Drissa Boro, P. Florent Kieno, Emmanuel Ouedraogo
Laboratoire d’Energies Thermiques et Renouvelables (L.E.T.RE), Université Ouaga 1 Pr Joseph KI-ZERBO, Unité de Formation et de Recherche en Sciences Exactes et Appliquées (UFR/SEA), Burkina Faso
Correspondence to: P. Florent Kieno, Laboratoire d’Energies Thermiques et Renouvelables (L.E.T.RE), Université Ouaga 1 Pr Joseph KI-ZERBO, Unité de Formation et de Recherche en Sciences Exactes et Appliquées (UFR/SEA), Burkina Faso.
Email: |  |
Copyright © 2017 Scientific & Academic Publishing. All Rights Reserved.
This work is licensed under the Creative Commons Attribution International License (CC BY).
http://creativecommons.org/licenses/by/4.0/

Abstract
This paper presents an experimental study on the thermal and mechanical characterization of local materials used in building. These materials are blocks of compressed earth and stabilized with sawdust. Measurements of the thermophysical properties reveal that thermal conductivity decreases as the volume of sawdust in the mixture increases. It is 0.962 W.m-1.K-1 for simple compressed earth blocks, 0.907 W.m-1.K-1 for 1% sawdust and 0.512 W.m-1.K-1 for a dosage at 8%. These materials diffuse less heat and rapidly warm up significantly on the surface if the rate of sawdust increases. Dry compressive strength tests show an improvement of the mechanic strength when the dosage rate ranges between 0% and 1%. Lastly, we have also calculated the depth of diffusion of a room according to the various types of blocks used to build it.
Keywords:
Thermophysical properties, Mechanical strength, Compressed earth blocks, Sawdust, Stabilization
Cite this paper: Drissa Boro, P. Florent Kieno, Emmanuel Ouedraogo, Experimental Study of the Thermal and Mechanical Properties of Compressed Earth Blocks Stabilized with Sawdust According to the Rates for the Thermal Insulation of a Building, International Journal of Construction Engineering and Management , Vol. 6 No. 3, 2017, pp. 103-109. doi: 10.5923/j.ijcem.20170603.05.
1. Introduction
Housing issue in developing countries like Burkina Faso will not be handled with modern building materials. Production capacities are limited and, above all, their costs are high [1]. As a result, research is being conducted on local materials to improve their thermal behavior, strength and reliability to be used in buildings. However, it is obvious that the earthen-made habitat suffers from a lack of mechanical strength. To overcome these weaknesses, the physical stabilization by adding sawdust and the mechanical one with mechanical compression remain the appropriate solutions. P. Meukan et al. [2] in a thermal and mechanical study of earth stabilized with sawdust have shown that laterite blocks to which sawdust is added are better thermal insulators than simple laterite blocks.In this paper, we expound the study of the thermophysical and mechanical properties of the compressed earth blocks stabilized with sawdust at different dosage rates. The work by Imbga et al [3] presented the thermophysical parameters of various formulations with laterite and slaked lime. This investigation has shown that the thermal conductivity decreases when the rate of lime is lower than 4% and increases when the rate is higher than 4%. This study indicates also that the compressive strength increases but very slowly. J. Torkaman et al [4], in a parametric experimental study on the effects of partial replacement of Portland cement by wood fiber, rice husk ash and limestone powder waste for producing a lightweight concrete block as building materials, have founded that the optimum replacement level of these materials was 25% by weight; this replacement percentage resulted in good physic-mechanical properties. Amir Rahimi et al [5] studied the effects of using different percentages of organic waste ash instead of cement by weight on the mechanical properties and ductility of concrete specimens. Their results showed that 5% of these organic waste ashes instead of cement by weight lead to optimum performance. Influence of banana fibers on the mechanical properties of banana compressed block have been studied by Marwan M. et al [6]. They concluded that the blocks constructed by adding banana fibers throughout the mix performed better than the block with no fibers. We particularly study the impact of wood sawdust content on the thermal conductivity and the compressive strength of the stabilized materials and we will determine the optimum wood residue content for the best thermal and mechanical performances.The compressed earth block is raw clay molded into metal molds (iron, steel) or any other material offering the same qualities. It is a thermal mass which is a stock of heat or freshness, due to its high density and its quality as a hygrometric regulator [7].
2. Methods and Techniques used in Materials Production
The experimental device used to make test tubes includes a manual press, a mold with its accessories. The BTC production process is subdivided into five (5) steps (choice of soil, riddling, mixing, production, drying). The production of BTC is done using the relevant manual presses for the various types of blocks produced. Concerning the production site, presses are designed to produce two or more bricks at a time. The press used to produce blocks is provided with a steel mold to reduce deformations due to the compression load. This mold includes two (02) blocks of the following dimensions: Length L = 14cm, Width l = 14cm Height H = 8cm. Bricks marked L0 are obtained after preparing the mixture without any stabilizer. The bricks marked L1 are made of lateritic earth plus 1% of the mass of sawdust used to prepare the mixture. The bricks market L2 are those made of lateritic earth plus 2% of the mass of sawdust used to prepare the mixture. This process was repeated by varying the rate of sawdust to 8% of the total mixture. Beyond this content of sawdust, the material no longer has the least mechanical strength.
3. Study of the Dry Compressive Strengths of Materials According to the Dosage Rate of the Sawdust
Tests to determine the compressive strength of dry materials have been developed. These tests consist in applying to a dry block sample (dry BTC), using a crushing press, a simple compressive load until the complete breaking and determining the load value (read on the screen of the crushing press). The dry compressive strength of the bricks is given by the following formula:
With:• RC(MPa) compressive strength;• F(N) the maximal load born by the brick;• S(mm²) the strength pressure area.
3.1. Measurements of the Compressive Strengths of Earth Blocks Stabilized with Sawdust
Table 1 below gives the results of the compressive strength measurements.Table 1. Compressive Strength Values 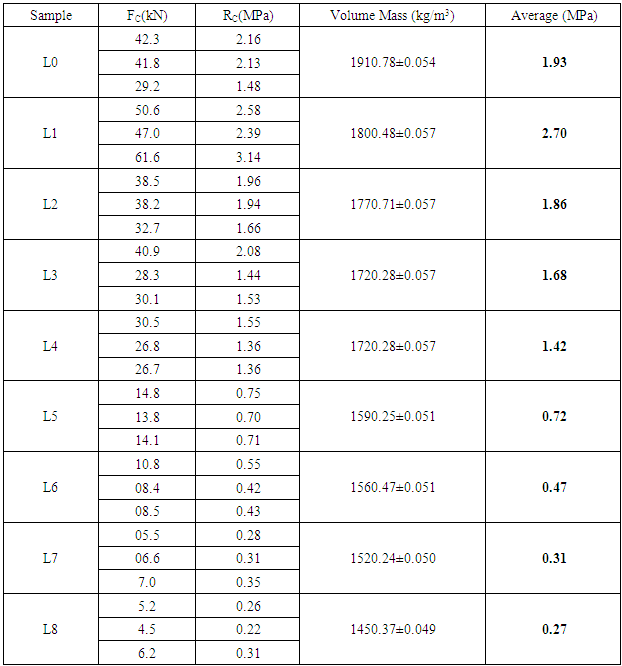 |
| |
|
The various values of the compressive strengths presented in the table above enables us to draw the variation curve of the compressive strength according to the level of stabilizer in materials (Figure 1).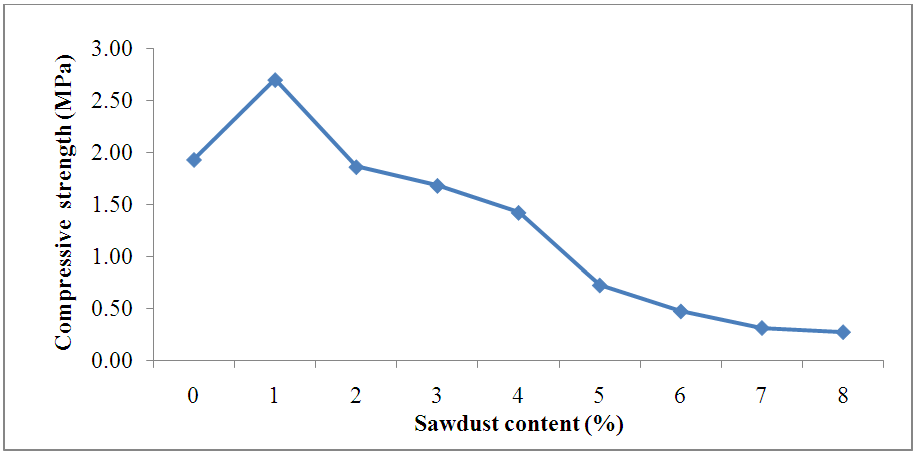 | Figure 1. Variation of the compressive strength according to the sawdust content |
3.2. Analysis and Interpretation of Results
We find that the average value of the compressive strength of compressed laterite bricks without stabilizer is 1.93 MPa. This strength increases to reach the maximum average value of 2.70 MPa if we add 1% of sawdust, accounting for an increase of 0.77 MPa or 28.51%.The dry compressive strength is due to the molecular cohesion; the greater the cohesion, the higher the compressive strength. For a sawdust content of more than 1%, the strength value decreases due to poor adhesion of the laterite with the sawdust. Indeed, test tubes become thicker and thicker (larger dimensions than simple earth test tubes) just after the release from the mold when the sawdust content increases.The volume increase could be explained by the fact that water absorbed by wood alters these physical properties, including its elasticity modulus (mechanical stress that would lead to an extension of 100% of the initial length of the material). The increase in the volume of the test tube after the release from the mold may be explained by the fact that the elastic limit (value of the maximum stress which generates irreversible deformation) is not reached during compaction. The sawdust therefore tends to resume its initial dimensions as soon as the compressive strength stops. Thus, the reduction in density is accompanied by losses in mechanical compressive strength due to the decrease in the material compactness. For 1% stabilization, the compressive strength increases by 0.77 MPa, i.e. 28.51%.
4. Experimental Measurements of the Thermal Properties of Laterite Bricks
4.1. Principle to Measure Thermal Properties
Thermophysical properties of materials are quantities that characterize the behavior of materials. They constitute the capacity of heat to spread in a body or to be stored in it. Thermophysical characteristics such as thermal conductivity, thermal diffusivity and volumetric specific heat were measured using KD2-pro, a dual-needle probe developed by Decagon [8]. This device uses the model proposed by CRASLAW and JAEGER [9] to solve the equation of heat transfer using the linear heat source propagation method in a transitory regimen in semi-infinite medium, an equation published in the IEEI [10]. The advantages of this type of method lie mainly in the simplicity of the equipment, the speed of measurements and the possibility to do it in situ under any hygrothermal conditions.
4.2. Results of the Measurements of Thermophysical Properties
The results of the measurements of the thermal properties are registered in the table 2 below.Table 2. Thermal Property Values of BTC 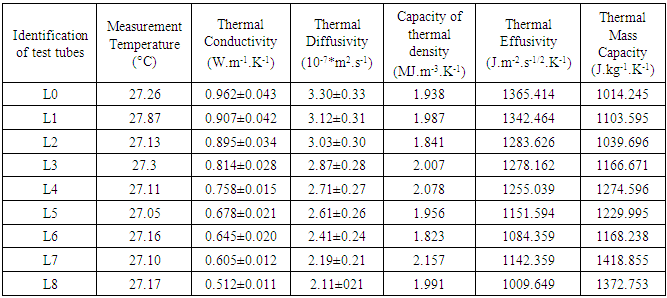 |
| |
|
4.3. Analysis and Interpretation of Experimental Results
a) Thermal ConductivityThermal conductivity values per rates of sawdust are represented in figure 2.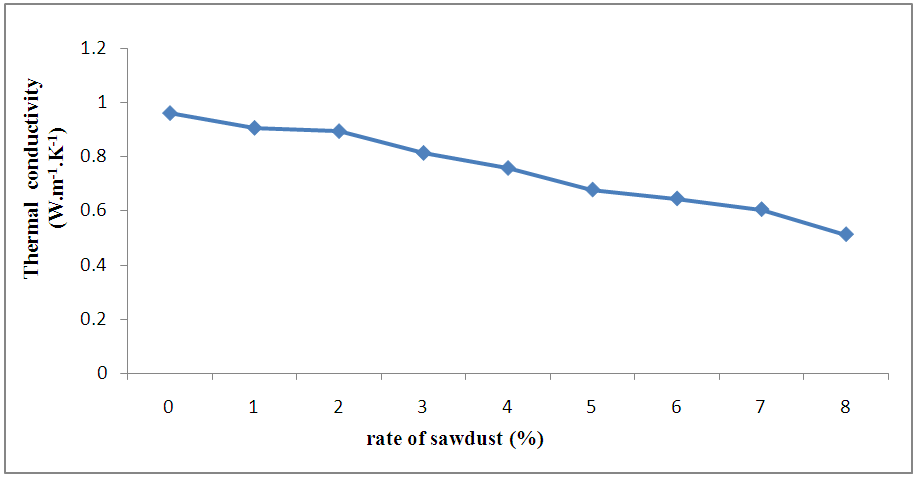 | Figure 2. Variation of the thermal conductivity with the rate of sawdust |
We see that the conductivity changes against the sawdust rate. The conductivity of the mixture decreases when the rate of sawdust added increases. Thus, by increasing the proportion of sawdust in the earth, the material becomes more thermally insulating.The thermal conductivity value of the lateritic earth without stabilizer has an average value of 0.962 W.m-1.K-1. This value decreases when the sawdust content of the wood in the block increases. This is 0.907Wm-1.K-1, when 1% of the mass of sawdust is added and 0.512Wm-1.K-1 if 8% of the mass of sawdust is added, i.e. 46.78% decrease of conductivity. Indeed, sawdust makes it possible to create pores within the material and the higher its content, the more porous the material. The material obtained after mixing is more porous than the initial material and contains more air besides the sawdust. Since air and sawdust are insulators (with very low thermal conductivities), they contribute to the insulation of the block. Increasing the rate of stabilization reduces heat propagation in the material due to the increased vacuum induced by sawdust.b) Thermal diffusivityFigure 3 gives the values of the thermal diffusivity.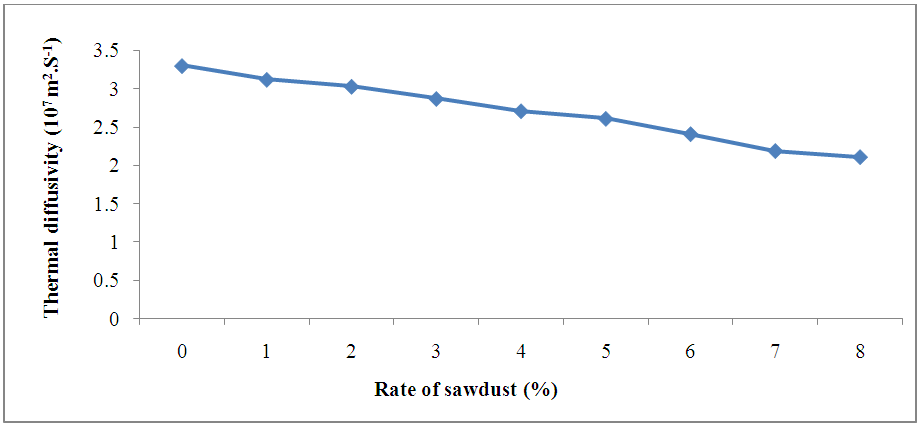 | Figure 3. Thermal diffusivity values with the rate of sawdust |
The variation curve of the thermal diffusivity shows that this decreases when the wood residue content increases. The more insulating the material is, the lower the diffusivity.This decrease in diffusivity could be explained by the presence of sawdust, which is a material with low diffusivity (a = 1.38 * 10-7 m2.s-1) [11] compared to the non-stabilized laterite we have formulated (3.3*10-7 m2.s-1). Moreover, Meukan's work [12] on compressed earth blocks revealed that the thermal diffusivity of the unstabilized single laterite had an average value of 4.6 *10-7 m2.s-1. Therefore, the higher the sawdust content, less the block will diffuse heat. c) Thermal EffusivityFigure 4 gives the thermal effusivity values according to the sawdust content.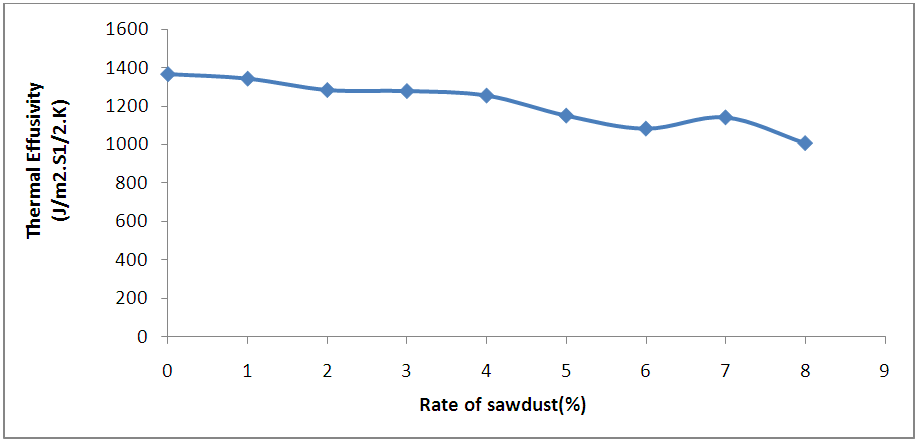 | Figure 4. Variation of the thermal effusivity with the fraction of sawdust |
The calculation of the effusivities led to nearly the same results as that of the materials with precise effusivity. [8] The value of the thermal effusivity of the simple lateritic soil without stabilizer has an average value of 1365,414 J.m-2. s-1/2.K-1. This value decreases as the sawdust is added. The effusivity of laterite bricks is thus a decreasing function of the wood residue content. The lower the thermal effusivity, less heat will be absorbed by the material in order to heat up significantly at surface level because of the slow diffusion of this heat due to the incorporation of low thermal effusivity sawdust (E = 360- 600 Jm-2.s-1/2.K-1) depending on the type of wood used [13].d) Specific heatFigure 5 provides the values of the specific heat.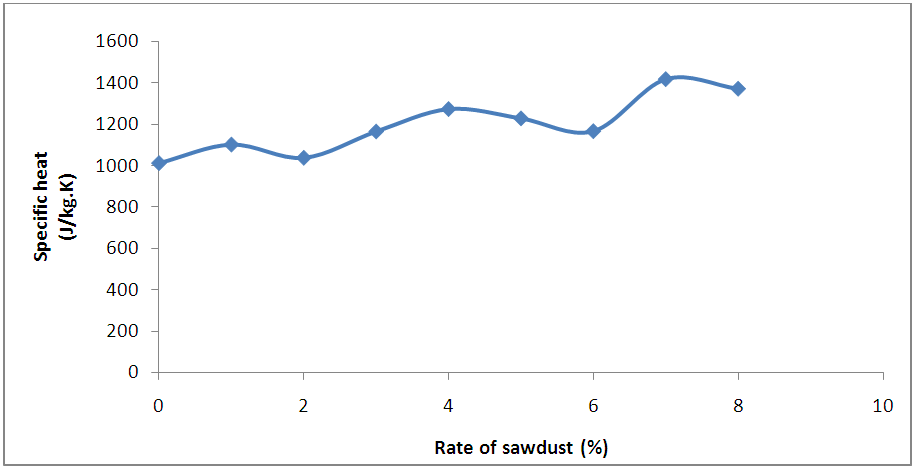 | Figure 5. Values of the specific heat according to the rate of sawdust |
On the whole, the curve of variation of the specific heat capacity shows that this is an increasing function of the wood residue content. This is probably due to the high calorific capacity of the wood (Cpbois = 1660 to 1710 J.kg-1.K-1 for a density ranging between 700 and 800 kg / m3) compared to earth. This high thermal capacity will ensure good heat storage.
5. Depth of Heat Diffusion
Heat diffusion in the material depends on its diffusivity (𝜶) which is the ratio of its thermal conductivity λ (W.m-1.K-1) by the volume specific heat. Thermal diffusivity α (m2.s-1) determines the rate at which heat spreads over in the material. More the material has high conductivity and small calorific capacity, more the diffusivity is high. The relationship between the thickness requested and the heat diffusion time within a homogeneous component of the building is established by calculating the product of the surface calorific capacity Qs (J.mol-1.K-1.m-2) by the thermal strength of this component R (m2.KW-1).The diffusion time t (s) is given by the equation (1) below.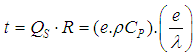 | (1) |
Over a given period t, the heat will penetrate more into a material with a greater diffusivity. The diffusion depth e (m) of heat in a material is given by the following formula (2). 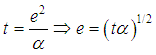 | (2) |
The values of the diffusion depths of the various materials enable us to draw the figure 6.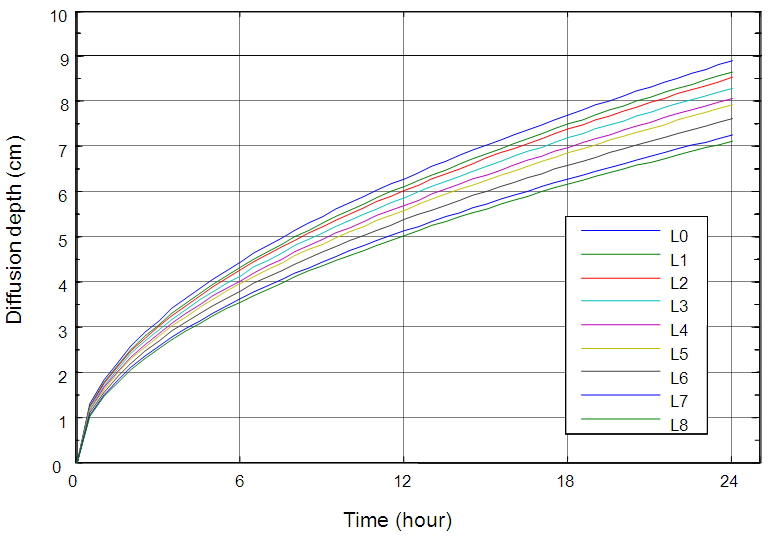 | Figure 6. Diffusion depth of BTC formulated |
Let’s take the length of sunshine on a daily cycle of 12 hours, the characteristic thickness reached is 7.70 cm for the bricks in case of single earth (L0), 7.49 cm for the bricks stabilized at 1% of sawdust (L1), these values decrease to 6.16 cm for a stabilizer level of 8%. With these results, we see that the depth of diffusion is less than the thickness of the wall whatever the material used; thus, these materials have good thermal properties.
6. Conclusions
At the end of our study, we were able to determine the thermophysical properties (thermal conductivity, diffusivity, effusivity, volumetric heat) and the compressive strength of the compressed earth bricks stabilized with sawdust.For a sawdust ratio ranging between 0% and 8%, the value of the thermal conductivity decreases from 0.962 to 0.512 W.m-1.K-1, i.e. a decrease of 46.77%.On the whole, the thermal conductivity decreases when the sawdust content in the making up of the mixture increases, which shows that these materials have good thermal insulation. We also find that the compressive strength of the compressed laterite brick without stabilizer has an average value of 1.93 MPa. This strength increases to reach the maximum average value of 2.70 MPa if we add 1% of sawdust and then decreases as from 2%. The mechanical strength (strength higher than 1 MPa) of the blocks is acceptable for a maximum stabilization rate of 4%. If we take the thermal and mechanical properties of the bricks into account, the best rate of sawdust to recommend is 1%. Concerning thermal performances, we see that the depth of diffusion is less than the thickness of the wall whatsoever the material used.
References
[1] | NOUDA A., Matériaux de construction traditionnels: Un bilan des recherches et des expériences, L’Economiste, 25-06-1992. |
[2] | Meukam P., Jannot Y., Noumove A. ,Kofane T. C., Thermo -physical characteristics of economical building materials, Construction and Building Materials, vol18, (2004). |
[3] | Imbga K., Sambou V., Kieno P. F., Dieye Y., Thermal and mechanical study of laterite stabilized with lime for sustainable building applications in Sahel countries, International Journal of Current Research, Vol. 9, Issue, 01, pp. 44609-44615, 2017. |
[4] | Torkaman J., Ashori A., Momtazi A. S., Using wood fiber waste, rice husk ash, and limestone powder waste as cement replacement materials for lightweight concrete blocks, Construction and Building Materials, 15 January 2014. |
[5] | Rahimi A., Dogmechi F., Daemi M. R., Experimental study on utilization of organic waste ash in concrete mixtures, KSCE Journal of Civil Engineering, pp. 1-7, 2017. |
[6] | Marwan M., Nasim U., Experimental analysis of Compressed Earth Block (CEB) with banana fibers resisting flexural and compression forces, Case Studies in Construction Materials 5, pp.53-63, 2016. |
[7] | KHELIFI H., Formulation de matériaux argileux stabilisés pour la fabrication de blocs constructifs, XXXe Rencontres AUGC-IBPSA Chambéry, Savoie, 6 au 8 juin 2012. |
[8] | DECAGON, KD2 Pro Specifications, Decagon Inc., http://www.thermal.decagon.com, 2006. |
[9] | CRASLAW J., JAEGER H., Conduction of Heat in Solids, Oxford, 2nd Edition, Londres, 1959. |
[10] | IEEI 442 «Guide for Soil Thermal Resistivity Measurement in: Proceedings of the IEEE Standards Committee» 1981. |
[11] | Thanh Hung Pham et al, Etude expérimentale et numérique de la conductivité thermique d’un composite chaux- chanvre. XXXe rencontres AUGC- IBPSA. Chambéry- Savoie, 6 au 8 juin 2012. |
[12] | Al Rim K., Ledhem A., Douzane O., Dheilly RM., Queneudec M., Influence of the proportion of wood on thermal and mechanical performances of clay-cement-wood Composites, Cement and Concrete Composites, vol. 21, 1999, p. 269-276. |
[13] | Mémento technique de bâtiment (ministère de l’équipement des transports, du logement, du tourisme et de la mer de la république française) www.certu.fr. |