Pourimani R. 1, Noori M. 2, Madadi M. 1
1Department of Physics, Faculty of Science, Arak University, Arak, Iran
2Department of Biology, Faculty of Sciences, Arak University, Arak, Iran
Correspondence to: Noori M. , Department of Biology, Faculty of Sciences, Arak University, Arak, Iran.
Email: |  |
Copyright © 2015 Scientific & Academic Publishing. All Rights Reserved.
Abstract
Plants can transfer radioactivity into human nutrient cycle and ecosystem directly by vegetable food products and indirectly by animal food products. Also their role is important in absorption of environmental radioactive pollutants. Plant radioactivity is different based on soil and stone kind and geographical situation. Then subtle plant recognition of each environment can prepare valuable information for researchers. In this work the natural and artificial radioactivity concentrations of 226Ra, 232Th, 40K and 137Cs in 8 medicinal and edible plant species including: Saliva nemorsa L., Triticum aestivum L., Peganum harmala L., Vitis vinifera cv. Shirazi, Medicago sativa L., Gondelia tournefortii L., Descorainia sophia (L.) Webb et Berth and Achillea vermicularis Trin. were determined using HPGe gamma ray spectrometry system. Samples were collected from Bagh-e-Baraftab Village, Shazand in Markazi Province, Iran. Measured radioactivity concentrations for 137Cs, 40K, 232Th and 226Ra obtained from MDA to 1.02±0.35, from MDA to (2.75±0.01) ×103, from MDA to 7.79±1.40 and from 2.27±0.45 to 7.43±0.60 in Bq/kg respectively. Soil -to -plant transfer factor (TF) calculated for all samples. Results showed Peganum harmala had the maximum TF (3.17 for 40K) and Vitis vinifera cv. Shirazi was minimum (9.62×10-2 for 137C). Internal and external hazard indices were calculated for all samples that were less than unity. These results show that consumption of the studied plants would impose no health consequences to the consumers.
Keywords:
Natural radioactivity, HPGe, Medicinal plants, 137Cs, 232Th, 40K, 226R
Cite this paper: Pourimani R. , Noori M. , Madadi M. , Radioactivity Concentrations in Eight Medicinal and Edible Plant Species from Shazand, Iran, International Journal of Ecosystem, Vol. 5 No. 1, 2015, pp. 22-29. doi: 10.5923/j.ije.20150501.03.
1. Introduction
Natural and artificial radioactivity are the main radiation sources for human exposition. Uranium and Thorium series and Potassium are the main elements contributing to natural terrestrial radioactivity [1]. The average of 238U contents in the Earth’s crust has been estimated to be 2.7 mg kg-1 and its concentration may be as high as 120 mg kg-1 in phosphate rocks [2]. Firestone et al (1996) recorded average of 232Th content of the Earth’s crust about 9.6 mg/kg [3]. Environmental pollutions by radioactive isotopes come from nuclear weapon tests and accidents in nuclear power plants [4, 5]. Releasing radionuclides to the environment from nuclear facilities, during their normal operation or an unplanned situation, can potentially lead to radiation exposure of the nearby biota and human populations. Radioactivity that is released and dispersed into the atmosphere enters the terrestrial environment due to dry and wet deposition on soil and vegetation, causing exposure to either for human beings or biota [6]. So, the study of radionuclides in agricultural areas is of general concern because, once in the soil, most of them can recycle within the biota, similar to nutrients. Interactions between radionuclides and soil depend on the chemical form of element and some soil properties such as pH, mineralogical composition, organic matter content and nutrient status [7-9]. Plant uptake of radionuclides will be dependent on these interactions and will also depend on the metabolic and physiological characteristics of the plant species. Knowledge of the mobility of radionuclides in soils is needed to assess potential hazards from radionuclide inputs into the food chains [10]. Absorbed elements by plants through the root system (indirect contamination) or through the aerial organs (direct contamination) may be redistributed within plants [11, 8]. There are considerable differences in the uptake and translocation of long-lived radionuclides among different plant species [12]. They are transported to specific tissues based on the function of the element in plant metabolism and it gets reflected in its higher concentration in a particular part compared to others. Radionuclides can also be picked up along with nutrients and may have similar chemical behavior as the essential nutrient. In order to study the translocation and preferred site of accumulation of a radionuclide, the total content of a radionuclide in whole plant has been normalized for dry weight fraction of each plant part in some cases [13]. In this work radioactivity concentration determined using gamma ray spectrometry method included high resolution HPGe detector system in different medicinal and edible plant species.
2. Materials and Methods
2.1. Sampling and Sample Preparation
Eight medicinal and edible plant samples were collected from Bagh e Baraftab Village, Shazand in Markazi Provine, Iran. Shazand laid in latitude 33° N and longitude 49° E geographic coordinate system which has been shown in Figure 1. The collected medicinal and edible plants were Saliva nemorsa L., Triticum aestivum L., Peganum harmala L., Vitis vinifera cv. Shirazi, Medicago sativa L., Gondelia tournefortii L., Descorainia sophia (L.) Webb et Berth and Achillea vermicularis Trin. In this study using random integration and experimental sampling, a combined operation was undertaken to collect samples. Samples have been taken from various stages with average weight 5 kg fresh complete plants (root and aerial parts with the exception of Vitis vinifera that aerial part was used).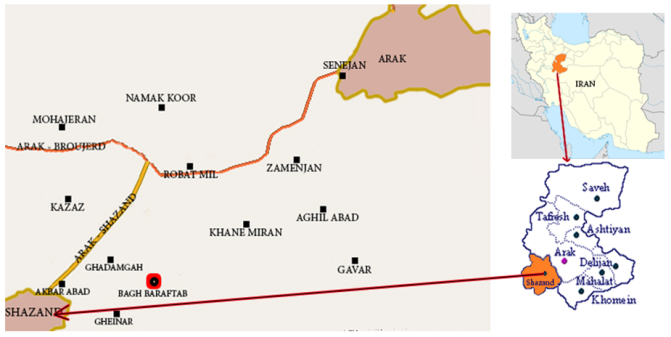 | Figure 1. Topography of sampling site, Shazand location in Markazi Province, Iran |
Root samples were put in distilled water for 15 minutes, and washed to remove the soil and other unwanted material. Then, they were chopped by plastic knife into small pieces and spread in place with special take care for preliminary drying. Dried plants were put in 80℃ oven temperature for two days. All the collected samples were crushed, homogenized and passed through 50-mesh screen changing into fine powder in laboratory. The prepared samples kept in standard sealed Marinelli Beakers container for minimum 50 days. This time is necessary for taking radioactive chain equilibrium where the decay rate of the daughters became equal to that of the parent. Net weight of each sample was 330 g. Soil samples were taken from the same area that collected plant samples at 5 cm soil depth, employing template method. The soils samples were dried at 200℃ oven for 12 hours. In order to obtain homogeneous samples, the dried soil samples were pulverized by a grinder into fine powder and passed through 10-mesh and 50-mesh screen. Also the same procedure used for packing and sealing in Marinelli Beakers container. Net weight of each sample was 950 g [14].
2.2. Plants Identification
All collected plants transferred to the plant systematics Laboratory in Arak University, Iran and were identified using available references [15, 16].
2.3. Radionuclide Activity Determination
Gamma ray spectrometry was done using an HPGe detector with a relative efficiency of 30% and full width at maximum (FWHM) of 1.95keV for 60Co gamma-energy line at 1332.520 keV. The detector is shielded in a chamber of two layers 10 cm thick lead and 2 mm thick by copper. This shield serves to reduce background radiation. The soft components of cosmic ray, consisting of photons and electrons, are reduced to a very low level by 100 mm of lead shielding. The X-ray (73.9 keV) emitted from lead by its interaction with external radiation is suppressed by the copper layer [17]. To minimize the effect of scattering radiation from the shield, the detector was located in the center of the chamber. Then, the sample was placed in a face-to-face geometry over the detector. The obtained spectra were registered by LRSMBSI software with multichannel analyzer (8192 channel). The spectra analysis were carried out using MEASTRO GAMMAVISION Ortec software. The 226Ra activity of the samples was determined through the intensity of 351.9 keV and 609.3 keV gamma ray lines of 214Pb and 214Bi, respectively. 232Th activity was obtained using gamma ray line of 228Acat 911.21 keV with intensity 26.6% and 968.97 keV and with an emission percentage of 17.4%. 40K and 137Cs were obtained using gamma ray line 1460.7 and 661.6 keV respectively. Background radiation measured in the same condition with empty Marinelli Beaker container and was reduced from all the spectra. Standard plant sample sources, included of
with specified activities, were used for calculation of the absolute efficiency. The detector efficiency calibration was obtained, based on the IAEA 154 instruction [18]. The counting geometry of samples, standard mixed source for efficiency calibration and background measurement was kept constant. The counting times of the all of the spectra were 86400 s. According to registered gamma ray spectrum, absolute efficiency of detector configuration was calculated using the following formula 1: | (1) |
Where Netarea is net counts under the full-energy peak corresponding to the Ei energy, Act is radionuclide activity at measured date, Pn (Ei) is the probability of Ei photon emission and t is the counting time. Equation fitted to experimental data by polynomial curve has been showed in formula 2. In this formula where Y is efficiency, a, b, c, d, e, f are constants and x is the gamma ray energy in keV. | (2) |
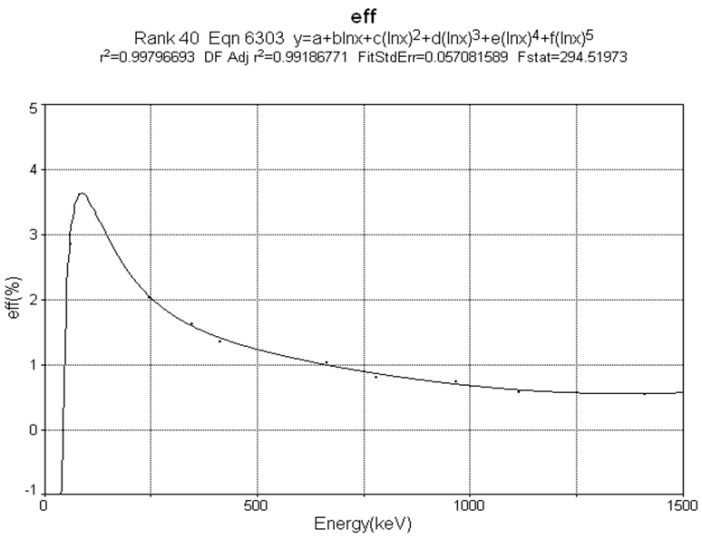 | Figure 2. HPGe detector efficiency variation versus gamma photon energy. The calibration curve has been shown as solid line. Squared points represent measured data |
The calibration curve fitted to experimental data by polynomial curve is showed in Figure 2 and the consequent nuclear activities were determined using the Formula 3 that expressed in Bq kg-1.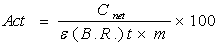 | (3) |
Where Cnet is the net count under peak, Act is activity concentration in (Bq/kg), ϵ is energy efficiency for gamma ray by detector, B.R. is the branching ratio of gamma ray intensity percentage, t is the time of spectra (sec) and finally m is the mass of sample (kg).
2.4. External Hazard Index (Hex)
The external hazard index is a relation that quantifies the exposure factor [19]. A widely used hazard index (reflecting the external exposure) called the external hazard index Hex. The external hazard index is an evaluation of the hazard of the natural gamma radiation. It is defined using formula 4 [20, 21]. Where ARa, ATh and AK are the activity concentrations of 226Ra, 232Th and 40K respectively. | (4) |
2.5. Internal Hazard Index (Hin)
In addition to external hazard index, radon and its short-lived products are also hazardous to the respiratory organs. The internal exposure to radon and its daughter products is quantified by their internal hazard index Hin, which is given by the Formula 5 [22, 23]. Where ARa, ATh and AK are the activity concentrations of 226Ra, 232Th and 40K respectively. The values of the indices (Hex, Hin) must be less than or equal to unity for the radiation hazard to be negligible. | (5) |
2.6. Radium Equivalent Activity (Raeq)
To represent the activity levels of 238U, 232Th and 40K which take into account the radiological hazards associated with them, a common radiological index has been introduced. This index is called radium equivalent activity and is mathematically defined as Formula 6 [20]. | (6) |
Where AU, ATh and AK are the specific activities of Uranium, Thorium, and Potassium respectively. This equation is based on the estimation that 10 Bq/kg of 238U equal 7 Bq/kg of 232Th and 130 Bq/kg of 40K produced equal gamma dose. The maximum value of Raeq must be less than 370 Bq/kg. Also the Raeq value of 370 Bq/kg is equivalent to the annual dose equivalent of 1.5 mSv/y, which that assumed to be the maximum permissible dose to human from their exposure to natural radiation from soil in one year.
2.7. Transfer Factor (TF)
The soil-to-plant trans factor (TF) indicated the transfer of radionuclides from soil to plant taken through the plant roots. From measured activity concentrations of the radionuclide in the plant and in the corresponding soil, the TF values were calculated according to the Formula 7 [24]. | (7) |
3. Results and Discussion
Plants identification results have shown that the plants except Achillea vermicularis and Gondelia tournefortii are from different plant families. Studied soils, plant species and their families are shown in Table 1. Calculated radionuclides activity concentrations (Bq kg-1) in 8 studied plant species have been shown in Table 2. Table 3 also shows calculated radionuclides activity concentrations (Bq kg-1) in studied soil samples, their nuclear hazards index and Raeq. Soil to plant transfer factor (TF) for all of plant species samples have been come in Table 4.Tabel 1. Studied soils, plant species and their families 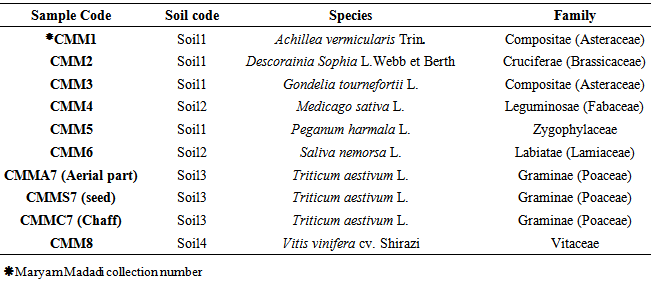 |
| |
|
Table 2. Calculated radionuclides activity concentrations (Bq kg-1) in 8 studied plant species 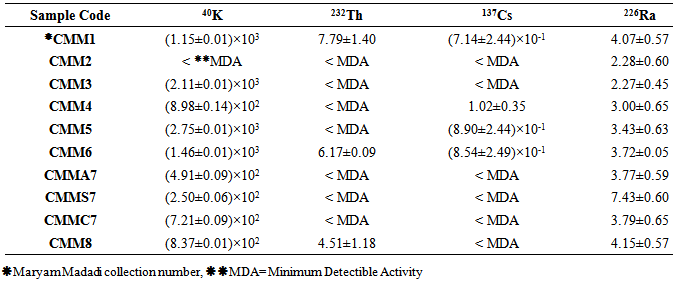 |
| |
|
Table 3. Calculated radionuclides activity concentrations (Bq kg-1) in studied soil samples, their nuclear hazards index and Raeq 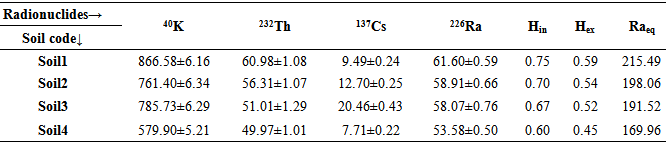 |
| |
|
Table 4. Soil to plant transfer factor (TF) 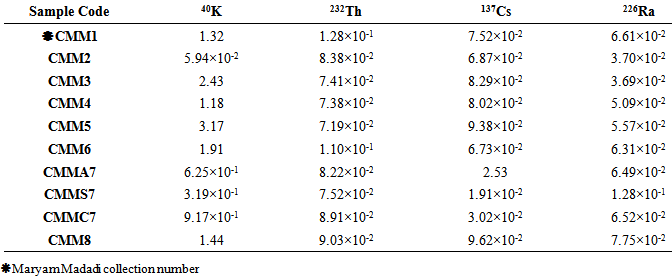 |
| |
|
3.1. 137Cs
137Cs activity concentrations have compared in Figure 3. It shows that 137Cs activity concentrations haven’t considerably changed in the different studied plants. It also was observed that Medicago sativa, Peganum harmala, Saliva nemorsa and Achillea vermicularis have maximum 137Cs activity concentrations of the radionuclides that have been given in Table 2.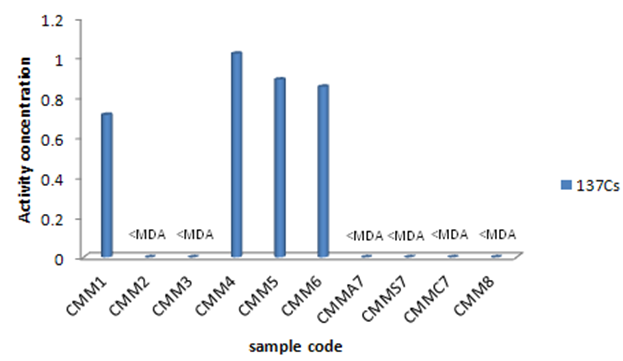 | Figure 3. 137Cs activity concentration of the studied plant species (Bq kg-1) |
3.2. 40K
40K activity concentrations have compared in Figure 4. 40K activity concentration is obviously more than other radionuclides with the exception of Descorainia Sophia. It also shows that Peganum harmala has the maximum 40K activity concentration in comparison to the other plants, so it could be a suitable choice for collecting 40K. Then Gondelia tournefortii, Saliva nemorsa and Achillea vermicularis showed the most 40K activity concentrations respectively and the least 40K activity concentrations observed in Descorainia Sophia, Triticum aestivum aerial part and seed respectively. This study showed that 40K activity concentration in T. aestivum chaff is more than its seed. Since 40K is a necessary material for plants growth. It is solved in water and carried through stems and leaves, so it’s clear that its amount in stems and leaves is more than the other parts as Pulhani et. al. reported (2005) for Medicago sativa [25, 26]. 40K activity concentration of Medicago sativa was calculated (8.98±0.14)×102 Bq kg-1 (Table 2) while Pulhani et. al (2005) reported from 41.34±1.24 to 292.72±10.00 which is less than the recent study.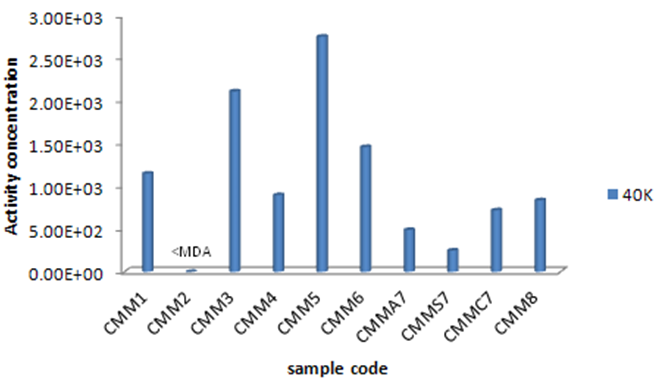 | Figure 4. 40K activity concentration of the studied plant species (Bq kg-1) |
3.3. 232Th
232Th activity concentration has been calculated as an average using two gamma energies from its daughter 228Ac (911.20 and 968.97 keV). 232Th activity concentrations varied from MDA to 7.79±1.40 as has been observed in three kinds of plants more than MDA values. They are compared in Figure 5. The most 232Th activity concentration has been determined for Achillea vermiculari, Saliva nemorsa and Vitis vinifera.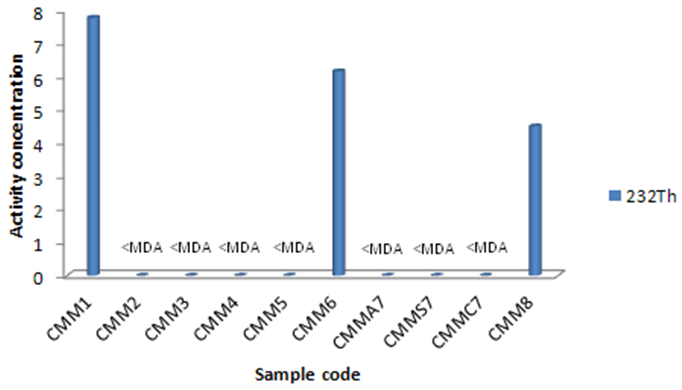 | Figure 5. 232Th activity concentration of the studied plant species (Bq kg-1) |
3.4. 226Ra
226Ra activity concentration has been calculated as an average using two gamma energies from its daughters 214Pb (295.22 and 351.93 keV) and 214Bi (609.31, 1120.28 and 1764.47 keV). 226Ra activity concentrations varied from 2.27±0.45 to 7.43±0.60. They are compared in Figure 6. The most 226Ra activity concentration has been determined for Triticum aestivum seed, Vitis vinifera and Achillea vermicularis.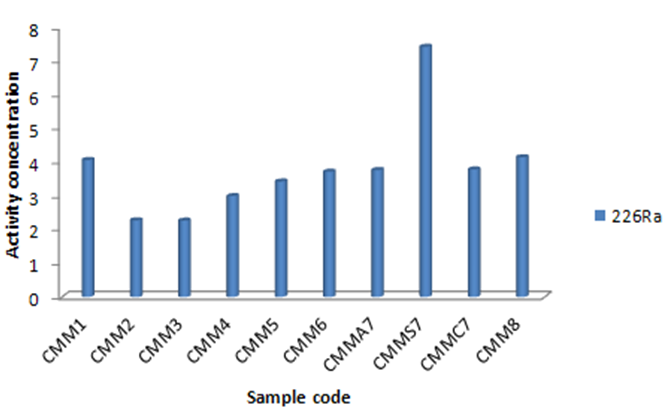 | Figure 6. 226Ra activity concentration of the plant samples in Bq kg-1 |
4. Conclusions
As measured activity concentration of the radionuclides in all of studied medicinal and edible plant species are close to or lower than the MDA (minimum detectable activity), consumption of the plants would impose no health cuences to consumers. Calculated internal and external hazard indices for all samples were less than unity. Also calculation of soil -to -plant transfer factor (TF) for all samples showed that Peganum harmala had the maximum TF (3.17 for 40K) and Vitis vinifera cv. Shirazi was minimum (9.62×10-2 for 137C). Measuring radionuclides activity concentration in agricultural crops is suggested in contaminated areas for human health.
ACKNOWLEDGEMENTS
The authors would like to thank of Arak University Research Council.
References
[1] | UNSCEAR. 1993. Sources and effects of ionizing radiation. United Nations Scientific Committee on the effect of atomic radiation, Report to General Assembly with Scientific Annexes. United Nation Publication sales No. E.94.IX.2. United Nations, New York. |
[2] | PADAM, S, RANA N, NAQAVI A and S RIVASTAVA D. 1996. Levels of uranium in water from some Indian cities determined by Fission Track Analysis. Radiation Measurements. 26: 683-687. |
[3] | FIRESTONE BR, SHIRLEY SV, BAGLIN MC, FRANK CHU SY & ZIPKIN J. 1996. The 8th Edition of Table of isotopes, CD-ROM, John Wiley & Sons Inc. |
[4] | KALAČ P. 2001. A review of edible mushroom radioactivity. Food Chemistry, 75 (1): 29-35. |
[5] | DUPRE de BOULOIS H, JONER EJ & LEYVA C. 2008. Role and influence mycorrhizal fungi on radiocesium accumulation by plant, Journal of Environmental Radioactivity, 99: 785-800. |
[6] | VARG B. 2008. Regulations for radioisotope content in food and feedstuffs, Food and Chemical Toxicology; 46: 3448-3457. |
[7] | SCHULLER P. et al., 2002. Global weapons’ fallout 137Cs in soils and transfer to vegetation in south-central Chile, J. Environ. Rad. 62: 181–193. |
[8] | SCHULLER P. et al. 1993. 137Cs concentration in soil, prairie plants, and milk from sites in Southern Chile, Health Phys. 64 (2): 157–161. |
[9] | SCHULLER P. et al. 1998. Long-term decrease of atmospheric test 137Cs concentration in the soilprairie plant-milk pathway in Southern Chile, Health Phys. 75 (1): 86–88. |
[10] | KÜHNE LA, NISSEN JP. 1973. Estudio mineralógico, petrográfico y físico-hídrico de los principales suelos de la provincia de Valdivia, Tesis Ing. Agr. Valdivia, Universidad Austral de Chile, Facultad de Ciencias Agrarias, 172 p. |
[11] | POSCHL M & NOLLET L. 2007. Radionuclide concentrations in food and the environment, Taylor & Francis Group, Printed in the United States of America. |
[12] | CHENA SB, ZHUA YG & HU QH. 2005. Soil to plant transfer of 238U, 226Ra and 232Th on a uranium mining-impacted soil from southeastern, China J. Environ. Radioactivity, 82: 223-236. |
[13] | UNSCEAR (United Nations Scientific Committee on the Effects of Atomic Radiation). 2000. Report of UNSCEAR to the General Assembly”, United Nations, New York, USA., 111–125. |
[14] | IAEA-TECDOC-360 (International Atomic Energy Agency). 2003. Collection and preparation of bottom sediment samples for analysis of radionuclides and trace elements, IAEA, Vienna. |
[15] | Ghahreman A. 1979-2006. Flore de l'Iran, A join project by the Research Institute of Forests and Rangelands (Iran) and Tehran University, Published by RIFR, Ministry of Reconstruction Jahad, Volumes 1-24. |
[16] | Rechinger KH, 1984. Flora Iranica .Akademische Druch-Und verlagsantalt Graz. |
[17] | AZIZ A. 1981. Methods of low-Level Counting and Spectrometry Symposium", Berlin, 221. |
[18] | IAEA-154 (International Atomic Energy Agency). 2000, Radionuclides in whey powder, Analytical Quality Control Services, Vienna, Austria. |
[19] | GIRIGISU S, IBEANU IGE, ADEYEMO DJ, ONOJA RA, BAPPAH IA & OKOH S. 2013. Scholars Research Library Journal, Archives of Applied Science Research, 5 (3): 204-210. |
[20] | BERETKA J & Mathew PJ. 1985. Natural radioactivity of Australian building materials, Industrial wastes and by-products, Health Phys, 48: 87-95. |
[21] | MUJAHID SA, RAHIM A, HUSSAIN S & FAROOQ M. 2008. Measurements of natural radioactivity and radon exhalation rates from different brands of cement used in Pakistan, Radiat. Prot. Dosim., 130: 206-212. |
[22] | XINWEI L. 2004. Natural radioactivity in some building materials of Shaanxi, China. J. Raioanal. Nucl. Chem., 262: 775-777. |
[23] | UNSCEAR, 2000. Sources effects and risks of ionizing radiation, Report to the General Assembly, With Annexes, United Nations Scientific Committee on the Effects of Atomic Radiation, New York, United Nations. |
[24] | IUR (International Union of Radioecologists). 1994. Handbook of parameter values for the prediction of radionuclide transfer in temperate environments, Technical reports series no. 364, Vienna: International Atomic Energy Agency (IAEA). |
[25] | PULHANI VA, DAFAUTI S, HEGDE AG, SHARMA RM & MISHRA UC. 2005. Alpha, Beta and Gamma Radioactivity, Burlington House, Journal of Royal Society of Chemistry, Piccadilly, London, W1V 0BN, Registered Charity Number 207890. |
[26] | IAEA (International Atomic Energy Agency). 1989. Measurements of radionuclides in food and the environment, A guide book technical report series, No. 295, pp 27–28. Vienna, Austeria. |