7] was not supported in this study. This could be due to that fact that equlivalnce ratio was not taken as a variable in the their study. According the present study the efficiency was found to incrase slightly with the incrase of throat diameter, but with different equlivalence ratios.Table 1 compares higest values of calorific value, cold gas efficiency and the specific gas production at their coressponding equilvalnce ratios. Table 1. Comparison of performance at different throat diameters 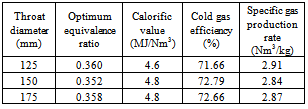 |
| |
|
Table 2. Gas composition ranges obtained with different throat diameters 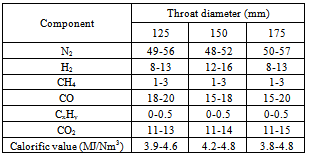 |
| |
|
Table 2 depicts the measured range of different gasses for all three throat diameters. No significant variation of composition ranges and calorific value ranges were evident for different throat diameters.
6. Conclusions
Based on the results obtained during this study, following conclusions were made.● There was a clear variation of performance of the gasifier with equivalence ratio for all three throat diameters. ● Lower equivalence ratios gave better performance in terms of calorific value and cold gas efficiency.● The spacific gas production showed incraesing trend up to a certain value of equivalence ratio and then decreased with the incrasee of equivalnce ratio.● The cold gas efficiency showed a slight increase with the increase of throat diameter, but with different equivalence ratios.● The gas compositions obtained for three different throat diameters are comparable with typical producer-gas composition and did not show appreciable change with the change of throat diameter.● For analysing the effect of fuel size, more trials should be done.
References
[1] | World Energy Outlook, International Energy Agency, 75739, Paris Cedex 15, France, 2008. |
[2] | Bergerson, J. and Keith, D. 2006, Life cycle assessment of oil sands technologies, Alberta Energy Futures Project, Institute for Sustainable Energy, Environment, and Economy (ISEE), University Calgary. |
[3] | Annual Report (2010) Central Bank of Sri Lanka. |
[4] | Franco, C., Pinto, F., Gulyurtlu, I. and Cabrita, I., 2003, The study of reactions influencing the biomass steam gasification process, Fuel, 82, 835-842. |
[5] | Zainal, Z.A., Ali Rifau, Quadir, G.A. and Seetharamu, K. N., 2002, Experimental Investigation of a Downdraft Biomass Gasifier, Biomass and Bioenergy, 23, pp 283-289. |
[6] | Pratik, N.S and Babu, B. V., 2009, Experimental studies on producer gas generation from wood waste in a downdraft biomass gasifier, Bioresource Technology, 100, 3127-3133. |
[7] | Siva Kumar, S., Pitchandi, K. and Natarajan, E., 2008, Modeling and simulation of down draft wood gasifier, Journal of Applied Sciences 8(2), pp 271-279. |
[8] | Sharma, A. K., 2011, Experimental investigations on a 20 kWe, solid biomass gasification system, Biomass & Bioenergy 35 (1), pp 421-428. |
[9] | Solar Energy Research Institute, Handbook of Biomass Downdraft Gasifier Engine Systems, Solar Technical Information Program, Colorado, 1988, ISBN: 91-7283-506-0. |
[10] | FAO Forestry paper 72, Wood gas as engine fuel, Food and Agriculture, Organization of the United Nations, 1986, ISBN: 92-5-102436-7. |