Charles U. Ndujiuba 1, Adetokunbo O. Oloyede 2
1Electrical & Information Engineering, Covenant University, Ota, Nigeria
2Computer Engineering Department, College of Technology, Yaba Lagos, Nigeria
Correspondence to: Charles U. Ndujiuba , Electrical & Information Engineering, Covenant University, Ota, Nigeria.
Email: |  |
Copyright © 2015 Scientific & Academic Publishing. All Rights Reserved.
This work is licensed under the Creative Commons Attribution International License (CC BY).
http://creativecommons.org/licenses/by/4.0/

Abstract
This work analyses the performance of different feeding techniques for rectangular microstrip patch antennas used in wireless communications applications, such as in Wimax and LTE technologies. Three types of feeding arrangements are discussed here; Microstrip Line feed, Coaxial probe feed, and Aperture-coupled feed techniques. The performance of microstrip patch antenna system depends on the characteristics of the antenna element and the substrate as well as the feed configuration employed. Here the principal characteristics of interest are the antenna input impedance, mutual coupling, bandwidth, radiation pattern and return loss. In this paper, we analyze these characteristics for each feed technique, and compare them with those of the other techniques. This enables the system designer to make well informed judgement on the best feeding arrangement for his application. MATLAB has been used for the simulations and evaluations of the various performance metrics.
Keywords:
Return Loss, LTE systems, Antenna array, Mutual coupling, Input impedance, Bandwidth, Radiation pattern, MIMO
Cite this paper: Charles U. Ndujiuba , Adetokunbo O. Oloyede , Selecting Best Feeding Technique of a Rectangular Patch Antenna for an Application, International Journal of Electromagnetics and Applications, Vol. 5 No. 3, 2015, pp. 99-107. doi: 10.5923/j.ijea.20150503.01.
1. Introduction
The Microstrip patch antennas are well known for their performance and their robust design, fabrication and their extent of usage. The usage of the Microstrip antennas is spreading widely in all the fields and areas and now they are booming in the commercial aspects due to their low cost of the substrate material and the fabrication. It is also expected that due to the increasing usage of the patch antennas in the wide range this could take over the usage of the conventional antennas for the maximum applications [4]. Microstrip patch antenna has several applications, some of which are discussed below:
1.1. Mobile and Satellite Communication Application
Mobile communication requires small, low-cost, low profile antennas. Microstrip patch antenna meets all requirements and various types of microstrip antennas have been designed for use in mobile communication systems. In case of satellite communication circularly polarized radiation patterns are required and this can be realized using either square or circular patch with one or two feed points.
1.2. Global Positioning System Applications
Nowadays microstrip patch antennas with substrate having high permittivity materials are used for global positioning system. These antennas are circularly polarized, very compact and quite expensive due to its positioning. It is expected that millions of GPS receivers will be used by the general population for land vehicles, aircraft and maritime vessels to find their position accurately.
1.3. Radio Frequency Identification (RFID)
RFID uses in different areas like mobile communication, logistics, manufacturing, transportation and health care [2]. RFID system generally uses frequencies between 30 Hz and 5.8 GHz depending on its applications. Basically RFID system is a tag or transponder and a transceiver or reader.
1.4. Worldwide Interoperability for Microwave Access (WiMax)
The IEEE 802.16 standard is known as WiMax. It can reach up to 30 mile radius theoretically and data rate 70 Mbps. Microstrip patch antenna generates three resonant modes at 2.7, 3.3 and 5.3 GHz and can, therefore, be used in WiMax compliant communication equipment.
1.5. Radar Application
Radar can be used for detecting moving targets such as people and vehicles. It demands a low profile, light weight antenna subsystem, the microstrip antennas are an ideal choice. The fabrication technology based on photolithography enables the bulk production of microstrip antenna with repeatable performance at a lower cost in a lesser time frame as compared to the conventional antennas.
1.6. Rectenna Application
Rectenna is a rectifying antenna, a special type of antenna that is used to directly convert microwave energy into DC power. Rectenna is a combination of four subsystems i.e. Antenna, ore rectification filter, rectifier, post rectification filter. In rectenna application, it is necessary to design antennas with very high directive characteristics to meet the demands of long-distance links. Since the aim is to use the rectenna to transfer DC power through wireless links for a long distance, this can only be accomplished by increasing the electrical size of the antenna [15].
1.7. Telemedicine Application
In telemedicine application antenna is operating at 2.45 GHz. Wearable microstrip antenna is suitable for Wireless Body Area Network (WBAN). The proposed antenna achieved a higher gain and front to back ratio compared to the other antennas, in addition to the semi directional radiation pattern which is preferred over the omni-directional pattern to overcome unnecessary radiation to the user's body and satisfies the requirement for on-body and off-body applications. A antenna having gain of 6.7 dB and a F/B ratio of 11.7 dB and resonates at 2.45GHz is suitable for telemedicine applications.
1.8. Medicinal Applications of Patch
It is found that in the treatment of malignant tumours the microwave energy is said to be the most effective way of inducing hyperthermia. The design of the particular radiator which is to be used for this purpose should possess light weight, easy in handling and to be rugged. Only the patch radiator fulfils these requirements. The initial designs for the Microstrip radiator for inducing hyperthermia was based on the printed dipoles and annular rings which were designed on S-band. And later on the design was based on the circular microstrip disk at L-band. There is a simple operation that goes on with the instrument; two coupled Microstrip lines are separated with a flexible separation which is used to measure the temperature inside the human body. The most preferred antennas on any mobile unit for a Multiple-Input-Multiple-Output (MIMO) system are microstrip or patch antennas, due to their low cost and ease of fabrication. These benefits justify our interest in this subject.The main drawback of these antennas is low bandwidth and there are various techniques proposed for improving the bandwidth.The bandwidth of the microstrip patch antenna can be improved by increasing the thickness of substrate or by decreasing its electric permittivity value.In addition to compatibility with integrated circuit technology, microstrip antenna systems offer other benefits such as thin profile, light weight, low cost and conformability to a shaped surface. Its main disadvantage is inherent narrow bandwidth arising from the fact that the region under the patch is basically a resonant cavity with a high quality factor.Feed structures for microstrip antennas take various forms. The main ones are the coaxial probe, the microstripline, and the aperture coupling methods.The choice of the feed arrangement may depend on the application of the antenna system. For example, at millimeter wave frequencies the use of the aperture coupling obviates problems of large probe self-reactances associated with probe feeds. The connector effects, at the junction of the probe and the antenna element, give rise to fundamental limits to antenna performance due to radiation from the discontinuity at the junction.In the microstrip line feed technique, a conducting strip is connected directly to the edge of the Microstrip patch. The conducting strip is smaller in width as compared to the patch. This kind of feed arrangement has the advantage that the feed can be etched on the same substrate to provide a planar structure. However, an increase in the thickness of thedielectic substrate will increase surface waves and spurious feed radiation, which hampers the bandwidth of the antenna. This feed radiation also leads to undesired cross polarized radiation. This method is advantageous due to its simple planar structure.The coaxial feed or probe feed is a very common technique used for feeding Microstrip patch antennas. The inner conductor of the coaxial connector extends through the dielectric and is soldered to the radiating patch, while the outer conductor is connected to the ground plane. The main advantage of this type of feeding scheme is that the feed can be placed at any desired location inside the patch in order to match with its input impedance.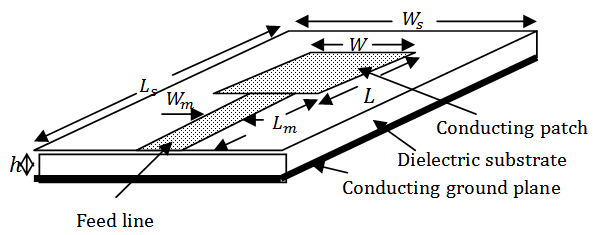 | Figure 1. Microstrip line feed techniqu |
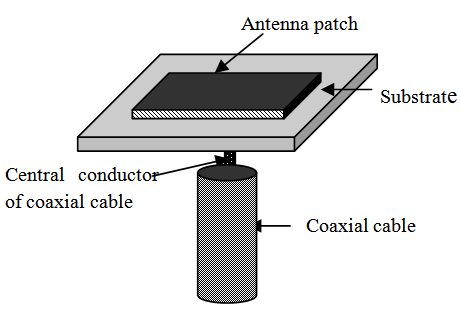 | Figure 2. Coaxial probe feed technique |
However, its major drawback is that it provides narrow bandwidth of 2-5% and is difficult to model since a hole has to be drilled in the substrate and the connector protrudes outside the ground plane, thus not making it completely planar for thick substrates. Also, for thicker substrates the increased probe length makes the input impedence more inductive leading to matching problems. The microstrip line feed and the coaxial feed suffer from numerous disadvantages. The basic geometry of a single aperture-coupled microstrip patch antenna is shown in Figure 3 [1, 2]. It consists of two substrates bonded together and separated by a ground plane between them. On the top substrate is printed the radiating patch (antenna) while a microstrip feedline is printed on the bottom substrate, which is electromagnetically coupled to the patch by means of a small resonant aperture in the ground plane.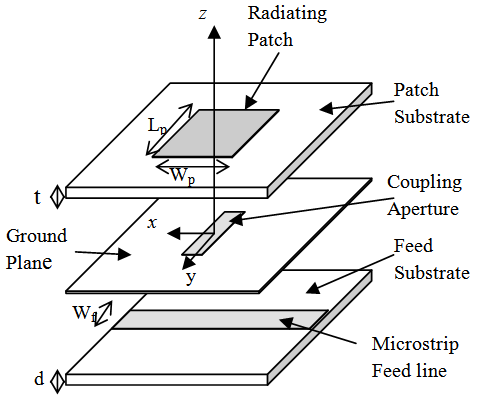 | Figure 3. Aperture-coupled feed technique |
Several advantages are obtained by the use of such two-sided configuration. These include isolation of the feed network from the radiating aperture, which eliminates the spurious feed network radiation that can degrade polarization and sidelobe levels. Also, the two-sided configuration provides two distinct microstrip line media so that the antenna substrate can be chosen to optimize the performance of the radiating patches (e.g. low permittivity to improve radiation and increase bandwidth), and the feed substrate can be chosen independently to optimize feed performance.
2. Analysis of the Performance Parameters
In general, there are two lines of approach to deduce the radiation fields. One is to find the current distributions along the antenna structure and then obtain the radiation fields from these current sources. The other is to find the fields at the exit region. These fields act as equivalent sources, from which the radiation fields are obtained. Under these two approaches a number of methods of analysis are in use. They can be broadly classified under two categories: i. Simplified Method● Transmission-line model● The Cavity model● Method of segmentationii. Exact or Rigorous Method● The Integral Equation methodIn this work the Transmission-Line Model is employed in order to have a fast and efficient procedure for computing the parameters of the radiating patch.
2.1. Transmission-Line Analysis of Patch Antennas
In this model, a rectangular microstrip antenna patch, of figure 3, is viewed as a resonant section of a microstrip transmission line, since it has a physical structure derived from microstrip transmission. The transmission line model does not include surface waves. Therefore, the application is limited to antenna configurations where the thickness and the substrate permittivity are sufficiently small to avoid considerable excitation of those surface waves. But in practice, this is not a severe limitation.The patch is characterised by the resonant length L (resonant for the fundamental mode), the width W, and conductivity σp, while the substrate is electrically characterised by a relative permittivity εr, and a loss tangent δs. Also for the purpose of the analysis the dielectric substrate is supposed to have infinite dimensions in the plane of the patch, but in practice, it has a length Ls, and a width Ws, and a thickness h.The main step in the modelling of the microstrip antenna by the transmission-line equivalent is the representation of the open-ended terminations by a parallel admittance Ys. These open-ends do not perform as perfect open circuits (Figure 4), because the field lines do not stop abruptly at the end of the conductor. This extension of stray fields beyond the ends of the strip can be interpreted as an electrical lengthening ΔL of the line which implies an amount of stored energy.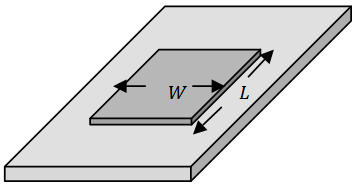 | Figure 4. Rectangular microstrip patch |
In the fundamental mode, only the contribution from the two open ends is important. The sources of radiation can be limited to two narrow zones along the two open ends of the patch.The field in these two narrow zones can be thought of as the field of two rectangular slots in an infinite, perfectly conducting plane.For the fundamental mode of the microstrip antenna the tangential field in these two slots can be considered to be uniformly distributed. The calculation can then be decomposed into:a) Calculation of the radiated field which permits the determination of Gs,b) Calculation of the input admittance, hence the resonant frequency.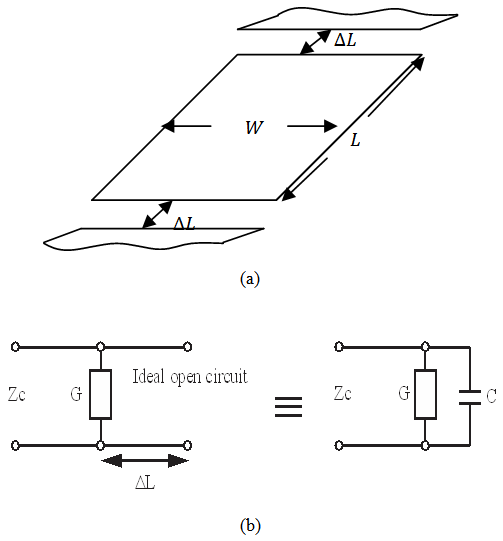 | Figure 5. Schematic representation of open-ends of patch |
2.2. Calculation of the Radiated Field
The tangential electrical field in the slot apertures can be written as: | (1) |
As a result of the presence of image on the slot we find that | (2) |
The uniform distribution of the tangential electric field,
on the slots permits us to calculate the far-field,
from each of the slots. | (3) |
where w = length of the sloth = width of the slot | (4) |
and
N’ describes the surface of the slot with 
with the magnetic current source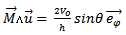 | (5) |
we obtain
with 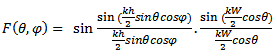 | (6) |


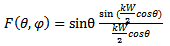 | (7) |
The total field radiated by two slots is obtained by:(a) Redefining the origin at the center of the antenna,(b) Applying the theory of translation to the fields radiated by each of the slots, Es1 and Es2.Thus
where, | (8) |
2.2.1. Calculation of the Input Impedance of the Antenna
The application of poynting theorem on the field radiated by each slot enables us to deduce the corresponding radiated power on a semi-sphere of radius r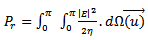 | (9) |
where
with 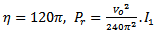

Hence,
It remains to calculate the subsceptance B associated with each of the slots. For this purpose we consider the rectangular microstrip resonator as an open circuit which can be represented by an equivalent capacitance C, or by a small length of line ΔL such that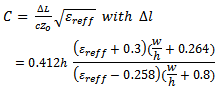 | (10) |
where c is the velocity of light, εreff the effective permittivity of the microstrip line of width w and characteristic impedance Zo,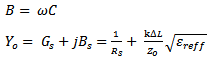 | (11) |
The input impedance and resonant frequency of the different feed configurations can be derived.
2.3. Input Impedance for the Microstrip Line Feed
From Figure 6,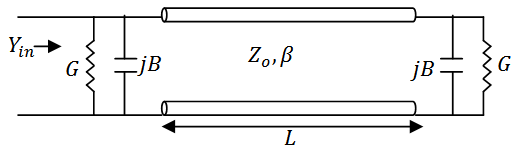 | Figure 6. Impedance model of microstrip line feed |
 | (12) |
where
At resonance the input impedance or admittance of the antenna is real, hence


2.4. Input Impedance for the Coaxial Cable Feed
From Figure 7,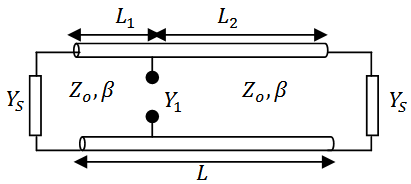 | Figure 7. Impedance model of Coaxiable cablefeed |
 | (13) |
The coaxial cable introduces a reactance, XL to the input impedance of the antenna, hence the effective impedance of the antenna becomes
h = thickness of the substrate penetrated by the central conductor of the coaxial cableεr = relative permittivity of the substrate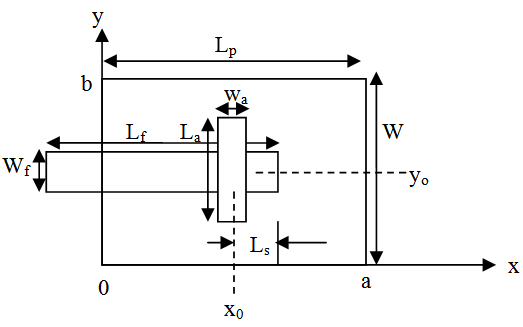 | Figure 8. Geometry layout of Aperture-coupled Patch Antenna |
The antenna system is separated into two regions as shown in Figure 9(a).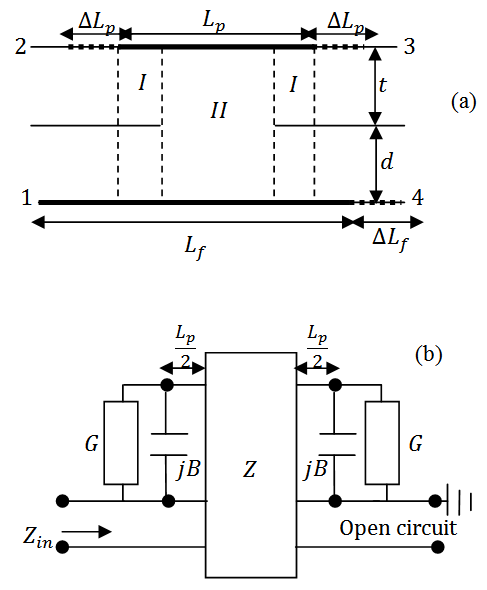 | Figure 9. Patch antenna system for analysis: (a) Side view, (b) Transmission line model of the patch antenna |
There are two symmetrical regions, represented as regions I, in which the microstrip line is separated from the antenna patch by the ground plane. This is the uncoupled region.Region II describes the medium of electromagnetic coupling between the feedline and the antenna patch. This region can be given a physical interpretation using an impedance model as in Figure 9(b) [4].Different circuit arrangements can be used to interpret this model. Figure 10 represents one possible arrangement.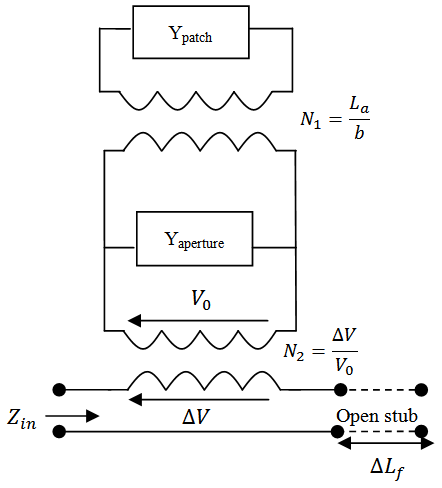 | Figure 10. Equivalent circuit of Aperture-couple patch antenna |
The resonant length of the rectangular patch antenna determines the resonant frequency and is λ/2 in its fundamental mode. In its fundamental mode, the length and width are calculated by the formulas [5] | (14) |
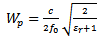 | (15) |
As shown in Figure 9(a), the fringing effects of the discontinuities at the open ends 2, 3 and 4, are represented by a hypothetical electrical extensions
and
. The effective lengths of the patch and feedline, respectively, become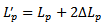 | (16) |
 | (17) |
where [2]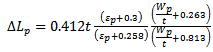 | (18) |
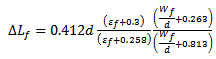 | (19) |
and
and
are the effective dielectric constants of the patch substrate and the feedline substrate, respectively.Since the patch radiates electromagnetic energy mainly through the two narrow slots along the two open ends of the patch,
is used to express the radiation conductances at these ends. This is shown in Figure 9(b). Using the modified Sobol’s formular [6], the conductances are calculated as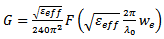 | (20) |
where | (21) |
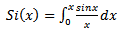 | (22) |
 | (23) |
and | (24) |

The effective width,
takes into account the fringing effects while
and
are the effective dielectric constant and the characteristic impedance respectively, of the patch.When
equation (7) may be simplified as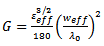 | (25) |
The value of the susceptance is calculated from [7] | (26) |
where
is given in equation (5), while
is the free space velocity.From Figure 11,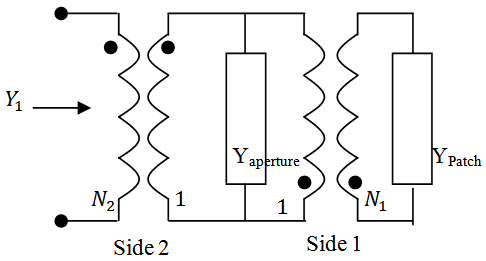 | Figure 11. Coupling between feedline and aperture (side 2), and coupling between aperture and radiating patch (side 1) |
 | (27) |
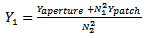 | (28) |
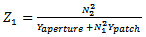 | (29) |
Taking into account the open-circuited stub in Figure 9(a), the total input impedance is | (30) |
where
is the characteristic impedance and
is the effective propagation constant on the microstrip feedline of open-circuited length
which accounts for
According to [8], the transformation ratio
is equal to the fraction of current flowing through the aperture over the total intensity: | (31) |
and  | (32) |
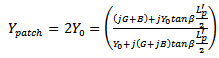 | (33) |
The knowledge of
enables the aperture admittance to be determined using equation (17).
3. Simulation Results and Evaluation
In practice, IEEE 802.11 WiMAX standards consist of 3.5-GHz (3.3–3.6 GHz) and 5.5-GHz (5.25–5.85 GHz) frequency bands. The resulting input impedance, and return are simulated at 5 GHz centre frequency using MATLAB. The results are shown in Figures 12 to 15 and the comparison of the performance characteristics of the different feed techniques are summarised in table 1.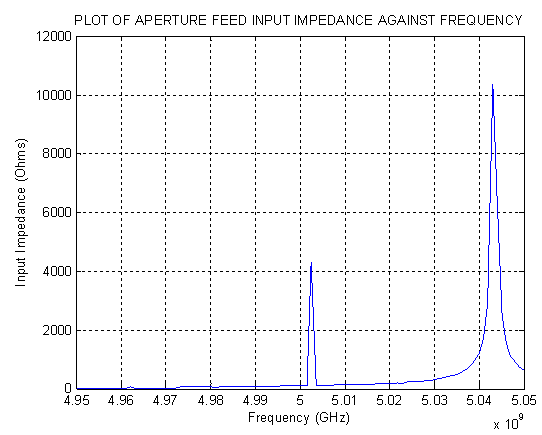 | Figure 12. Input impedance of Aperture-feed patch antenna at centre frequency of 5GHz |
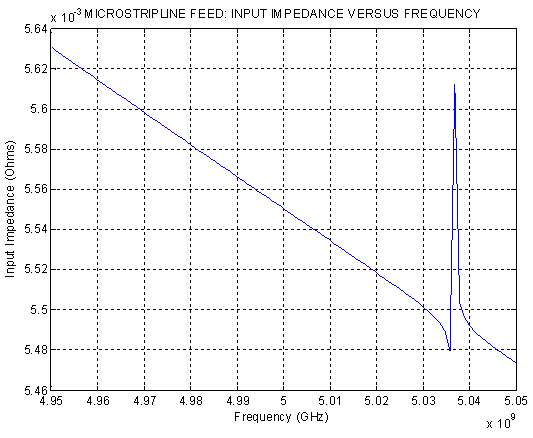 | Figure 13. Input Impedance response of Microstrip line feed |
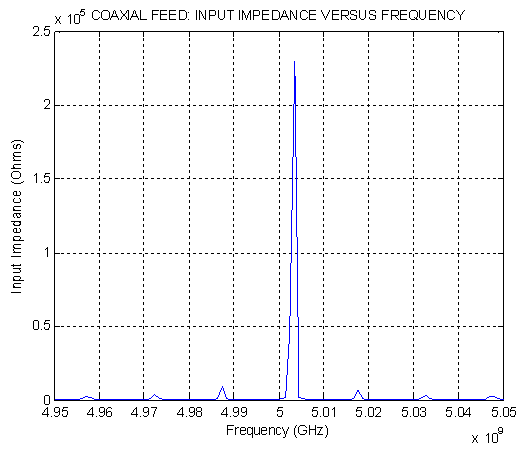 | Figure 14. Input Impedance response of Coaxial-feed |
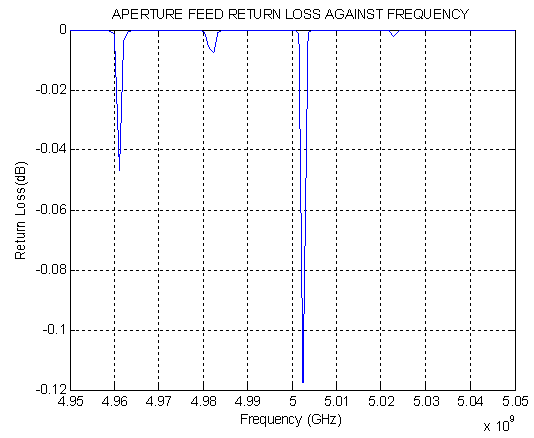 | Figure 15. Return Loss response of Aperture-feed at 5GHz Centre frequency |
Table 1. Characteristics comparison of different feeding techniques 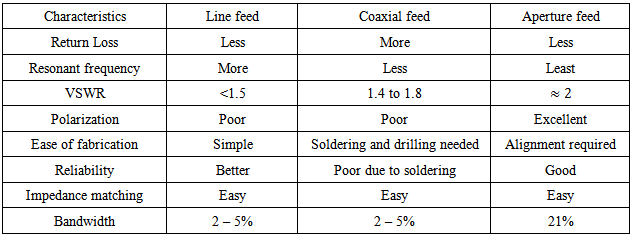 |
| |
|
Maximum bandwidth can be achieved by aperture coupling, at an input impedance of around 50Ω. Figure 16 & 17 shows simulated input impedance of around 50Ω between 4.95 – 5.0 GHz.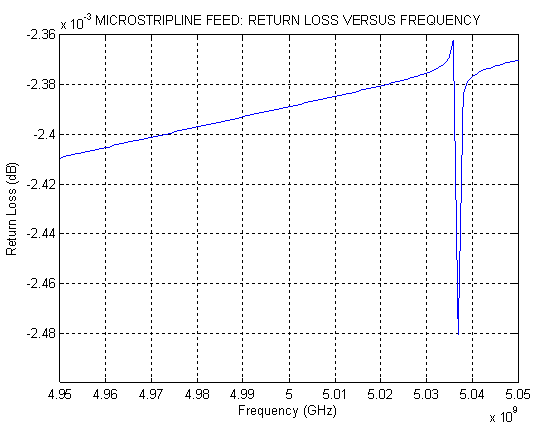 | Figure 16. Return Loss response of Lline feed technique |
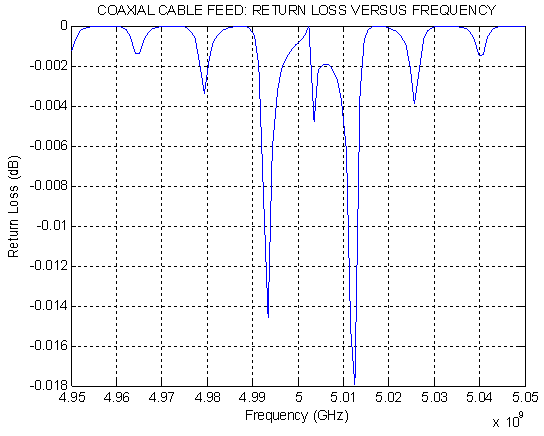 | Figure 17. Return Loss response of Coaxial-feed |
4. Conclusions
It can be seen from Table 1 tha selection of the feeding technique for a microstrip patch antenna is an important decision because it affects the bandwidth and other parameters. A microstrip patch antenna excited by different excitation techniques gives different bandwidth, different gain, different efficiency etc. Aperture coupled antennas are advantageous in arrays because they electrically isolate the feed and phase shifting circuitry from the patch antennas. The disadvantage is the required multilayer structure which increases fabrication complexity and cost.
References
[1] | T. Dunga et al; “Comparison of Circular and Rectangular Microstrip Patch Antennas”, IJCEA vol.2 Issue 4, pp. 187-197, July 2011. |
[2] | Q. Zhang, Y. Fukuoka, T. Itoh., “Analysis of a Suspended Patch Antenna excited by an Electromagnetically coupled Microstrip Feed” IEEE Transaction on Antennas and Propagation, Vol.33, n*8, August 1985, pp. 895-899. |
[3] | Robert W. Heath, Jr., Member, (2005), IEEE, and David J. Love Member, IEEE “Multimode Antenna Selection for Spatial Multiplexing Systems With Linear Receivers” IEEE transactions on signal processing, 53(8), pp 30423056. |
[4] | Adarsh B. Narasimhamurthy and Cihan Tepedelenlioglu, (2005), Member, IEEE,” Antenna Selection for MIMO OFDM Systems with Channel Estimation Error” IEEE transactions on vehicular technology, 58(5), pp 22692278. |
[5] | D. Orban and G.J.K. Moernaut “The Basics of Patch Antennas, Updated” September 29, 2009 edition of the RF Globalnet (www.rfglobalnet.com) newsletter. |
[6] | Charles Uzoanya Ndujiuba, Oluwadamilola Oshin, Nsikan Nkordeh “MIMO Deficiencies Due to Antenna Coupling”, International Journal of Networks and Communications 2015, 5(1): 10-17 DOI: 10.5923/j.ijnc.20150501.02. |
[7] | Gonca CAKIR, Levent SEVGI; “Design, Simulation and Tests of a Low-cost Microstrip Patch Antenna Arrays for the Wireless Communication”; Turk J ElecEngin, VOL.13, NO.1; 2005. |
[8] | Leo G. Maloratsky; “Reviewing the Basics of Microstrip Lines”; Microwave and RF; March 2000. |
[9] | Indrasen Singh, Dr. V.S. Tripathi; “Microstrip Patch Antenna and its Applications: a Survey”; Available online@www.ijcta.com; IJCTA; SEPT-OCT 2011. |
[10] | Marek Bugaj, Rafal Przesmycki, Leszek Nowosielski, and Kazimierz Piwowarczyk; “Analysis Di®erent Methods of Microstrip Antennas Feeding for Their Electrical Parameters”; PIERS Proceedings, Kuala Lumpur, MALAYSIA, March 27{30, 2012. |
[11] | Ahmed H. Reja; “Study of Microstrip Feed Line Patch Antenna”; Eng& Tech Journal, Vol 27, No.2; 2009. |
[12] | Rachmansyah, Antonius Irianto, and A. Benny Mutiara; “Designing and Manufacturing Microstrip Antenna for Wireless Communication at 2.4 GHz”; International Journal of Computer and Electrical Engineering, Vol. 3, No. 5, October 2011. |
[13] | John R. Ojha and Marc Peters; “Patch Antennas and Microstrip Lines”, Microwave and Millimeter Wave Technologies: Modern UWB antennas and equipment; www.intechopen.com; June 2012. |
[14] | Marwa Shakeeb; “ Circularly Polarized Microstrip Antenna”; The Department of Electrical and Computer Engineering Concordia University, Montreal, Quebec, Canada; Master’s Thesis, December 2010. |
[15] | Nagraj Kulkarni, S. N. Mulgi, S. K. Satnoor; “Design and Development of simple low cost Rectangular Microstrip Antenna for multiband operation”; International Journal of Electronics and Electrical Engineering; Volume 1, Issue 1 ISSN : 2277-7040; March 2012. |
[16] | Elena Pucci, Ashraf UzZaman, Eva Rajo-Iglesiasand Per-Simon Kildal; “New Low Loss Inverted Microstrip Line using Gap Waveguide Technology for Slot Antenna Applications”; Proceedings of the 5th European Conference on Antennas and Propagation, EUCAP 2011. Rome; 11-15 April 2011. |
[17] | Q. Zhang, Y. Fukuoka, T. Itoh., “Analysis of a Suspended Patch Antenna excited by an Electromagnetically coupled Microstrip Feed” IEEE Transaction on Antennas and Propagation, Vol.33, no.8, August 1985, pp. 895-899. |
[18] | D. Orban and G.J.K. Moernaut “The Basics of Patch Antennas, Updated” September 29, 2009 edition of the RF Globalnet (www.rfglobalnet.com) newsletter. |
[19] | K. Jagadeesh Babu, Dr. K. SriRama Krishna, Dr. L. Pratap Reddy; “A Modified E Shaped Patch Antenna For MIMO Systems”; International Journal on Computer Science and Engineering, 2(7), pp 24272430; 2010. |
[20] | Charles U. Ndujiuba, Adebiyi A. Adelakun, Oboyerulu E. Agboje; “Hybrid method of analysis for aperture-coupled patch antenna array for MIMO systems”. International Journal of Electromagnetics and Applications, 2015, 5(2): 90-97. DOI: 10.5923/j.ijea. 20150502.03. |